Effects of orexins on myoelectric activity of sphincter of Oddi in fasted rabbits1
Introduction
The sphincter of Oddi (SO) is situated at the junction of the bile and pancreatic ducts, where they enter the duodenum, and it serves to regulate the flow of bile and pancreatic juices as well as to prevent the reflux of duodenal contents into the pancreatobiliary system[1]. Although the existence of the SO has been known for more than a century, our knowledge about the physiological functions, pathological features, and modulation of SO is still inadequate.
The orexins are a pair of neuropeptides (orexin-A and orexin-B; OXA and OXB) involved in the regulation of appetite and arousal. These neuropeptides are produced by a small group of neurons in the lateral hypothalamic area, a region involved in the control of mammalian feeding behavior[2–4]. Several lines of evidence have shown that the peripheral orexin system can modulate digestive organs. For example, orexin has been found to increase the myoelectric motor complex (MMC) cycle length of the duodenum and stimulate motility in isolated guinea pig colon[5,6], and play an important role in the regulation of gastric motility via both the brain-gut axis and the enteric nervous system (ENS)[7–10]. Furthermore, it can stimulate the secretion of gastric acid and pancreatic juice[11,12]. However, the effects of orexins on SO have received little attention.
Given that orexins can increase the MMC cycle length of the duodenum and stimulate motility of isolated colon[5,6], it is probable that it also has effects on SO. In the present study, we examined the effects of peripheral OXA and OXB on myoelectric activity of SO in fasted rabbits, and then made a preliminary attempt to explore the mechanisms underlying such effects.
Materials and methods
Animals All experiments were performed in accordance with Chinese Animal Welfare Legislation, and the Third Military Medical University’s Committee on Ethics in the Care and Use of Laboratory Animals approved the experimental procedures. Adult rabbits (n=84) of both sex weighing approximately 2.0 kg were housed in a laboratory in which the temperature was regulated to 22 °C. Each animal was fasted for 12 h prior to the experiments being undertaken.
Rabbits were randomly divided into 5 groups. As a control for the tested drugs, 0.9% saline (the same volume as used for the tested drugs) was administered through the ear vein in 6 rabbits. Animals in the second group were intravenously injected with OXA or OXB at 4 different doses. The doses of OXA or OXB used were 0.5, 1, 2, and 4 nmol/kg, respectively. Members of the third group were injected with 10 µL saline in the subsera of the SO. Controls received no further treatment, but members of the treatment group received 0.4 nmol OXA or OXB (10 µL) injected at the same site 5 min later. In the fourth group, the muscarinic receptor antagonist atropine (0.1 mg/kg) was used intravenously in 6 animals. In the fifth group, OXA (4 nmol/kg) or OXB (4 nmol/kg) was administered systemically as above, and then atropine (0.1 mg/kg) was injected intravenously soon after (within approximately 1 min). Each intravenous application was in a total volume of 40 µL/kg.
Electrical recordings Each rabbit was anesthetized with urethane (3%, 4 mL/kg) through an ear vein, and then fixed on a rabbit table. The peritoneal cavity was opened with a right mid-rectus incision. Then the duodenum was stitched to the abdominal wall after it was rotated 90–120° clockwise. In this way, the SO was exposed.
All electrical experiments were carried out in a PBG-1 physiological experiment shield cabinet, as described previously[13,14]. Briefly, two pairs of bipolar stainless steel electrodes were implanted into subsera of the SO and duodenum respectively. The electrodes were connected to a multi-channel physiological recording and signal processing system (RM6240; Chengdu Instrument Factory, Chengdu, China). Recordings were then controlled and monitored with a Pentium 3 personal computer. The time constant was set at 1 s, the velocity was set at 500 mV/div, the sensitivity was set at 1.00 mV, and the initial high-cutoff frequency was set at 10 Hz. Prior to analysis, all signals, unless otherwise stated, were digitally high-pass filtered at 10 Hz to remove the noise signals of gastrointestinal electrical activity and breathing activity. Baseline activity was recorded for 10 min before the drugs were administered, and recordings lasted for at least 30 min following administration.
