Inducible effects of icariin, icaritin, and desmethylicaritin on directional differentiation of embryonic stem cells into cardiomyocytes in vitro1
Introduction
Embryonic stem (ES) cells are pluripotent cells derived from the inner cell mass of the pre-implantation blastocyst[1]. These cells can proliferate in an undifferentiated state and remain totipotent when grown on a suitable fibroblast feeder layer. Under certain conditions ES cells are capable of differentiating into a variety of cell types in vitro, including spontaneously beating cardiac myocytes[2,3]. The entire differentiating process is rich in developmental-dependent biological information, for example, the sequence of expression of cardiac-specific genes and proteins during differentiation of ES cells in vitro accords with that in vivo[4]. Control of the ES cell mitotic cycle displays an unusual feature, and regulation of the cell cycle is critical in maintaining the transition towards differentiation[5,6]. The apoptotic signaling potential of ES cells is necessary to trigger ES cell differentiation[7,8]. Therefore, the system of ES cells can provide a platform for studying the effects of drugs on differentiation and detecting the specific or unique targets of drug action.
Up to now, the interfering effects of the traditional Chinese medicine on ES cells and their directional differentiation in vitro have not been investigated. Icariin (ICA), icaritin (ICT), and desmethylicaritin (DICT) (Figure 1) are constituents of Epimedium, a traditional Chinese herbal medicine that has many biological functions, particularly in cardiovascular function improvement, hormone regulation, immunological function modulation, and antitumor activity[9]. Our former work has shown that icariin can be metabolized to icartin and demethylicartin by human intestinal bacteria in vitro[10]. Furthermore, icaritin and desmethylicaritin, but not icariin, exert estrogen-like activity using the estrogen receptor-positive human breast adenocarcinoma MCF-7 cell proliferation assay[11]. In addition, icariin is the major active part in “Xin-shen-ning” tablets, which are used for the treatment of heart disease[12]. However, the pharmacological effects and mechanism of the icariin series on the cardiovascular system are not yet known.
In the present study, the inducible effects of icariin, icaritin, and desmethylicaritin on the directional differentiation of ES cells into cardiomyocytes were examined in vitro. The cardiomyocytes derived from ES cells were verified using immunocytochemistry. The possible mechanisms of inducible action in transcription level, cell cycle distribution and apoptosis were investigated using reverse transcription-polymerase chain reaction(RT-PCR) and cell cycle analysis, with a view to detecting the targets of drug action on promoting ES cells to differentiate in this culture system.
Materials and methods
Animals and cells NIH mice (male, weighing 24±2 g; female, weighing 22±2 g) were obtained from the Experimental Animal Center, Zhejiang University, Hangzhou, China (Grade II, Certificate N
Drugs and reagents Icariin was obtained from the Drug Biology Product Examination Bureau, Beijing, China (Batch N
Cultures of undifferentiated ES cells Fetuses were obtained from the mice on d 13 of gestation for the preparation of embryonic fibroblast (MEF) cells[13]. The MEF cells of generation three to generation five were used as feeder cells, which were treated with 1 mg/L mitomycin C for 1 h and plated at an appropriate density. ES-D3 cells were maintained in an undifferentiated state by culturing on a monolayer of MEF feeders in DMEM, supplemented with 10% FCS, 0.1 mmol/L β-mercaptoethanol, NEAA, and 1×106 U/L LIF.
Differentiation determination of cardiomyocytes and icariin, icaritin or desmethylicaritin treatment For differentiation of ES cells, EBs were generated using the hanging drop method[14,15]. Thirty microlitre of drops containing approximately 600 ES-D3 cells were placed on the lids of Petri dishes filled with D-Hanks solution, and cultivated in hanging drops for 3 d followed by another 2 d in the Petri dishes. On d 5, EBs were plated separately onto gelatin-coated 24-well culture plates in differentiation medium that consisted of DMEM, 20% FCS, 0.1 mmol/L β-mercaptoethanol, and NEAA. Icariin, icaritin, or desmethylicaritin were added to the differentiation medium at a concentration of 1×10-7 mol/L according to the preliminary test. Our previous study examining the concentration-effect relationship of icariin revealed that treatment with icariin 1×10-7 mol/L significantly enhanced cardiac differentiation (unpublished data). Thus, a concentration of 1×10-7 mol/L was used in the present experiment. ES-D3 cells treated with RA 1×10-8 mol/L and with 0.1% Me2SO were used as positive and negative controls, respectively.
