Effects of {2-[(3-carboxy-1-oxoprogy1)amino]-2-deoxy-D-glucose} on human hepatocellular carcinoma cell line1
Introduction
Apoptosis is a cellular suicidal process that plays an important role in the elimination of unwanted or damaged cells. Under the physiological condition, apoptosis counteracts with cellular proliferation to maintain homeostasis. Dysregulated apoptotic response has been associated with certain diseases such as neural degenerative disorders and cancers. Many chemotherapeutic agents, radiation therapy, and therapeutic cytokines are known to induce apoptosis. Thus, apoptosis has been recognized as one of the major modes of cell death in cancer therapy. Resistance to undergo apoptosis is one of the important mechanisms that leads to treatment failure in cancer[1–2].
In recent years, agents or treatment modalities that result in apoptosis have become a new focus in cancer therapy[3–5]. {2-[(3-carboxy-1-oxoprogy1)amino]-2-deoxy-D-glucose} (COPADG)[6] is a derivative of D-glucose (molecular structure shown in Figure 1). D-amino-glucose is a monose that comes from the chitosan’s degradation. Previous studies by others have discovered that some D-amine-glucose derivatives were able to induce leukemia cells K562 to differentiate into macrophages[7]. However, it is still unknown whether derivatives of D-amine-glucose can induce apoptosis in tumor cells. In this study, we aimed to determine whether COPADG could induce apoptosis on human hepatocellular carcinoma cells (HepG2).
Materials and methods
Materials Human hepatocellular carcinoma cells (HepG2) were provided by the Department of Pathology, Fourth Military Medical University, China. COPADG was newly synthesized by the Lanzhou Institute of Chemical Physics, Academy of Sciences, China, and was dissolved in distilled water, filter-sterilized with 0.22 µm filter disc, and stored at 4 ºC until use. The structure of COPADG is shown in Figure 1. RPMI-1640 medium was purchased from Invitrogn (Invitrogen Corporation, CA, USA). MTT, agarose, and all routine chemicals were purchased from Sigma-Aldrich (Sigma-Aldrich Chemicals, Louis Mo, USA). Trypsin, acridine orange, and fetal calf serum were purchased from Shanghai Biological Engineering Limited (Shanghai, China).
Cell culture HepG2 cells were cultured in RPMI-1640 medium supplemented with heat inactivated 10% fetal calf serum (FCS), streptomycin 100 mg/mL and benzylpenicillin 100 mg/mL . Cells were maintained in a humidified atmosphere of 5% CO2 with 95% air at 37 °C. Medium is changed every 48 h.
MTT colorimetric assay The MTT test is based on the enzymatic reduction of the tetrazolium salt MTT in viable/metabolically active cells. Cells at approximately 85%–90% confluency were harvested with 0.25% Trypsin/0.02% edetic acid solution and seeded into a 96-well plate at a density of 4×103 cells/well. Cells were incubated with various concentrations (1–30 µmol/L) of COPADG for indicated time (24–96 h). Control cells were treated in the same way except that COPADG was replaced by sterile PBS. After treatment, medium was changed to fresh medium, and cells were incubated with 5 g/L of MTT for 4 h. MTT was then dissolved with 150 µL of 10% Me2SO for 1 h. The optical densities (OD) in the 96-well plates were determined using a microplate reader at 490 nm. Cell growth inhibition was estimated by using the following formula:
% Growth inhibition=1–OD (treated cells)/OD (control cells)×100%
Morphological study of apoptosis by acridine orange Acridine orange (AO) is a membrane-permeable fluorescent dye. It is specific for apoptotic cell death and does not significantly stain necrotic cells[8]. Apoptotic nuclei exhibiting typical changes such as nuclear condensation and segmentation will be stained yellow by AO. Cells (2×105/well) were seeded into 6-well plate, grown to approximately 85% confluency, treated with various concentrations of COPADG for various durations, fixed in methanol: glacial acetic acid (3:1) for 30 min at room temperature, washed in PBS, and stained with 0.01% AO. Stained cells were washed with distilled water, viewed under a fluorescence microscope. Apoptotic cells were counted and expressed as a percentage of the total number of cells counted.
Transmission electron microscopies (TEM) Cells were processed for TEM to further evaluate apoptosis. Cells of logarithmic growth were treated as described above, harvested by trysinization, fixed in 3% glutaraldehyde for 1 h. After removal of the primary fixative, cells were washed three times in MOPS buffer, post fixed in 1% osmium tetroxide (OsO4), dehydrated in graded alcohol, and embedded in epoxy resin. Ultra thin sections were double-stained with lead citrate/uranyl acetate before being examined using JEF-100CX transmission electron microscope (Japan).