Drugs and data analysis All of the chemicals were obtained from Sigma (St Louis, MO, USA). All the drugs were dissolved in saline and mixtures were prepared fresh daily. The spike potential of the SO (SPSO) was defined as a clearly distinguishable activity of which the frequency and amplitude were calculated to describe the changes in myoelectric activity of the SO. The frequency of SPSO is given as mean±SEM, and the amplitude was calculated as the range in the same period. Statistical comparisons were carried out by using Student’s t-test with SPSS (version 10) software, as appropriate. A minimum P value of <0.05 was selected to determine significance.
Results
Basal myoelectric activity All experiments started with a control recording of basal myoelectric activity for 10 min, during which time original activities of both the duodenum and the SO were observed to ensure that the myoelectric activity of the SO was different from that of the duodenum. To obtain original activities of the duodenum, these signals were not digitally high-pass filtered. In the control recording of basal myoelectric activity, single, regular SPSO with frequencies ranging from 0.17 to 0.88 Hz (mean frequency, 0.45±0.15 Hz, n=78), amplitudes of 65–257 µV, and maximum potentials of 320–350 µV were observed (Figure 1).
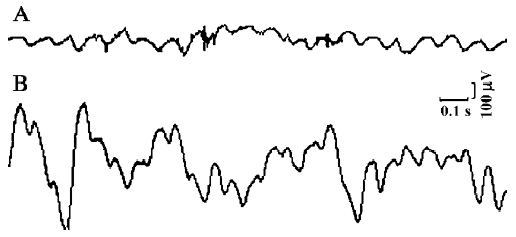
Excitatory effects of orexins on myoelectric activity of the SO The frequency and amplitude of SPSO after intravenous injection of OXA (4 nmol/kg) were obviously increased in 0.5 min after injection (n=6, P<0.01) relative to the same parameters after the injection of saline (Figure 2A, 2D). In some experiments (n=3), SPSO clusters with 4–7 single SPSO in each were observed. Also, intravenous application of 4 nmol/kg OXB had markedly excitatory actions on the myoelectric activity of the SO (n=6, P<0.01) (Figure 2C, 2D). As shown in Figure 2D, the response induced by OXA or OXB (4 nmol/kg) rose to a peak rapidly during the first 3 min, and then declined and gradually approached the control level during the subsequent 9 min. Furthermore, the myoelectric activities of the SO were tested with 4 different concentrations of orexins, and their effects were found to be dose-dependent. As illustrated by the concentration-response curve presented in Figure 2E, 4 nmol/kg OXA produced potent effects on the myoelectric activity of the SO (n=6, P<0.01), whereas 2 nmol/kg OXA produced a weaker response (n=6, P<0.01). Doses of 0.5 nmol/kg and 1 nmol/kg had no effect (n=6, P>0.05, respectively). Similarly, OXB also dose-dependently activated the myoelectric activity of the SO (data not shown). Therefore, these data indicate that orexins can exert a dose-dependent excitatory effect on the myoelectric activity of the SO.
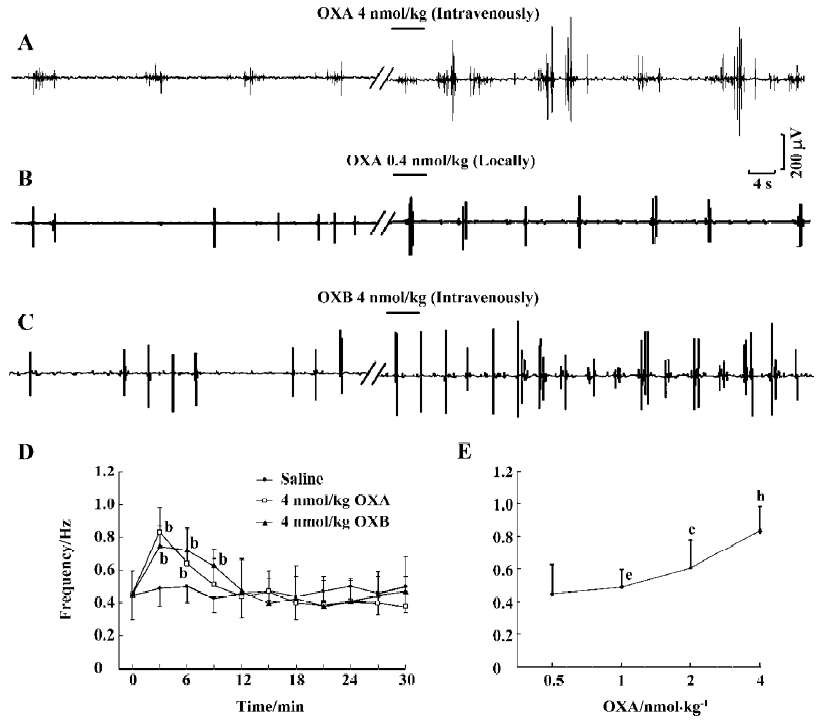
Local injection of 10 µL saline (0.9%) had no significant influence on the frequency or amplitude of SPSO. In contrast, the frequency was obviously increased after local application of 0.4 nmol OXA (n=6, P<0.05) (Figure 2B) or 0.4 nmol ORB (n=6, P<0.05) (data not shown).