In the experiment, d 1 referred to the day of dissociation of ES cells from MEF and the initiation of differentiation by the formation of EBs. EBs were observed with light microscopy every day to record the morphology and the number of the spontaneously beating EBs from d 7 to d 7+23. According to the percentage of the beating EBs, the inducing effects of icariin, icaritin, or desmethylicaritin on the directional differentiation of ES-D3 cells into cardiomyocytes were evaluated using the time-effect relationship curve. Rhythmically beating EBs were considered to be spontaneously beating cardiomyocytes in EB outgrowths, and were defined as the marker of successful differentiation[14,15].
Expression of cardiac-specific proteins analyzed using immunocytochemistry Cardiomyocytes were isolated from the beating areas of EBs using a modified procedure described by Maltsev et al[16,17]. For immunostaining, cells were rinsed twice with PBS, and fixed with cold acetone for 10 min. After being treated with goat serum for 30 min, specimens were incubated at 4 ºC overnight with the primary antibody, monoclonal anti-sarcomeric-actinin (clone number EA-53, 1:200 dilution, Sigma) or monoclonal anti-troponin T (clone number JLT-12, 1:100 dilution, Sigma). The following day, the specimens were washed with PBS three times and incubated with the fluorescent antibody: FITC-conjugated F(ab)2 fragment of affinity-purified goat anti-mouse IgG (1:1000 dilution, Rockland Inc Gilbertsville, PA, USA) for 1.5 h at 37 ºC. Specimens were then rinsed in PBS, mounted onto a coverslip with 90% glycerol in PBS and examined using a fluorescence microscope (Leica DMIL,California Nevada, Germany).
Expression of cardiac-specific genes using semi-quantitative RT-PCR The expression of the cardiac-specific α-myosin heavy chain (α-MHC) gene and myosin light chain-2v (MLC-2v) in EBs was confirmed using semi-quantitative RT-PCR. Total RNA was isolated from EBs (n=20) induced by 1×10-7 mol/L icariin, icaritin, or desmethylicaritin, and 1×10-8 mol/L RA or solvent, respectively, at d 7+0, d 7+5, d 7+9 using the Trizol reagent according to the manufacturer’s instructions. After extraction, mRNA was precipitated using the recommended procedures and dissolved in 0.1% diethylpyrocarbonate solution. To synthesize first strand cDNA, 7 µL total RNA was incubated in 0.5 µg of oligo (dT) 6 primer and 5 µL deionized water at 65 ºC for 15 min. Reverse transcription reactions were carried out with 200 U of M-MuLV reverse transcriptase in 5×reaction buffer and 1 mmol/L dNTP mixture for 1 h at 42 ºC. Multiplex polymerase chain reactions of 50 µL contained 1 µL of the RT reaction product, 10×PCR buffer, 25 U Taq polymerase, 1 µL of 10 mmol/L dNTP mixtures, and 30 pmol of each primer. The specific primer pairs were designed as follows: the cardiac-specific α-MHC gene (5'-CTGCTGGAGAGGTTATTCCTCG-3',5'-GGAAGAGTGAGCGGCGCATCAAGG-3'; 301 bp)[18] and MLC-2v (5'-TGTGGGTCACCTGAGGCTGTGGTTCAG-3',5'-GAAGGCTGACTATGTCCGGGAGATGC-3'; 189 bp)[18], and the housekeeping gene β-actin was used as an internal standard (5'-TGACGGGGTCACCCACACTGTGCCCATCTA-3',5'-CTAGAAGCATTTGCGGTGGACGATGGAGGG-3'; 660 bp).
