Magnesium lithospermate B ameliorates renal cortical microperfusion in rats1
Introduction
Magnesium lithospermate B (MLB) is a tetramer of caffeic acid. It was recently isolated from a plant used in Chinese herbal medicine, Salviae miltiorrhizae, and has been found to improve renal function and ameliorate experimental renal failure. The effect of MLB on renal function was first reported by Yokozawa et al[1,2], who studied these effects in several animal models of renal failure and suggested that MLB increased renal function by improving the renal circulatory state through activation of kallikrein, promotion of prostaglandin E2 production, and scavenging radicals in rats with renal failure[3–13]. Other researchers have confirmed the renoprotective property and free radical scavenging effect of MLB by using different animal models. Lee et al found that MLB suppressed the progression of renal injury in streptozotocin-induced diabetic rats, and inhibited reactive oxygen species generation that leads to protein kinase C activation and transforming growth factor (TGF)-1/fibronectin upregulation in mesangial cells cultured in high glucose conditions[14]. Kang et al demonstrated the strong inhibitive effect of MLB against the production of superoxide, hydrogen peroxide, and hydroxyl radicals, the three most common oxygen radicals, and suggested that MLB ameliorated renal defects in rats with ischemia-reperfusion-induced acute renal failure via scavenging of reactive oxygen species[15]. Wu et al reported that MLB was an inhibitor of lipid peroxidation and scavenged superoxide anions and hydroxyl radicals both in vitro and ex vivo[16]. Soung et al demonstrated that MLB with a hydroxyl group and double bonds exerted an anti-nitration effect by scavenging peroxynitrite[17].
Although the renoprotective effects of MLB have been primarily attributed to scavenging oxygen free radicals, other protective mechanisms may play a role as well. Taking the importance of renal microcirculation in maintaining normal renal function into account, we hypothesized that MLB might ameliorate renal microcirculation, which is a more direct way to improve renal function. The purpose of the present study, therefore, was to describe the hemodynamic action of MLB with respect to the specificity of renal hemodynamics and microcirculation in rats.
Materials and methods
Drugs MLB was provided by the Department of Phytochemistry, Shanghai Institute of Materia Medica, Chinese Academy of Sciences. The purity of this compound was above 99.8%, which was verified by the supplier using high performance liquid chromatography methods.
Hemodynamics and renal microcirculation Studies of hemodynamics and renal circulation were carried out in 8 healthy 9−10-week-old male Sprague-Dawley rats weighing 292±6 g, which were purchased from the Shanghai SLAC Laboratory Animal Co (Certificate N
After surgery, the animals were allowed to recover for 30 min. Then, animals were injected intravenously with vehicle control (saline), and MLB at doses of 10, 30, and 60 mg/kg consecutively, with 45 min between injections.
Mean values for each determination were analyzed over a 0.5-min to 1-min period. Renal vascular resistance was calculated from the mean arterial pressure and the corresponding renal blood flow.
Statistical analysis Data were given as mean±SD, from 8 animals in each group. The statistical significance of differences in the hemodynamic parameters was assessed using one-way analysis of variance (ANOVA). Student’s t-test was used for comparison of the parameters with their baseline values. Statistical significance was set at P<0.05.
Results
Effects on renal microcirculation and hemodynamics There was no difference between groups with respect to the baseline values of renal hemodynamic and microcirculation parameters (Figure 1). Neither vehicle nor MLB administration had any significant effect on renal blood flow or renal vascular resistance. In contrast, MLB administration increased renal cortical microperfusion significantly, whereas the vehicle had no effect (Figure 1, Tables 1, 2). This microcirculation improving effect reached its peak 15 min after injection and returned to baseline after 45 min. Fifteen minutes after injection with 10, 30, or 60 mg/kg MLB, renal cortical microperfusion increased by 38.7%±27.3%, 51.4%±22.2%, and 62.4%±20.2%, respectively (changes relative to baseline; P<0.01), whereas renal blood flow (2.3%±19.9%, 1.6%±20.4%, 3.7%±9.7%, respectively) and renal vascular resistance (-8.4%±13.8%, -1.6%±12.4%, -1.4%±9.1%, respectively) did not change significantly.