Agarose gel electrophoresis for detection of DNA fragmentation Upon completion of treatment, cells were processed for DNA fragmentation analysis by agarose gel electrophoresis as described previously[9]. Briefly, cells (detached and attached) were incubated in 400 µL of lysis buffer (Trinton X-100, 10 mmol/L Tris, 1 mmol/L edetic acid, pH 8.0) for 30 min. The whole cell lysates were centrifugated at 12 000 rpm at 4 °C for 15 min . The supernatants were then incubated with 1 g/L proteinase K at 56 °C for 3 h followed by incubation with 2 g/L RNase A for 2 h. The solution was then sequentially extracted with an equal volume of phenol (pH 8.0), phenol/chloroform (1:1), and chloroform/isoamyl alcohol (24:1). Total genomic DNA was precipitated by adding 1/10 volume of 3 mol/L sodium acetate (pH 5.2) and 2 volumes of ice-cold ethanol for overnight at -20 °C. DNA was collected by centrifugation at 13 000 rpm at 4 °C for 10 min. The pellets were washed once with 70% alcohol and air-dried before being dissolved in TE buffer (10 mmol/L Tris-HCl, pH 8.0, 1 mmol/L edetic acid). DNA concentration was measured at OD 260 nm, and 5 µg was loaded onto a 1.5% agarose gel in TAE buffer. Electrophoresis was performed at 35 V for 4 h, the gel was stained with ethidium bromide, viewed under UV illumination, and photographed.
Flow cytometry analysis Following treatment, cells were rinsed with PBS, trypsinized by 0.25% trypsin/0.02% EDTA solution, and collected by centrifugation at 1000 rpm at 4 ºC for 5 min . The cell pellets were fixed in 70% ethanol at 4 °C for at least 1 h. The fixed cells were washed twice with PBS, resuspended in PBS containing 50 g/L RNase A and 50 mg/L of propidium iodide (PI). The suspension was incubated at 37°C for 30 min, filtered through 200 µm nylon mesh, and were analyzed by flow cytometer (Coulter EPICS XL). The apoptotic population was identified as cells appeared in sub-G0/G1 peak. Multicycle software was used for data analysis.
Statistical analysis The results are presented as mean±SD. Each experiment was repeated at least three times. Statistical differences between each group were determined by single factor analysis of variance and correlation analysis.
Results