For the semi-quantitative determination of α-MHC and MLC-2v mRNA levels, the products of the reverse transcription reactions were denatured for 3 min at 94 ºC, followed by 40 cycles (α-MHC), 45 cycles (MLC-2v), and 30 cycles (β-actin) of amplification in the reaction with Ampli Taq DNA polymerase: 45 s denaturation at 94 ºC, 40 s annealing at 66.4 ºC (α-MHC) or 61.1 ºC (MLC-2v) or 55 ºC (β-actin) and 45 s elongation at 72 ºC. The PCR products were analyzed using 1.5% agarose gel electrophoresis, visualized with ethidium bromide staining, and quantified using a bio-imaging analyzer (Bio-Rad, Hercules, CA, USA), and the density of the products was quantified using Quantity One version 4.2.2 software (Bio-Rad).
Analysis of cell cycle and apoptosis by flow cytometry To analyze the cell cycle distribution of ES cells and apoptosis as parameters in the early differentiation phase, ES-D3 cells were cultivated without MEF feeders and LIF, and treated with 1×10-7 mol/L icariin, icaritin, or desmethyl-icaritin, or 1×10-8 mol/L RA. After 48 h, the cells were harvested and 1×106 cells were placed into a polypropylene tube and centrifuged at 90×g. The supernatant was removed and 70% EtOH 1 mL in 4 ºC was dropped into the cell pellet while vortexing. The cells were kept at 4 ºC until the DNA was stained. Fixed cells were treated with RNase A in PBS for 1 h, followed by staining with 50 mg/L propidium iodide in PBS. Flow cytometric analysis of the cell cycle distribution and apoptosis was carried out using a BD FACSCalibur with a 488 nm (blue) argon (Becton Dickinson, San Jose, CA, USA). Data acquisition was carried out with CellQuest 3.1 software and the data were analyzed with ModFit LT 3.0 software (Variety Software House, Topsham, ME, USA).
Statistical analysis Each data point represents the mean±SD. At least three independent experiments were carried out. Statistical significance was evaluated using one-way ANOVAS with SPSS 10.0 for WINDOWS software. P<0.05 was considered statistically significant.
Results
Apparent cell morphological changes during the course of differentiation ES-D3 cells grew aggregates with clear boundaries and appeared ovoid or nodule shaped on MEF feeder cells (Figure 2A). Representative EBs at 5 d after the initiation of differentiation, and just prior to plating onto gelatin-coated 24-well culture plates, were formed by hanging drop cultures. EBs kept globular shaped structure. (Figure 2B). After plating for 2 d, cells started outgrowing from the EBs, and cardiomyocytes appeared as spontaneously contracting cell clusters[14] (Figure 2C). One EB contained one or more beating areas in which the enlarged size, increased contracting strength, and beating frequency were observed during the subsequent differentiation phase.
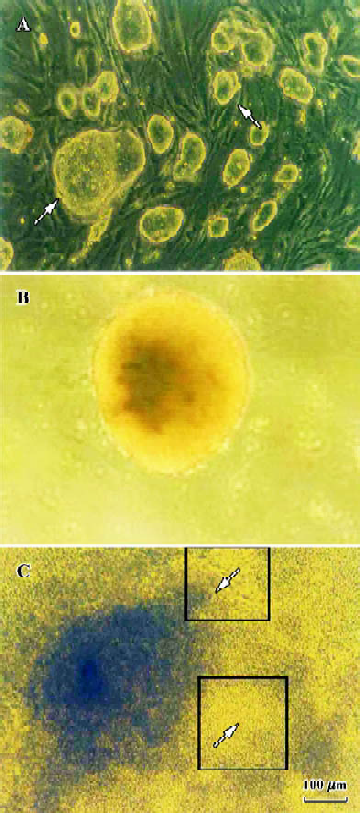
Inducible effect of icariin, icaritin, and desmethylicaritin on the directional differentiation of ES-D3 cells into cardiomyocytes Icariin, icaritin, and desmethylicaritin influenced the degree of cardiac differentiation of ES-D3 cells. During the course of differentiation (from d 7+2 to d 7+23), the total percentage of beating EBs treated with 1×10-7 mol/L icariin, icaritin, and desmethylicaritin was 87% (P<0.01), 59% (P< 0.01), and 49%, respectively. In particular, in the case of icariin at a concentration of 1×10-7 mol/L the inducing effect on the directional differentiation of ES-D3 cells into cardio-myocytes was remarkable compared with the control (P<0.05). Although only 46% of the EBs in control samples contained beating clusters, 68% of the EBs treated with 1×10-8 mol/L RA differentiated into beating cardiac clusters (Figure 3A).