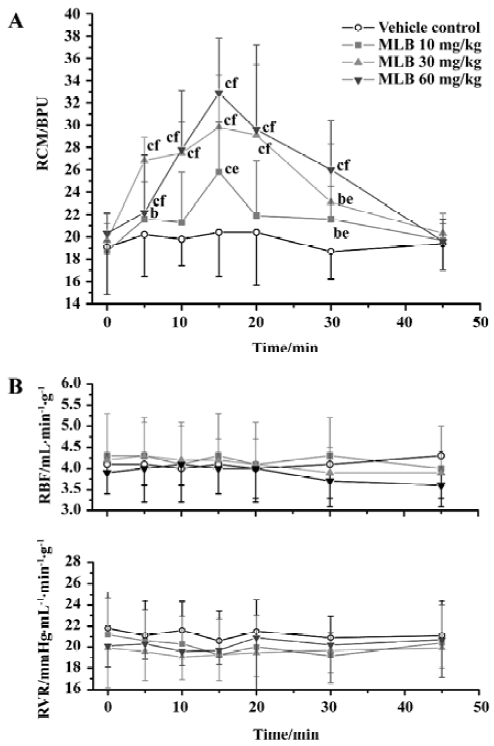
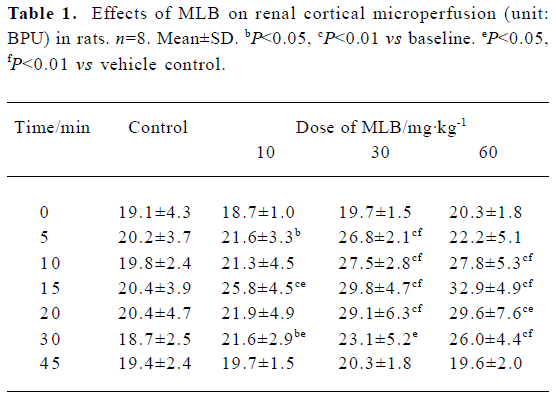
Full table
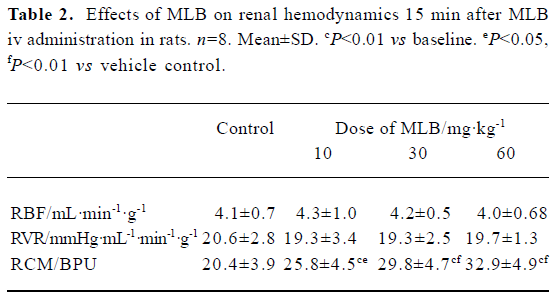
Full table
Effects on systemic hemodynamics There was no difference between groups with respect to the baseline values of systemic hemodynamic parameters (Figure 2). Vehicle administration had no effect on these parameters. Although MLB administration had some effect on these parameters at some time points, this effect was neither time-dependent nor dose-dependent. Fifteen minutes after injection with MLB 60 mg/kg, when the effect of MLB on renal cortical micro-perfusion had reached its peak, mean arterial pressure (1.9%±10.2% vs baseline), heart rate (0.2%±5.4% vs baseline), LVSP (0.4%±7.8% vs baseline), LVEDP (7.8%±33.4% vs baseline) and +dp/dtmax (3.0%±6.8% vs baseline) had not changed in a statistically significant way, whereas -dp/dtmax increased slightly (5.7%±6.2%; P<0.05 vs baseline).