Proliferation inhibition of HepG2 cells by COPADG As indicated by MTT assay results, proliferation of HepG2 cells was significantly inhibited by COPADG in a dose-dependent manner (Figure 2A). The inhibitory effect started at 24 h, and reached maximum at 48 h (Figure 2B).
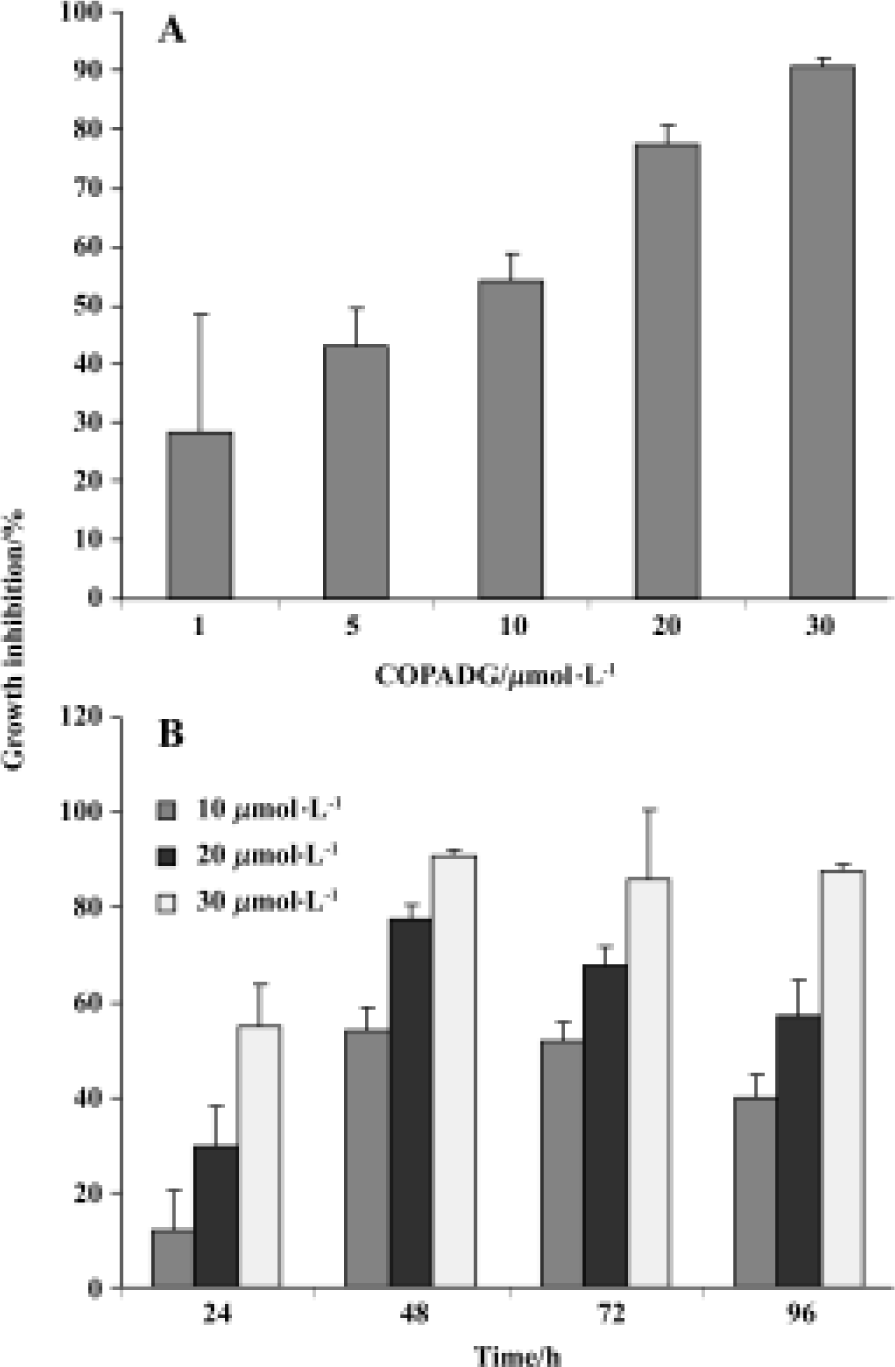
Apoptosis inhibition in HepG2 cells by COPADG
Apoptosis morphology by acridine orange fluorescence staining Apoptosis was first detected by typical morphology after staining with acridine orange (AO), one of the early techniques used for apoptosis detection. When HepG2 cells were treated with 20 µmol/L of COPADG for 48 h, cells with condensed or fragmented chromatin indicative of apoptosis were frequently observed, as compared to control cells which showed evenly distributed yellowish-green fluorescent chromatin (Figure 3).
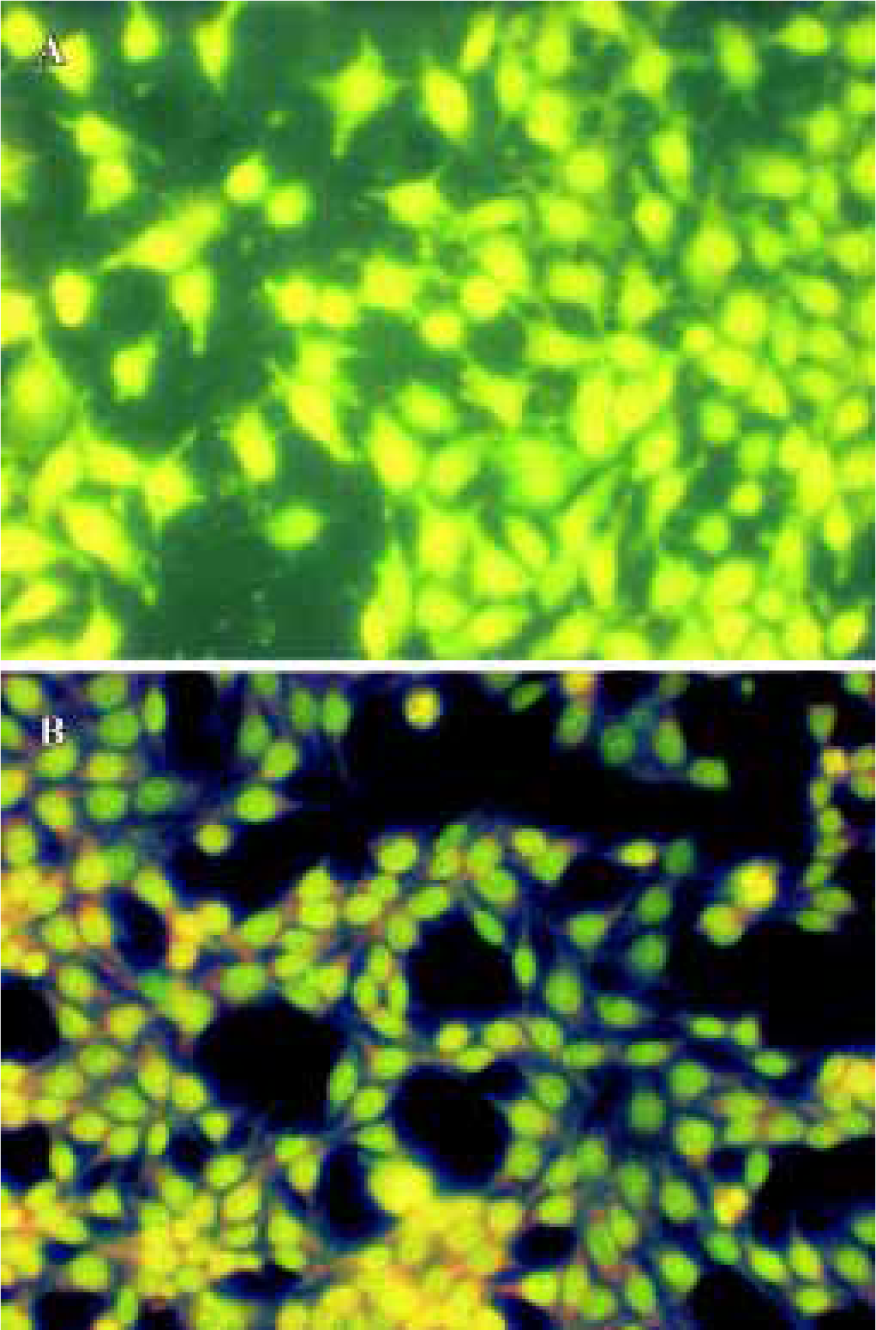
Induction of apoptosis by COPADG was in a dose- as well as a time-dependent manner, with 30 mmol/L of COPADG induced approximately 60% of apoptosis at 48 h. Figure 4 showed the quantitative results of COPADG-induced apoptosis in HepG2 cells.
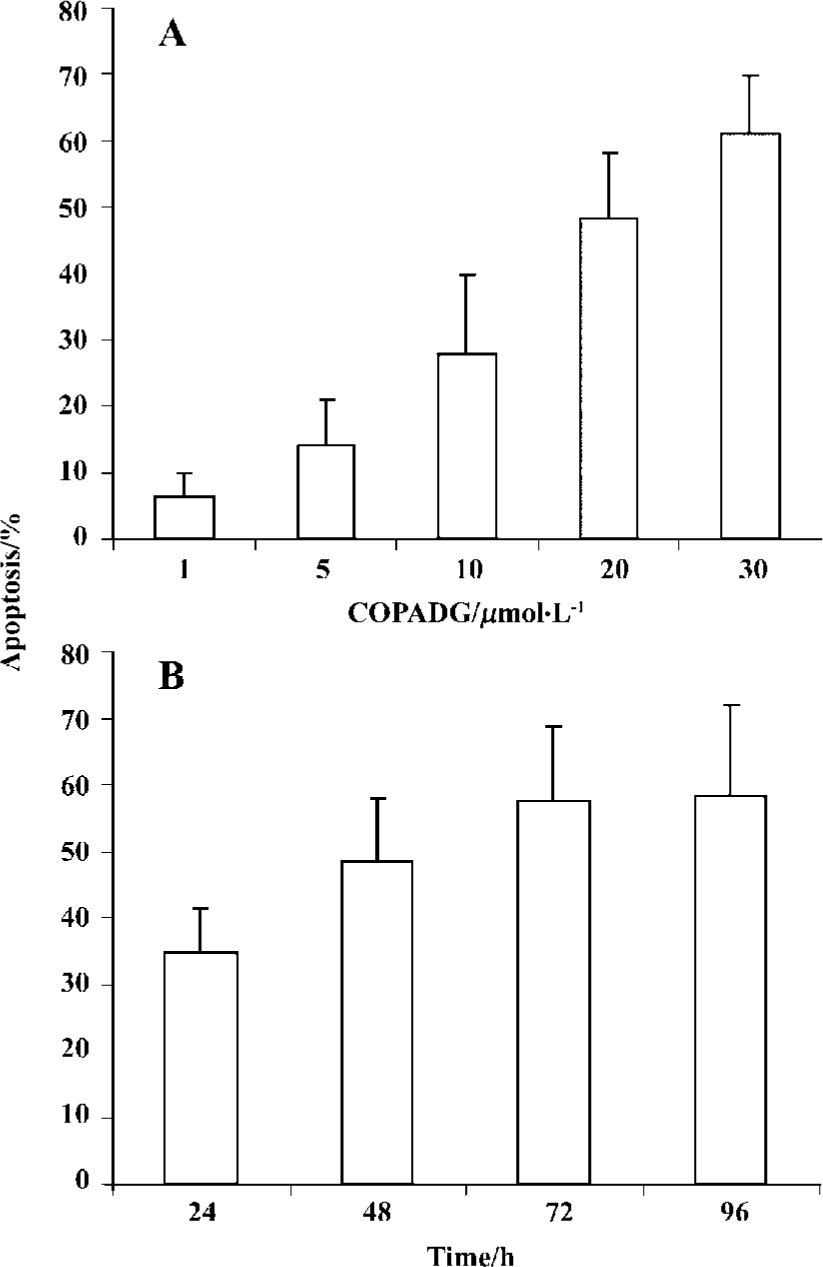