These results indicate that both intravenous and local injections of orexins produce modulatory effects on the SO.
Antagonistic effects of atropine on the excitatory effects of OXA Because the SO is richly innervated by cholinergic neurons and the enteric cholinergic system plays an important role in the regulation of SO motor activity[15], we hypothesized that the effects of orexins on SO motility might be associated with cholinergic modulation. To investigate this, we further tested the actions of atropine, a muscarinic receptor antagonist, on the orexin-increased myoelectric activity of the SO. First, when atropine (0.1 mg/kg) was intravenously administered alone, a complete antagonistic effect on the myoelectric activity of the SO was observed (n=6, P<0.01) (Figure 3A). Intravenous administration of atropine (0.1 mg/kg) also fully antagonized the excitatory effects of OXA (n=6, P<0.01; Figure 3B, 3C) and OXB (n=6, P<0.01; Figure 3C) on the myoelectric activity of the SO. These observations thus strongly imply that the excitatory effects of orexins on the myoelectric activity of the SO are partially, at least, dependent on cholinergic pathways.
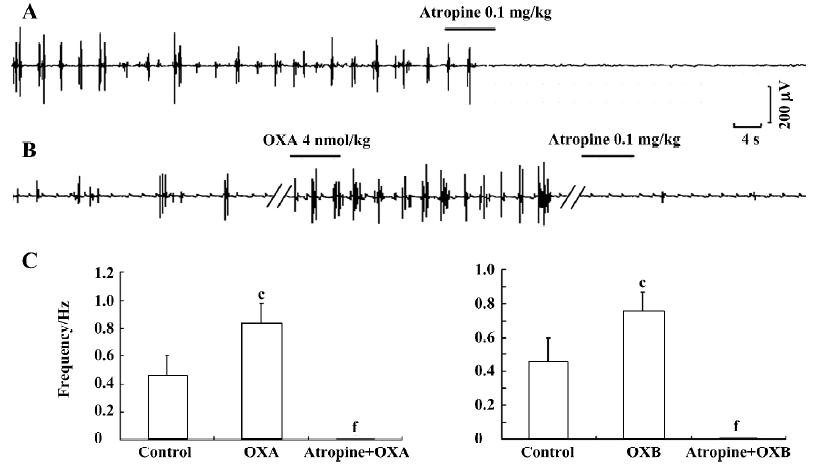
Discussion
The orexin system is generally considered to control food intake at the level of the hypothalamus. Interestingly, it has been shown that orexins and orexin receptors (OX-1R and OX-2R) are also expressed in neurons and endocrine cells in the gut[6,16,17]. Recent studies suggested that orexins, like 5-hydroxytryptamine (5-HT), vasoactive intestinal peptide (VIP) and several other gut hormones, could modulate gastrointestinal motility[5,7,18–20]. Given that the SO plays an important role in the digestive system, we hypothesized that orexins might play a role in modulating the motility of the SO.
In the present study, we investigated the effects of peripheral orexins on the SO in fasted rabbits by recording the myoelectric activity of the SO. In fasted rabbits, regular, single SPSO with frequencies of 0.17–0.88 Hz and amplitudes of 65–257 µV describes the basic rhythm of myoelectric activity of the SO. A dose-dependent excitatory effect of orexins on the frequency of SPSO was observed when either OXA or OXB was given at 4 different concentrations. Moreover, local administration of OXA or OXB also obviously increased the myoelectric activity of the SO.