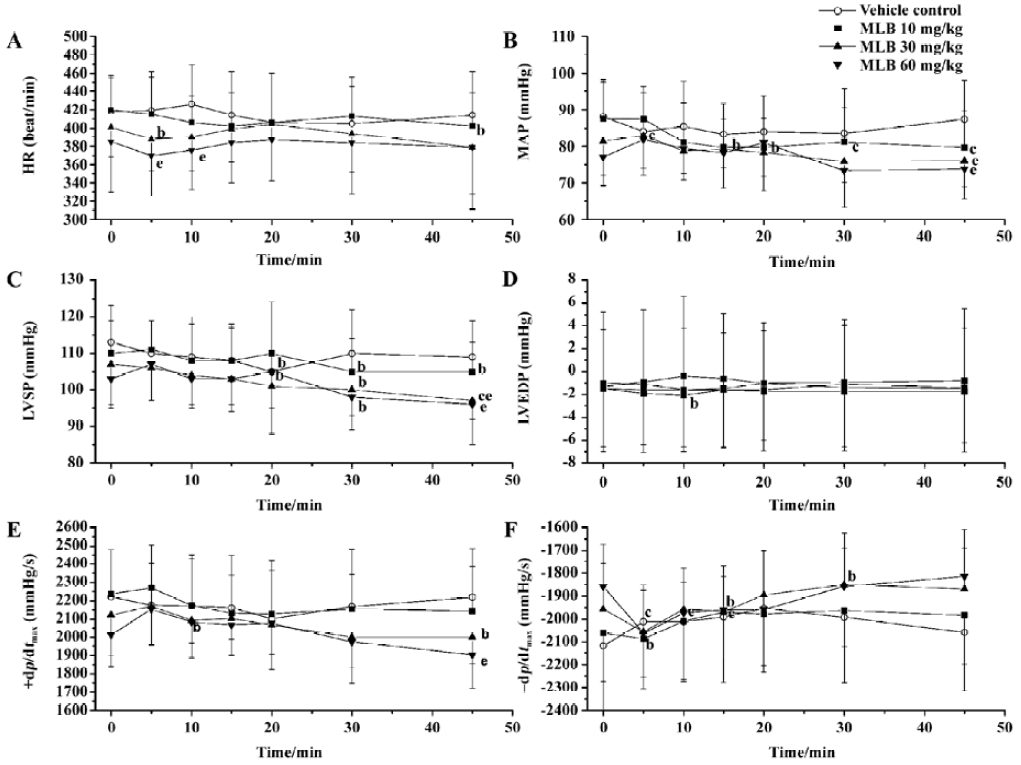
Discussion
The kidneys play a central role in the regulation of the body’s salt and water balance. A highly regulated microcirculatory and interstitial environment is essential for optimum function of the kidneys. Although the renal function improving property of MLB has been studied extensively, there has been no report concerning its effect on renal microcirculation. We demonstrated here, to our knowledge for the first time, that MLB could ameliorate renal microcirculation while causing no other significant changes to hemo-dynamics.
In our study, the effect of intravenously administered MLB on renal cortical microperfusion was dose-dependent, and reverted back to the baseline level 45 min after MLB administration. Li et al reported the pharmacokinetic parameters of MLB after iv administration in 6 beagle dogs, and showed that MLB was distributed and eliminated quickly[21]. The mean T1/2β values for MLB at doses of 3 mg/kg, 6 mg/kg, and 12 mg/kg were 43±9 min, 42±7 min, and 42±10 min, respectively. Therefore, the time-response curve of MLB was correlated with its serum concentration-time profiles.
This effect of MLB on renal circulation is consistent with previous studies. However, some differences exist. Yokozawa et al reported that MLB increased renal blood flow[20], which we did not find in our study. The different animal models and techniques we used may account for this difference. Yokozawa et al used rats with renal failure, which had significantly lower renal blood flow than normal rats, whereas we used normal Sprague-Dawley rats with normal renal blood flow. The techniques we used to measure renal blood flow were also different. Yokozawa et al used a needle-type bipolar electrode electrolytic organ rheometer, whereas we used a transit-time ultrasonic-Doppler flow meter with a much higher precision (±5%).