Ultrastructure of apoptosis by transmission electron microscopy (TEM) At the ultrastructural level, features of apoptosis were also observed by TEM. In HepG2 cells treated with 20 µmol/L of COPADG for 48 h, some nuclei displayed characteristic changes of apoptosis: chromatin condensation and margination, as well as nuclear fragmentation, cell shrinkage and cell blebbing (Figure 5). The intracellular organelles such as endoplasmic reticulum become loose and fuse with cell membrane resulting in vacuolation. These changes were in sharp contrast to untreated HepG2 cells, which showed normal cell membrane and nuclei with evenly distributed chromatin, as well as intact intracellular organelles.
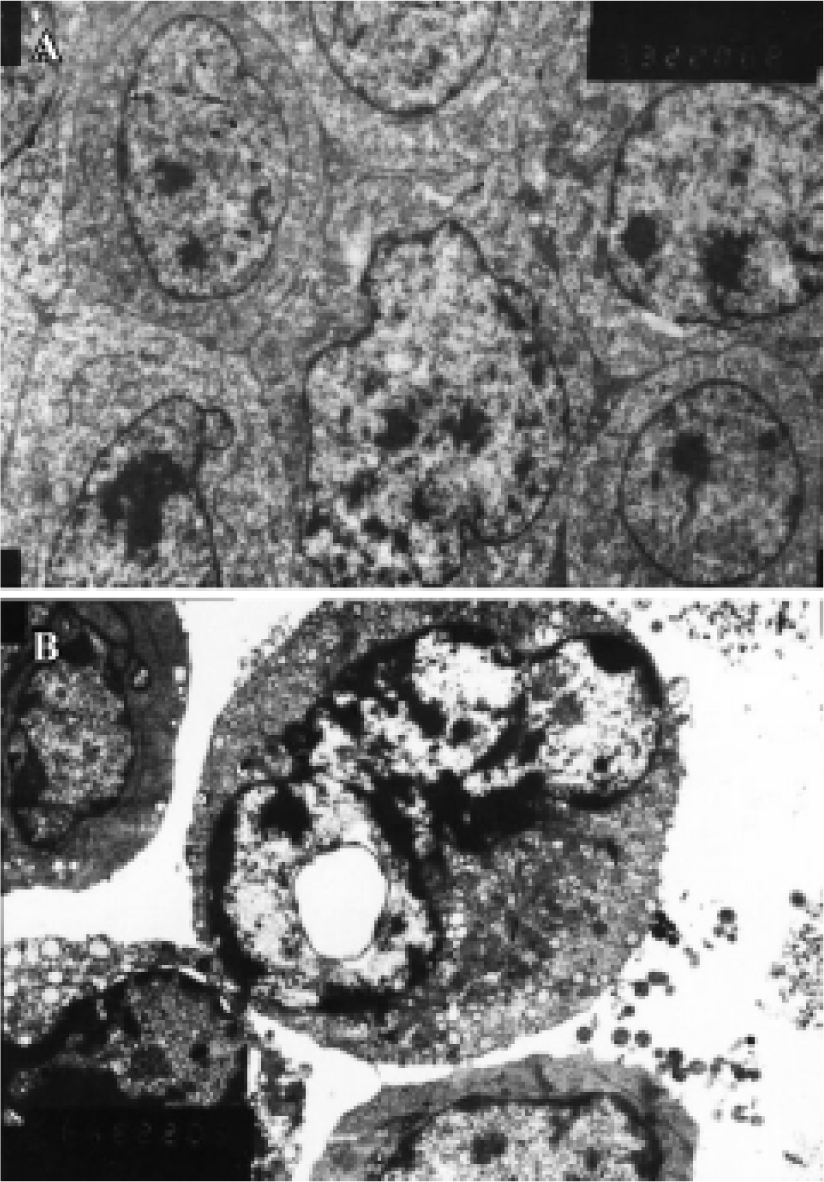
DNA fragmentation by agarose gel electrophoresis In order to investigate whether COPADG kills HepG2 cells by apoptosis, agarose gel electrophorsis of genomic DNA from COPADG-treated HepG2 cells were performed. As shown in Figure 6, typical DNA laddering patterns suggestive of apoptosis were observed when HepG2 cells were treated with 20–30 µmol/L of COPADG for 48 h (lanes 4 and 5, respectively).