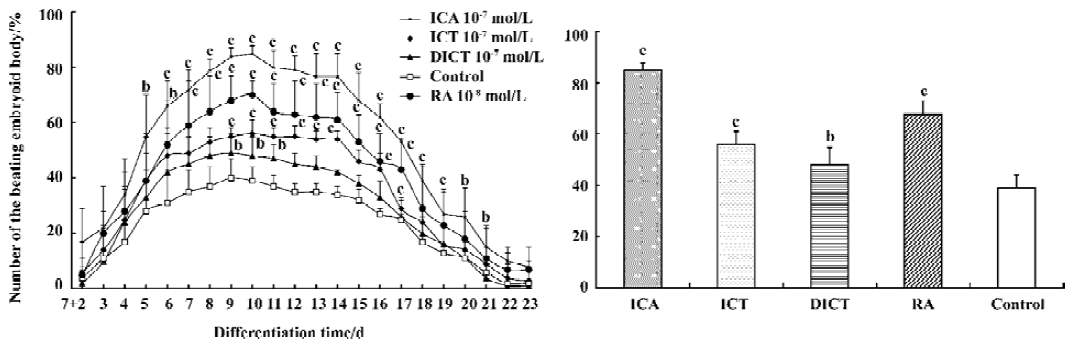
The results of the time-effect relationship revealed that the percentage of differentiation cultures containing contracting EBs with icariin 1×10-7 mol/L reached a peak level of 85% at d 7+10 and 10% at terminal stages. The potential of EBs treated with icariin 1×10-7 mol/L undergoing cardiac differentiation was enhanced compared with control cells over the period from d 7+5 to d 7+23 (P<0.05). Treatment of EBs with icaritin 1×10-7 mol/L also resulted in a remarkable increase in the number of EBs with spontaneously beating cardiomyocytes between d 7+9 and d 7+17 (P<0.05), whereas the differentiation effect of EBs induced by desmethylicaritin 1×10-7 mol/L was only prominent from d 7+9 to d 7+11 (P<0.05) (Figure 3B).
If culture of differentiation was continued, the numbers of spontaneously beating foci increased and the spontaneously contractile activity of cardiac myocytes strengthened[19].
Detection of cardiac-specific proteins during the differentiation phases of ES-D3 cells into cardiomyocytes To identify whether the observed cell types derived from ES-D3 cells expressed cardiac-specific proteins, indirect immunofluorescence was carried out. The results verified that differentiated beating cardiac cells stained positively with anti-α-actinin mAb and anti-troponin T mAb, and these results support previous studies[20] (Figure 4).
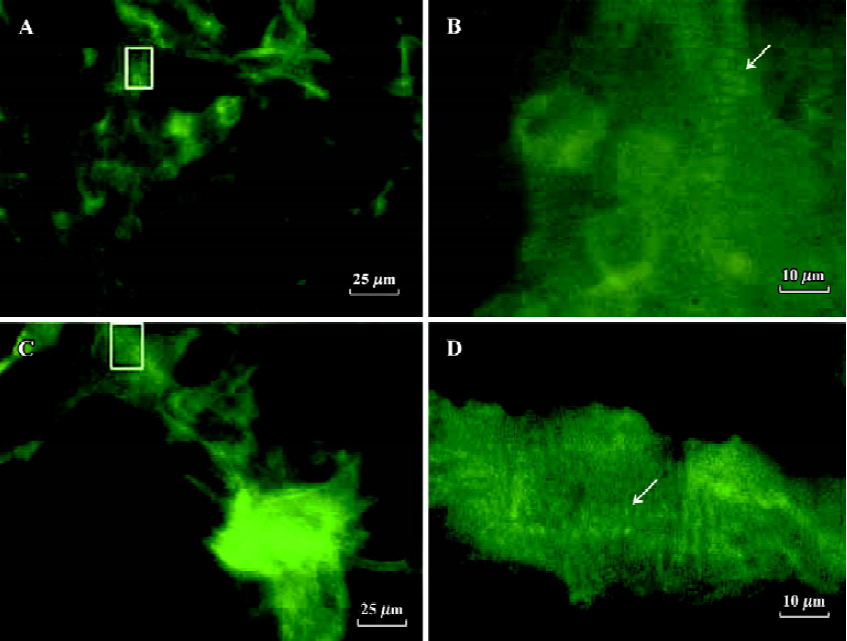
Effect of icariin, icaritin, and desmethylicaritin on the level of α-cardiac MHC and MLC-2v mRNA during the early cardiac developmental stage To explore whether icariin, icaritin, and desmethylicaritin influence the expression level of a-cardiac MHC and MLC-2v mRNA during cardiomyocyte differentiation, the beating EBs outgrowths differentiated from ES-D3 cells were studied using semi-quantitative RT-PCR analysis. mRNA expression levels of α-MHC by 1×10-7 mol/L icariin, icaritin, and of MLC-2v by 1×10-7 mol/L icariin increased during an early cardiac developmental stage for the period between d 7+0 and d 7+9. In particular, 1×10-7 mol/L icariin-induced MLC-2v expression was detected as early as d 7+0, whereas increased expression in control cells was not observed until d 7+5. The experiments also revealed that there was almost no difference between 1×10-7 mol/L desmethylicaritin treated cells and control cells from d 7+0 to d 7+9 (Figure 5).