Although we did not visualize changes in the pressure in the bile duct in the present study, the marked and continually increased myoelectric activity might indicate contractility of the sphincter[21]. This means that orexins appear to be potential stimulators of SO motility, resulting in a reduction in trans-sphincteric flow. Thus our findings have broadened the possible roles of the peripheral orexin system in the modulation of the digestive system. Nevertheless, the actual physiological role of the orexin system in the regulation of the SO needs to be further clarified.
Although orexins were administered peripherally in the present study, it is unclear whether orexins excite the SO in a peripheral or central manner. Morphological evidence indicates that orexin receptors and fibers are distributed in dosal vagal complex that plays a central role in controlling gastrointestinal function and comprises the dorsal motor nucleus of the vagus (DMN) and the nucleus of the solitary tract. Studies have suggested that orexins in the DMN have potent effects in promoting gastric motility[7,22,23]. Therefore, it is possible that orexins applied intravenously in the present study may stimulate the DMN after crossing the blood-brain barrier[24,25], which would subsequently increase the myoelectric activity of the SO. Furthermore, local administration of orexins (clearly not centrally) also evoked an increase in myoelectric activity. This implies that the peripheral pathway, in addition to the central pathway, may also be responsible for the effects of orexins on SO motility.
We next sought to elucidate the possible mechanisms underlying the effect of OXA on the myoelectric activity of SO. As we know, there is a vagal cholinergic excitatory pathway that, when active, has an important role in stimulating gastric function[26]. Thus we hypothesized that the increased motility of the SO caused by orexins might involve the enteric cholinergic neurons. To address this point, we injected the rabbits with 0.1 mg/kg atropine (a cholinergic muscarinic receptor antagonist) after intravenous injection of OXA or OXB. We found that atropine completely antagonized the orexin-increased myoelectric activity, indicating that the activation of the vagal cholinergic pathway is required for the stimulation effect of orexins on SO motor function. Our results are consistent with those of previous reports that have demonstrated that OXA can evoke the release of acetylcholine (ACh) from the enteric cholinergic neurons due to stimulation of the OX-1R, and then cause contractions of guinea pig ileum[27]. Additionally, we found in the present study that systemic administration of atropine alone blocked the myoelectric activity of the SO. Therefore, we can postulate that both basal electric activity and orexin-evoked electric activity of the SO may be mediated by cholinergic modulation. However, due to a lack of morphological data, our work cannot exclude the possibility that orexins directly regulate SO motility by binding to orexin receptors in SO tissue. For this reason, additional studies are needed to determine if orexins and orexin receptors are expressed in the SO area.
In summary, in general, peripheral OXA exerts excitatory effects on SO. It seems likely that the orexin system may play a significant role in modulating the function of SO, in part through activation of the cholinergic pathway.
Acknowledgement
We thank Hu-cheng LI, Hong-xiao FAN and Hong-mei XU for their technical assistance.
References
- Woods CM, Mawe GM, Toouli J, Saccone GT. The sphincter of Oddi: understanding its control and function. Neurogastroenterol Motil 2005;17 Suppl 1:31-40.
- Preti A. Orexins (hypocretins): their role in appetite and arousal. Curr Opin Investig Drugs 2002;3:1199-206.
- Sakurai T. Roles of orexins in the regulation of feeding and arousal. Sleep Med 2002;3 Suppl 2:S3-9.
- Yan J, Hu ZA. Involvement of orexin in the regulation of gastrointestinal function. Sheng Li Ke Xue Jin Zhan 2005;36:185-8. Chinese..
- Naslund E, Ehrstrom M, Ma J, Hellstrom PM, Kirchgessner AL. Localization and effects of orexin on fasting motility in the rat duodenum. Am J Physiol Gastrointest Liver Physiol 2002;282:G470-9.
- Kirchgessner AL, Liu M. Orexin synthesis and response in the gut. Neuron 1999;24:941-51.
- Krowicki ZK, Burmeister MA, Berthoud HR, Scullion RT, Fuchs K, Hornby PJ. Orexins in rat dorsal motor nucleus of the vagus potently stimulate gastric motor function. Am J Physiol Gastrointest Liver Physiol 2002;283:G465-72.