The effect of MLB on renal microcirculation may be attributed to several factors. The primary contributor may be its potent antioxidant properties. Recent studies suggest that free radicals may play a key role in regulating renal microvascular tone. Although studies investigating the roles of oxygen radicals in the physiological regulation of renal microcirculation have only recently begun, it is evident that oxygen radicals have important direct and indirect actions in both cortical and medullary microcirculation[22–25]. Because O2– and NO both contain unpaired electrons in their outer orbits, they undergo extremely rapid, diffusion-limited radical-radical reactions, leading to the formation of peroxynitrite anions (ONOO–), strong oxidants that could prompt the generation of hydroxyl radicals (OH–). In the renal microva-sculature, free radicals can cause vasoconstriction, mediate the vasoconstriction of other agonists, and modulate the action of vasodilators (inactivate nitric oxide and blunt endothelium-dependent vasodilation)[26–28]. These findings have led to the idea that antioxidants might be used therapeutically as part of a nephroprotective strategy[29–31]. Our current study supports this idea: MLB was proven to be a potent inhibitor of the production of superoxides, hydrogen peroxide, and hydroxyl radicals, the three most common oxygen radicals in the renal microvasculature. Here we propose that MLB, as a potent antioxidant, scavenges free radicals, blocks the O2–_ONOO–_OH– cascade, promotes NO bioavailability and thus ameliorates renal microcirculation.
Some other factors may play a role too. MLB has been reported to improve the renal circulatory state through activation of kallikrein and promotion of prostaglandin E2 production[7,9,13]. Tissue kallikrein cleaves the kininogen substrate to release the vasoactive peptide kinin, which binds to endothelial bradykinin B2 receptors and stimulates the release of potent vasodilators, including prostacyclin, nitric oxide, and endothelium-derived hyperpolarizing factor[32,33]. The paracrine agent PGE2 is the predominant cyclooxygenase metabolite of arachidonic acid in the kidney[34]. PGE2 plays an important role in tubular reabsorption of salt and water as well as in the control of renal vascular resistance and the maintenance of glomerular hemodynamics. Despite several reports of PGE2-induced vasoconstriction[35,36], there is convincing evidence that PGE2 acts primarily on the preglomerular vasculature to counteract the effects of the vasoconstricting hormones and protect the kidney from excessive vasoconstriction[37–41]. Although the effect of MLB on cyclooxygenase has not been studied, it is reported to be a potent inhibitor of 5-lipoxygenase, and such inhibition of lipoxygenase causes a shift of arachidonic acid from the lipoxygenase to the cyclooxygenase pathway, which is thought to result in increased formation of cyclooxygenase metabolites[3]. Indeed, Yokozawa et al reported that MLB increased urinary excretion of prostaglandin E2 (PGE2) and 6-keto-PGF1α, while thromboxane B2 (TXB2) remained unchanged or decreased in rats with renal failure[3]. Activation of kallikrein, promotion of PGE2 production, and scavenging of radicals could act simultaneously to increase the bioavailability of NO and prostacyclin, the major vasodilators in the kidney. These three effects are suggested to be the major contributors to increased renal microcirculation after MLB administration. Nonetheless, the exact mechanism by which MLB ameliorates renal cortical microperfusion is not completely clear, and should be evaluated further.
In conclusion, the major finding of this study is that intravenously administered MLB dose-dependently ameliorates renal microcirculation. This finding suggests that the renal protective properties of MLB may be mediated in part by vasodilation of the renal microvasculature.
References
- Yokozawa T, Chung HY, Oura H, Nonaka G, Nishioka I. Isolation of the active component having the uremia-preventive effect from Salviae miltiorrhizae radix extract. Chem Pharm Bull (Tokyo) 1988;36:316-20.
- Yokozawa T, Chung HY, Oura H, Nonaka G, Nishioka I. Effect of (Dihydro) caffeic acid tetramer isolated from Salviae miltiorrhizae. J Med Pharm Soc for Wakan-Yaku 1988;5:163-6.
- Yokozawa T, Chung HY, Lee TW, Oura H, Tanaka T, Nonaka G, et al. Contribution of prostaglandins to the renal responses to magnesium lithospermate B isolated from Salviae miltiorrhizae radix. Chem Pharm Bull (Tokyo) 1989;37:1568-71.