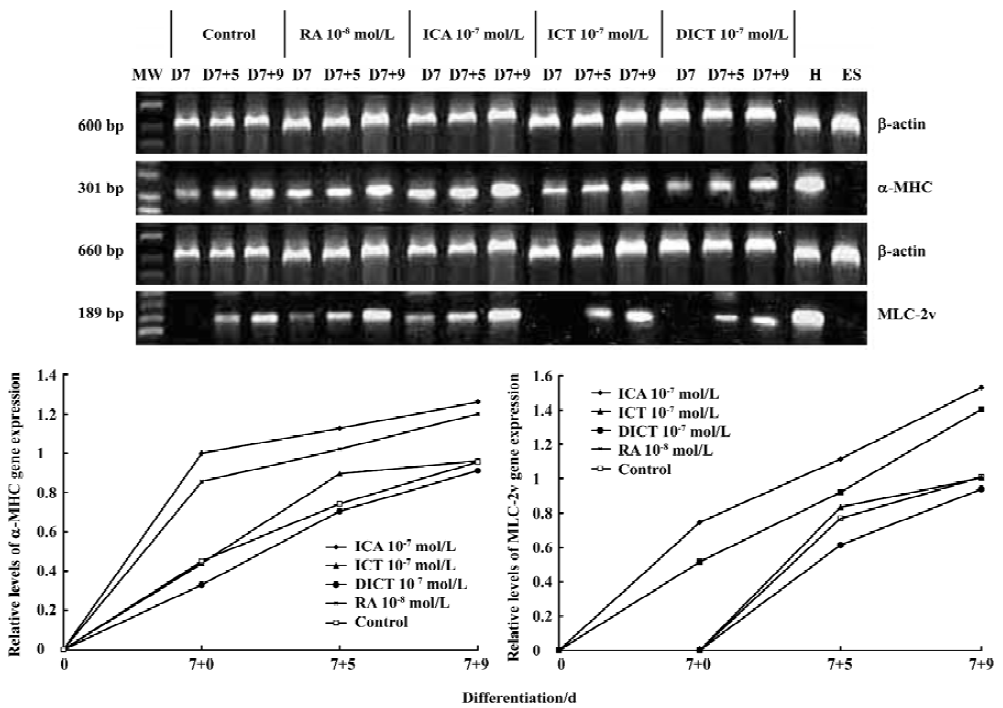
Analysis of cell cycles and apoptosis using flow cytometry The cell cycle distribution of ES-D3 cells was considered to be a parameter of their differentiation state[5,6], which enabled us to determine the effect of icariin, icaritin, and desmethylicaritin on early differentiation events before a shift to the cardiomyocyte phenotype. The results of the cell cycle analysis on propidium-iodide-stained cells showed a smaller percentage of ES-D3 cells in the G0/G1 phase compared with differentiation cells in control samples (approxi-mately 30% and 40%, respectively). Icariin, icaritin, and desmethylicaritin 1×10-7 mol/L induced the accumulation of cells in G0/G1 (approximately 44%, 40%, and 35%, respec-tively). In particular, the effect of icariin on the G0/G1 was remarkable compared with control cells (P<0.05). Treatment of 1×10-8 mol/L RA let ES cells present a similar effect on the accumulation of cells in G1 (48%). Proportions of S phase cells in either 1×10-7 mol/L icariin-treated cells or 1×10-8 mol/L RA-treated cells were remarkably reduced (29% and 27%, respectively, versus 47% of S phase in ES-D3 cells; P<0.05) (Figure 6).