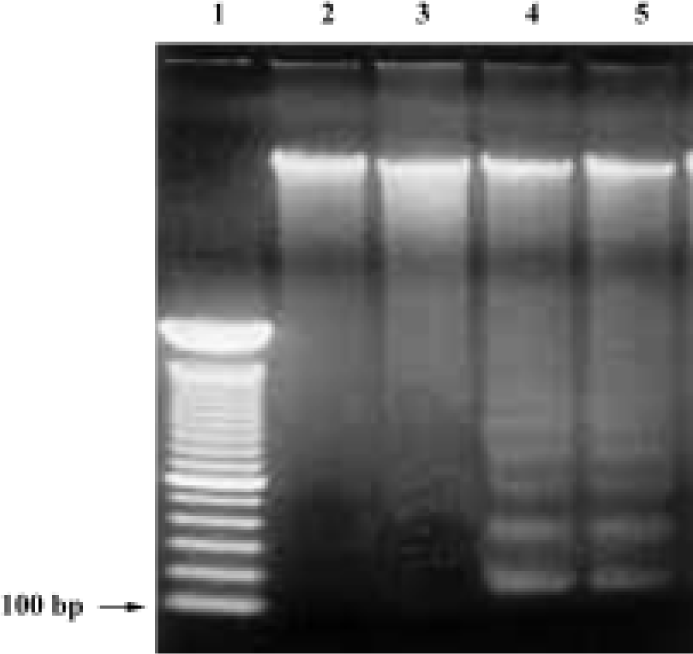
FACS analysis of apoptosis These biochemical features were verified by FACScan analysis, in which apoptosis was indicated by the presence of cells in the sub-G0/G1 peak. As shown in Figure 7 and Table 1, HepG2 cells treated with 10 µmol/L, 20 µmol/L, and 30 µmol/L of COPADG for 48 h displayed a dose-dependent accumulation of cells in the apoptosis peak (panel B, 17.4%; panel C, 26.8%; and panel D, 34.3%, respectively), as compared to untreated control (panel A).
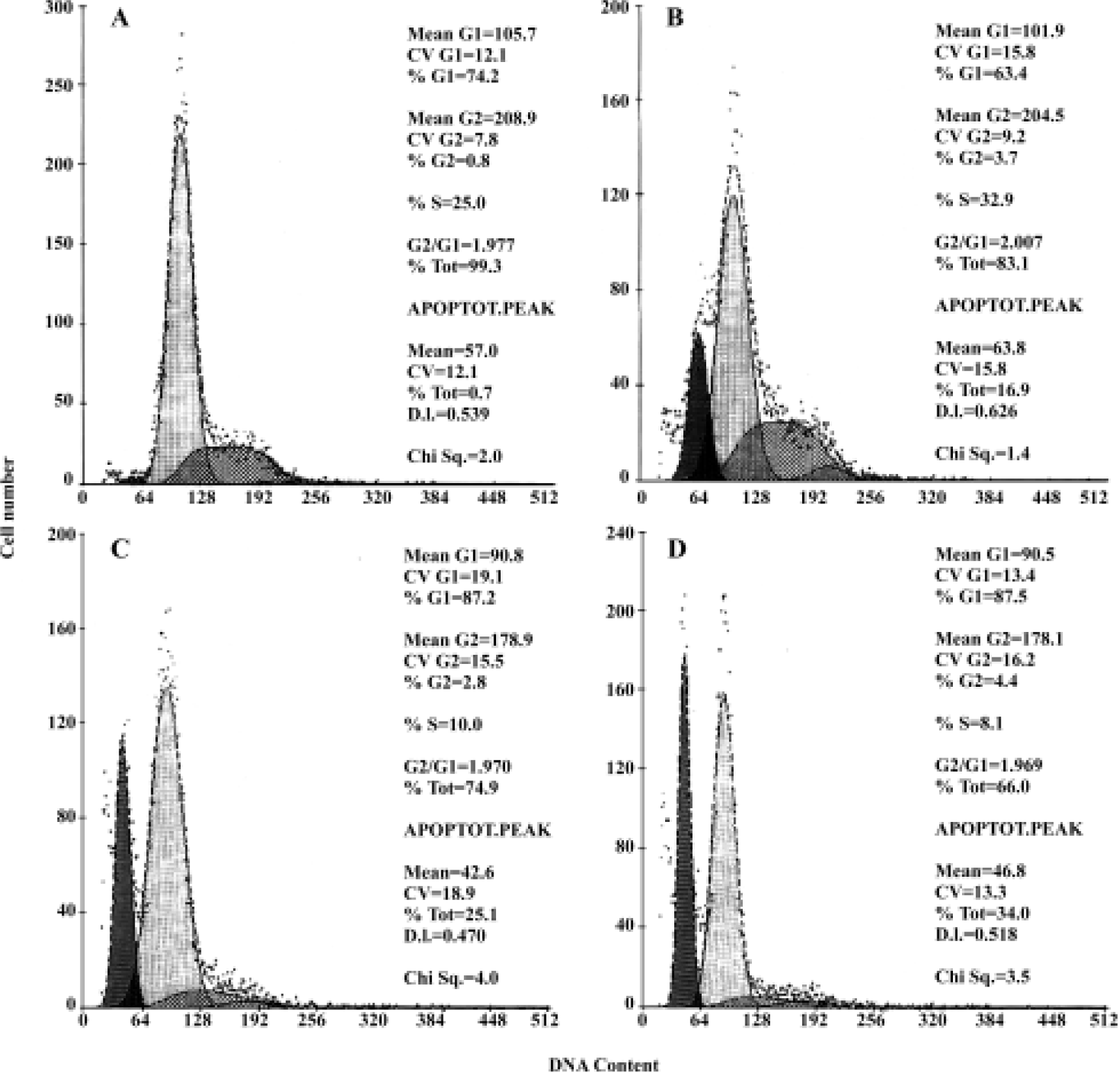
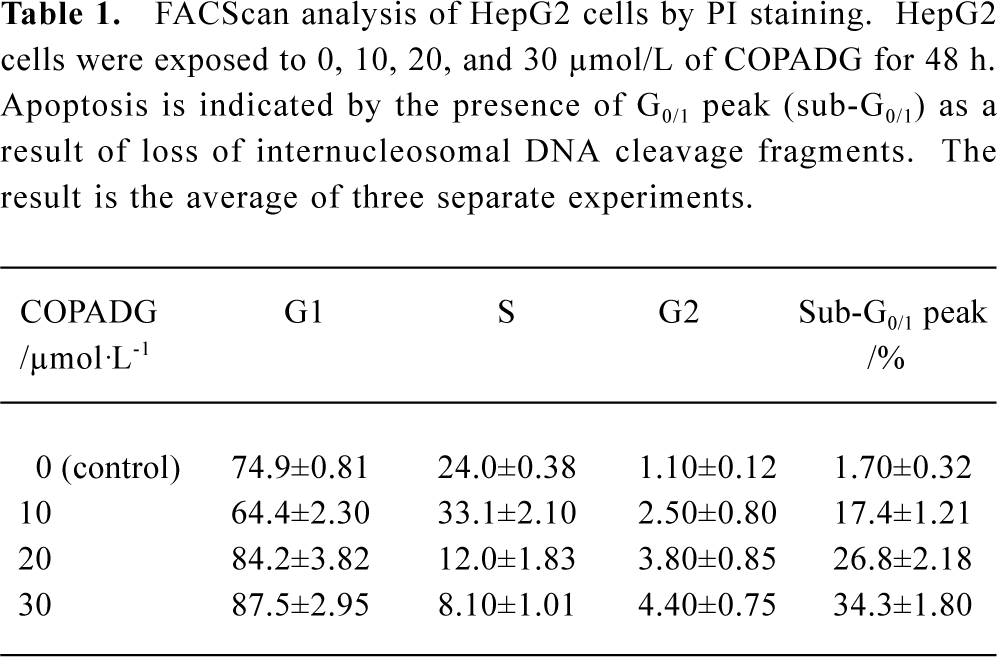
Full table
Discussion
D-glucose is a low molecular weight compound with multiple biological activities. Studies also have shown that D-glucose could inhibit tumor cell growth; some of the partial derivatives of D-glucose could potently induce the differentiation of tumor cells[10]. Our study was aimed to test whether the newest derivative of D-glucose (COPADG) had any effect on the proliferation and apoptosis in human hepatoma cells. We utilized several different methods to measure the effect of COPADG on cell proliferation and apoptosis. By MTT assay, it was shown that COPADG could effectively inhibit HepG2 cell proliferation in a dose-dependent manner. The inhibition of cell proliferation was associated with profound induction of cell apoptosis, as demonstrated by a series of complementary techniques for apoptosis detection, including morphologic study by acridine orange fluorescence staining and TEM, both showed typical morphology of apoptosis: cell pyknosis, chromatin condensation, and nuclear fragmentation. The nature of apoptotic cell death was also demonstrated by the presence of its biochemical feature (ie, typical “DNA ladder” on agarose gel electrophoresis indicating internucleosomal DNA fragmentation). These characteristics were further verified by FACS can analysis, which showed the presence of apoptotic peak in subG0/G1 peak. These results suggest that COPADG exerts its anti-tumor effects via two fundamental processes: suppression of cell proliferation and induction of apoptosis.