- Yokozawa T, Chung HY, Lee TW, Oura H, Tanaka T, Nonaka G, et al. Effect of magnesium lithospermate B on urinary excretion of arachidonate metabolites in rats with renal failure. Chem Pharm Bull (Tokyo) 1989;37:2766-9.
- Yokozawa T, Chung HY, Oura H, Nonaka G, Nishioka I. Isolation of a renal function-facilitating constituent from the oriental drug, Salviae miltiorrhizae radix. J Nephrol 1989;31:1091-8.
- Yokozawa T, Lee TW, Chung HY, Oura H, Nonaka G, Nishioka I. Effect of magnesium lithospermate B on urinary prostaglandins in rats with renal failure. J Nat Prod 1990;53:662-5.
- Yokozawa T, Chung HY, Lee TW, Oura H, Nonaka G, Nishioka I. Magnesium lithospermate B improves renal function via the kallikrein-prostaglandin system in rats with renal failure. J Nephrol 1990;32:893-8.
- Yokozawa T, Lee TW, Chung HY, Oura H, Nonaka G, Nishioka I. Renal response to magnesium lithospermate B. J Pharm Pharmacol 1990;42:712-5.
- Yokozawa T, Oura H, Lee TW, Nonaka G, Nishioka I. Augmentation of renal response by magnesium lithospermate B. Nephron 1991;57:78-83.
- Yokozawa T, Lee TW, Oura H, Nonaka G, Nishioka I. Effect of magnesium lithospermate B in rats with sodium-induced hypertension and renal failure. Nephron 1992;60:460-5.
- Yokozawa T, Dong E, Liu ZW, Shibata T, Hasegawa M, Watanabe H, et al. Magnesium lithospermate B ameliorates cephaloridine-induced renal injury. Exp Toxicol Pathol 1997;49:337-41.
- Yokozawa T, Dong E, Oura H, Kashiwagi H, Nonaka G, Nishioka I. Magnesium lithospermate B suppresses the increase of active oxygen in rats after subtotal nephrectomy. Nephron 1997;75:88-93.
- Yokozawa T, Lee TW, Oura H, Nonaka G, Nishioka I, Hattori M. Effect of magnesium lithospermate B on the renal and urinary kallikrein activities in rats with adenine induced renal failure. Nippon Jinzo Gakkai Shi 1993;35:337-42.
- Lee GT, Ha H, Jung M, Hong SW, Cha BS, Lee HC, et al. Delayed treatment with lithospermate B attenuates experimental diabetic renal injury. J Am Soc Nephrol 2003;14:709-20.
- Kang DG, Oh H, Sohn EJ, Hur TY, Lee KC, Kim KJ, et al. Lithospermic acid B isolated from Salvia miltiorrhiza ameliorates ischemia/reperfusion-induced renal injury in rats. Life Sci 2004;75:1801-6.
- Wu XJ, Wang YP, Wang W, Sun WK, Xu YM, Xuan LJ. Free radical scavenging and inhibition of lipid peroxidation by magnesium lithospermate B. Acta Pharmacol Sin 2000;21:855-8.
- Soung do Y. Peroxynitrite scavenging activity of lithospermate B from Salvia miltiorrhiza. J Pharm Pharmacol 2003;55:1427-32.
- Heyman SN, Fuchs S, Jaffe R, Shina A, Ellezian L, Brezis M, et al. Renal microcirculation and tissue damage during acute ureteral obstruction in the rat: effect of saline infusion, indomethacin and radiocontrast. Kidney Int 1997;51:653-63.
- Lien B, Norstein J, Salerud EG, Kvernebo K, Flatmark A. Renal microvascular perfusion evaluated by single fibre laser Doppler flowmetry. Int J Microcirc Clin Exp 1992;11:307-17.
- Yokozawa T, Lee TW, Oura H, Zhang XY, Hattori M, Nonaka G, et al. Haemodynamic effect of magnesium lithospermate B in rats with renal failure. Phytother Res 1992;6:194-9.