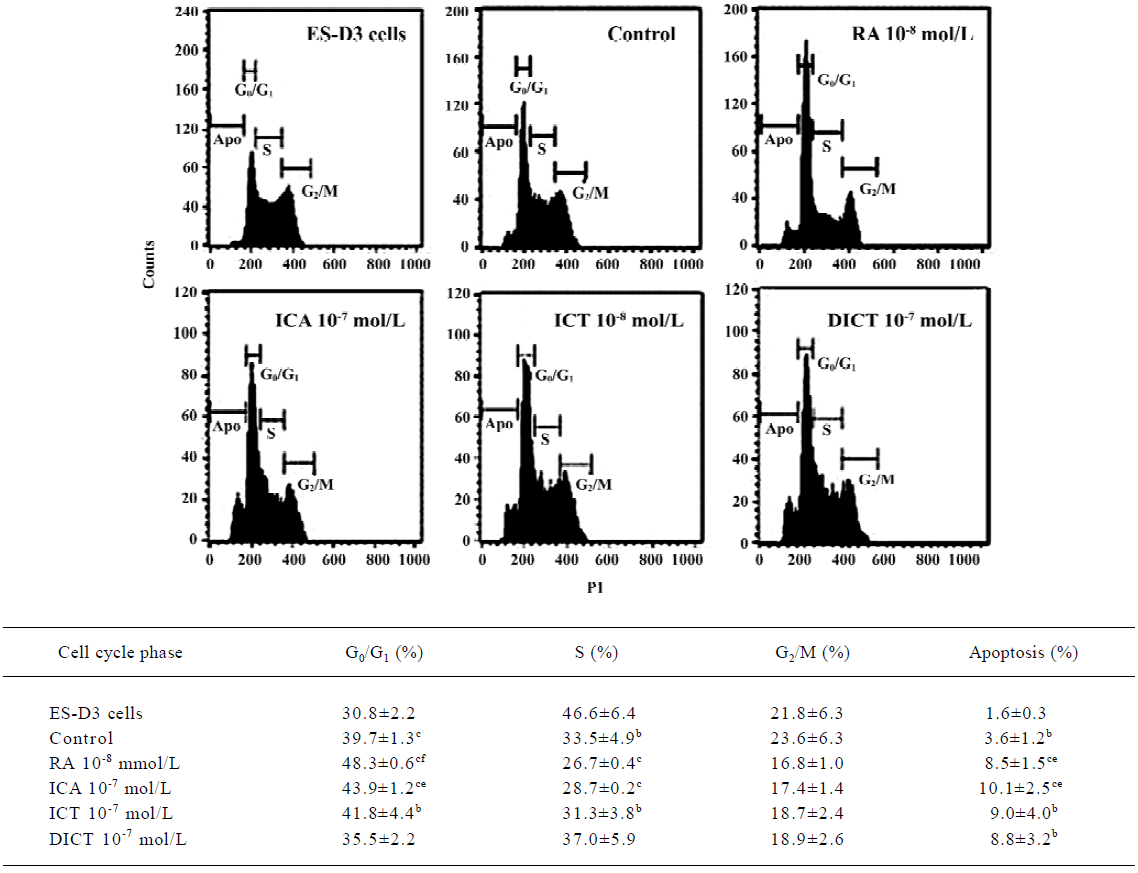
Apoptosis plays a vital role in development by removing unwanted cells[21], which is a possible checkpoint for the transition towards differentiation of ES cells. In the present study, ES-D3 cells induced by icariin 1×10-7 mol/L for 48 h showed greater levels of apoptosis (10%) than control cells (4%) (P<0.05). In contrast, treatment with icaritin 1×10-7 mol/L or desmethylicaritin resulted in apoptosis levels of approximately 9% and 8%, respectively. RA 1×10-8 mol/L treatment for 48 h also led to 8% apoptosis (P<0.05; Figure 6).