Under the physiological conditions, apoptosis is programmed cell dying process controlled by numerous genes[11–13]. The balance between well-controlled apoptosis and cell proliferation is an important determinant of cell fate. Thus, impaired apoptotic response or overactive proliferation is among the mechanisms of cancer initiation and progression. Human hepatocellular carcinoma (HCC) is one of the most common and chemoresistant cancers. At the present, there is no effective therapy against this deadly cancer, especially when it reaches advanced stage. Thus, novel and effective treatments are desperately needed. Agents or treatments that inhibit proliferation and /or promote apoptosis are under intensive investigation for their potential use in liver cancer therapy.
Our preliminary in vitro study suggested that COPADG could potently suppress proliferation and induce apoptosis in HepG2 cells. However, further studies are necessary to test the effect of this agent on other HCC cell lines or other types of cancer cells to make sure the effects we observed were not cell line specific. Studies are also warranted to elucidate the underlying mechanisms by which COPADG inhibits proliferation and induces apoptosis in HCC cells. In vivo studies in HCC-bearing nude mice may be also necessary to test the toxicity of this agent.
In conclusion, our current study has demonstrated that COPADG is a potent antiproliferative and proapoptotic agent against HepG2 cells. More studies are currently underway to expand these results to other cell lines.
References
- Thompson CB. Apoptosis in the pathogenesis and treatment of disease. Science 1995;10:1456-62.
- Carson DA, Riberio JM. Apoptosis and disease. Lancet 1993;341:1251-4.
- Kerr JF, Winterford CM, Harmon BV. Apoptosis. Its significance in cancer and cancer therapy. Cancer 1994;73:2013-26.
- Dive C, Hickman JA. Drug-target interactions: only the first step in the commitment to a programmed cell death? Br J Cancer 1991;64:192-6.
- Thompson HJ, Strange R, Schedin PJ. Apoptosis in the genesis and prevention of cancer. Cancer Epidemiol Biomarkers Prev 1992;1:597-602.
- Qiao Y, Wang AQ, Wang Z, Huang GS. The synthesis of 2-[(3-carboxy-1-oxoprogy1)amino]-2-deoxy-D-glucose. Chem Reagents 2004;26:107-8.
- Wang Z, Qiao Y, Huang GS, Wang AQ, Zhang YQ, Feng JL, et al. Glucosamine and glucosamine hydrochloride induced leukemia cell line K562 differentiation into macrophage. Chin Pharmacol Bull 2003;19:290-3.
- Abrams JM, White K, Fessler LI, Steller H. Programmed cell death during Drosophila embryogenesis. Development 1993;117:29-43.
- Qiao L, Farrell GC. Reciprocal control of apoptosis and proliferation in cultured rat hepatoma arl-6 cells: roles of nutrient supply, serum, and oxidative stress. Cell Dev Biol Anim 2000;36:465-75.
- Mc Donnel TJ, Meyn RE, Robertson LE. Implications of apoptotic cell death regulation in cancer therapy. Semin Cancer Biol 1995;6:53-60.
- Castaneda F, Kinne RK. Apoptosis induced in HepG2 cells by short exposure to millimolar concentrations of ethanol involves the Fas-respector pathway. J Cancer Res Clin Oncol 2001;127:418-24.
- Eichhorst ST, Muller M, Li-Weber M, Schulze-Bergkamen H, Angek P, Krammer PH. A novel AP-1 element in the CD95 ligand promoter is required for induction of apoptosis in hepatocellular carcinoma cells upon treatment with anticancer drugs. Mol Cell Biol 2000;20:7826-37.
- Lin HL, Liu TY, Chau GY, Lui WY, Chi CW. Comparison of 2-methoxyestradiol-induced, docetaxel-induced, and paclitaxel-induced apoptosis in hepatoma cells and its correlation with reaction oxygen species. Cancer 2000;89:983-94.