- Li XC, Yu C, Sun WK, Liu GY, Jia JY, Wang YP. Pharmacokinetics of magnesium lithospermate B after intravenous administration in beagle dogs. Acta Pharmacol Sin 2004;25:1402-7.
- Schnackenberg CG, Welch WJ, Wilcox CS. TP receptor-mediated vasoconstriction in microperfused afferent arterioles: roles of O2– and NO. Am J Physiol Renal Physiol 2000;279:F302-8.
- Wilcox CS, Welch WJ. Interaction between nitric oxide and oxygen radicals in regulation of tubuloglomerular feedback. Acta Physiol Scand 2000;168:119-24.
- Zou AP, Li N, Cowley AW Jr. Production and actions of superoxide in the renal medulla. Hypertension 2001;37:547-53.
- Ozawa Y, Hayashi K, Wakino S, Kanda T, Homma K, Takamatsu I, et al. Free radical activity depends on underlying vasoconstrictors in renal microcirculation. Clin Exp Hypertens 2004;26:219-29.
- Schnackenberg CG, Welch WJ, Wilcox CS. Normalization of blood pressure and renal vascular resistance in SHR with a membrane-permeable superoxide dismutase mimetic: role of nitric oxide. Hypertension 1998;32:59-64.
- Schnackenberg CG, Welch WJ, Wilcox CS. TP receptor mediated vasoconstriction in microperfused afferent arterioles: roles of O2– and NO. Am J Physiol Renal Physiol 2000;279:F302-8.
- Schoonmaker GC, Fallet RW, Carmines PK. Superoxide anion curbs nitric oxide modulation of afferent arteriolar ANG II responsiveness in diabetes mellitus. Am J Physiol Renal Physiol 2000;278:F302-9.
- Schnackenberg CG. Physiological and pathophysiological roles of oxygen radicals in the renal microvasculature. Am J Physiol Regul Integr Comp Physiol 2002;282:R335-42.
- Tylicki L, Rutkowski B, Horl WH. Antioxidants: A possible role in kidney protection. Kidney Blood Press Res 2003;26:303-14.
- Vaziri ND. Roles of oxidative stress and antioxidant therapy in chronic kidney disease and hypertension. Curr Opin Nephrol Hypertens 2004;13:93-9.
- Bhoola KD, Figueroa CD, Worthy K. Bioregulation of kinins: kallikreins, kininogens, and kininases. Pharmacol Rev 1992;44:1-80.
- Hornig D, Drexler H. Endothelial function and bradykinin in humans. Drugs 1997;54:42-7.
- Bonvalet JP, Pradelles P, Farman N. Segmental synthesis and actions of prostaglandins along the nephron. Am J Physiol Renal Physiol 1987;253:F377-87.
- Baer PG, McGiff JC. Comparison of effects of prostaglandins E2 and I2 on rat renal vascular resistance. Eur J Pharmacol 1979;54:359-63.
- Inscho EW, Carmines PK, Navar LG. Prostaglandin influences on afferent arteriolar responses to vasoconstrictor agonists. Am J Physiol Renal Physiol 1990;259:F157-63.
- Arendshorst WJ, Beierwaltes WH. Renal and nephron hemodynamics in spontaneously hypertensive rats. Am J Physiol Renal Physiol 1979;236:F246-51.
- Folkow B, Gothberg G, Ludin S. Structural “resetting” of the renal vascular bed in spontaneously hypertensive rats. Acta Physiol Scand 1977;199:270-2.
- Chatziantoniou C, Arendshorst WJ. Prostaglandin interactions with angiotensin, norepinephrine, and thromboxane in rat renal vasculature. Am J Physiol Renal Physiol 1992;262:F68-76.
- Jackson EK, Heidemann HT, Branch RA, Gerkens JF. Low dose intrarenal infusions of PGE 2, PGI2, and 6-keto-PGE1 vasodilate the in vivo rat kidney. Circ Res 1982;51:67-72.
- Lonigro AJ, Terragno NA, Malik KU, McGiff JC. Differential inhibition by prostaglandins of the renal actions of pressor stimuli. Prostaglandins 1973;3:595-606.