Discussion
ES cell differentiation into cardiomyocytes in vitro is a unique system that not only provides opportunities to study cardiomyocyte differentiation, but also offers a platform for evaluating the effects of drugs and detecting the specific targets of drug action during cardiogenesis. In the present study, the possible inducible effects of icariin, icaritin, and desmethylicaritin on the directional differentiation of ES cells into cardiomyocytes in vitro were explored. To investigate the partly inducible effect mechanisms of the three compounds involved in ES cell differentiation, the expression of cardiac developmental-dependent genes was detected using RT-PCR. Cell cycle distribution and apoptosis were analyzed using flow cytometry.
Our results revealed that treatment of EBs with icariin resulted in increased and accelerated differentiation into beating cardiomyocytes. The degree of the inducible effect of icaritin was less than that of icariin, and desmethylicaritin had the least inducing effect on the directional differentiation of ES cells into cardiomyocytes in vitro. Moreover, cardiac-specific sarcomeric proteins in the cardiomyocytes derived from ES-D3 cells were identified, since one of the major questions in muscle development is how a large number of protein subunits assembles into the remarkably regular structure known as the sarcomere[20]. Our results confirmed that the cardiomyocytes were derived from ES-D3 cells.
ES-cell-derived cardiomyocytes express the cardiac genes in a developmentally controlled manner, that is, atrial natriuretic factor (ANF), α-MHC, β-MHC, and MLC-2v[4]. In our experiment, α-MHC and MLC-2v were chosen as the evaluation targets for the directional differentiation. Our experiments demonstrated that icariin significantly advanced and increased the mRNA levels of α-MHC and MLC-2v at an early stage of differentiation, which suggested a partial mechanism for icariin involving in differentiation could be related to the expression of the cardiac developmental-dependent genes[4,22,23]. However, icaritin and desmethylicaritin had less effect on improving the mRNA level of α-MHC and MLC-2v during cardiac differentiation. Other studies have shown that α-MHC is expressed relatively early during the course of cardiogenesis[24]; however, MLC-2v mRNA is highly expressed relatively late in the process and is spatially restricted to the ventricular portion[25,26]. Our results support these studies. One of the mechanisms of icariin in differentiation is to increase and accelerate the mRNA level of α-MHC and MLC-2v at an early stage of differentiation.
Icariin is a new type of biological response modifier and differentiation agent[27] and can induce some cells to differentiate by regulating the cell cycle[27,28]. The cell cycle distribu-tion of ES cells was considered to be a parameter of their differentiation state[5,6]. This system of ES cell differentiation could recapitulate the in vivo differentiation process, including the occurrence of apoptosis accompanying differentiation. Furthermore, the potential in ES cell apoptotic signals is necessary to trigger ES cell differentiation. Alterna-tively, it is possible that some cells enter apoptosis and the remainder differentiate, and this may depend on their stage in the cell cycle[7,8]. To elucidate the mechanism of icariin action on differentiation before a shift to the cardiomyocyte phenotype, we examined the distribution of the ES cell cycle and apoptosis using flow cytometry. Our experiment showed that the induction of icariin was associated with remarkable enrichment of ES-D3 cells in the G0/G1 phase and a significant reduction in cells in the S phase. In addition, icariin-mediated differentiation leads to higher apoptosis. These results suggest that the effect of icariin is associated with cell cycle arrest and apoptosis. The balance between positive and negative regulators of the ES cell cycle is important in maintaining the transition towards differentiation. The apoptotic signal in ES cells also triggers the differentiation of ES cells[7].
In conclusion, ES-D3 cells could be remarkably induced to directionally differentiate into cardiomyocytes by icariin at a concentration of 1×10-7 mol/L. The promoting effect of icariin on cardiac differentiation depended on the advancing and increasing gene expression of α-cardiac MHC and MLC-2v. At an early differentiation stage, the inducible effect of icariin was related to the regulation of the cell cycle and the induction of apoptosis.