- Kobashi M, Furudono Y, Matsuo R, Yamamoto T. Central orexin facilitates gastric relaxation and contractility in rats. Neurosci Lett 2002;332:171-4.
- Kirchgessner AL. Orexins in the brain-gut axis. Endocr Rev 2002;23:1-15.
- Ehrstrom M, Naslund E, Ma J, Kirchgessner AL, Hellstrom PM. Physiological regulation and NO-dependent inhibition of migrating myoelectric complex in the rat small bowel by OXA. Am J Physiol Gastrointest Liver Physiol 2003;285:688-95.
- Miyasaka K, Masuda M, Kanai S, Sato N, Kurosawa M, Funakoshi A. Central orexin-A stimulates pancreatic exocrine secretion via the vagus. Pancreas 2002;25:400-4.
- Takahashi N, Okumura T, Yamada H, Kohgo Y. Stimulation of gastric acid secretion by centrally administered orexin-A in conscious rats. Biochem Biophys Res Commun 1999;254:623-7.
- Li HC, Dong JH, Hu ZA, Zhao HM, Xu HM. The effects of sandostatin on myoelectric activities of SO and its clinical significance. J Dig Surg 2004;3:54-7. Chinese..
- Zhao HM, Li HC, Hu ZA, Xu HM, Dong JH. Improvement of electrophysiological recording methods used for Oddi’s sphincter. Acta Academ Med Militaris Tertiae 2003;25:1772-3. Chinese..
- Funch-Jensen P. Sphincter of Oddi motility. Acta Chir Scand Suppl 1990;553:1-35.
- Voisin T, Rouet-Benzineb P, Reuter N, Laburthe M. Orexins and their receptors: structural aspects and role in peripheral tissues. Cell Mol Life Sci 2003;60:72-87.
- Nakabayashi M, Suzuki T, Takahashi K, Totsune K, Muramatsu Y, Kaneko C, et al. Orexin-A expression in human peripheral tissues. Mol Cell Endocrinol 2003;205:43-50.
- Behar J, Biancani P. Neural control of the sphincter of Oddi. A physiological role of 5-hydroxytryptamine in the regulation of basal sphincter of Oddi motor activity in the cat. J Clin Invest 1983;72:551-9.
- Hillsley K, Kircup AJ, Grundy D. Direct and indirect actions of 5-HT on the discharge of mesenteric afferent fibers innervating the rat jejunum. J Physiol 1998;506:551-61.
- Dahlstrand C, Dahlstrom A, Theodrsson E, Rehfeld J, Ahlman H. Is the CCK-8 induced relaxation of the feline sphincter of Oddi mediated by VIP neurons? J Auton Nerv Syst 1990;31:75-84.
- Chen JW, Schloithe AC, Toouli J, Saccone GT. Endothelin-1 stimulates sphincter of Oddi motility and decreases trans-sphincteric flow: a possible mechanism contributes to cholestasis in disease states. Neurogastroenterol Motil 2003;15:393-400.
- Harrison TA, Chen CT, Dun NJ, Chang JK. Hypothalamic orexin A-immunoreactive neurons project to the rat dorsal medulla. Neurosci Lett 1999;273:17-20.
- Ferguson AV, Samson WK. The orexin/hypocretin system: a critical regulator of neuroendocrine and autonomic function. Front Neuroendocrinol 2003;24:141-50.
- Zheng H, Patterson LM, Berthoud HR. Orexin-A projections to the caudal medulla and orexin-induced c-Fos expression, food intake, and autonomic function. J Comp Neurol 2005;485:127-42.
- Ouedraogo R, Naslund E, Kirchgessner AL. Glucose regulates the release of orexin-A from the endocrine pancreas. Diabetes 2003;52:111-7.
- Travagli RA, Hermann GE, Browning KN, Rogers RC. Musings on the wanderer: what’s new in our understanding of vago-vagal reflexes? III. Activity-dependent plasticity in vago-vagal reflexes controlling the stomach. Am J Physiol Gastrointest Liver Physiol 2003;284:G180-7.
- Matsuo K, Kaibara M, Uezono Y, Hayashi H, Taniyama K, Nakane Y. Involvement of cholinergic neurons in orexin-induced contraction of guinea pig ileum. Eur J Pharmacol 2002;452:105-9.