References
- Evans MJ, Kaufman MH. Establishment in culture of pluripo-tential cells from mouse embryos. Nature 1981;292:154-6.
- Doestschman TC, Eistetter H, Katz M, Schmidt W, Kemler R. The in vitro development of blastocyst-derived embryonic stem cell lines: formation of visceral yolk sac, blood islands and myocardium. J Embryol Exp Morph 1985;87:27-45.
- Robbins J, Doetschman T, Jones WK, Sanchez A. Embryonic stem cells as a model for cardiogenesis. Trends Cardiovasc Med 1992;2:44-50.
- Hescheler J, Fleischmann BK, Lentini S, Maltsev VA, Rohwedel J, Wobus AM, et al. Embryonic stem cells: a model to study structural and functional properties in cardiomyogenesis. Cardiovasc Res 1997;136:149-62.
- Rohwedel J, Sehlmeyer U, Shan J, Meister A, Wobus AM. Primordial germ cell-derived mouse embryonic germ (EG) cells in vitro resemble undifferentiated stem cells with respect to differentiation capacity and cell cycle distribution. Cell Biol Int 1996;20:579-87.
- Ludmila J, Marielle A, Stephanie GG, Suzy M, Pierre S. Differential contributions of ERK and PI3-kinase to the regulation of cyclin D1 expression and to the control of the G1/S transition in mouse embryonic stem cells. Oncogene 2002;21:5515-28.
- Kanaga S, Martina K, Rudolf J, Erwin FW. Regulation of ES cell differentiation by functional and conformational modulation of p53. EMBO J 1997;16:6217-29.
- Sarkar SA, Sharma RP. All-tras-retinoic acid-mediated modulation of p53 during neural differentiation in murine embryonic stem cells. Cell Biol Toxicol 2002;18:243-57.
- He W, Sun H, Yang B, Zhang D, Kabelitz D. Immunoregulatory effects of the herb Epimediia glycoside icariin. Arzneimittel-forschung 1995;45:910-3.
- Liu J, Lou YJ. Determination of icariin and metabolites in rat serum by capillary zone eletrophoresis: rat pharmacokinetic studies after administration of icariin. J Pharmaceut Biomed 2004;29:365-70.
- Wang ZQ, Lou YJ. Proliferation-stimulating effects of icaritin and desmethylicaritin in MCF-7 cells. Eur J Pharmacol 2004;504:147-53.
- Zhang YW, Morita I, Shao G, Yao XS, Murota SI. Screening of anti-hypoxia/reoxygenation agents by an in vitro model. Part 1: Natural inhibitors for protein tyrosine kinase activated by hypoxia/reoxygenation in cultured human umbilical vein endothelial cells. Planta Med 2000;66:114-8.
- Qiu GT, Huang B, Tang SB, Hu J, Liu ZX. The investigation of cell culture conditions maintained embryonic stem cells as totipotent cells. Acad J SUMS 2000;21:100-3.
- Metzger JM, Lin WI, Johnston RA, Westfall MV, Samuelson LC. Myosin heavy chain expression in contracting myocytes isolated during embryonic stem cell cardiogenesis. Circ Res 1995;76:710-9.
- Scholz G, Pohl I, Genschow E, Klemm M, Spielmann H. Embryotoxicity screening using embryonic cells in vitro: correlation to in vivo teratogenicity. Cells Tissues Organs 1999;165:203-11.
- Maltsev VA, Rohwedel J, Hescheler J, Wobus AM. Embryonic stem cells differentiation in vitro into cardiomyocytes representing sinusnodal, atrial and ventricular cell type. Mech Dev 1993;44:41-50.
- Maltsev VA, Wobus AM, Rohwedel J, Bader M, Hescheler J. Cardiomyocytes differentiated in vitro from embryonic stem cells developmentally express cardiac-specific genes and ionic currents. Circ Res 1994;75:233-44.
- Wobus AM, Kaomei G, Shan J, Wellner M, Rohwedel J, Guanju J, et al. Retinoic acid accelerates embryonic stem cell-derived cardiac differentiation and enhances development of ventricular cardiomyocytes. J Mol Cell Cardiol 1997;29:1525-39.
- Boheler KR, Czyz J, Tweedie D, Yang HT, Anisimov SV, Wobus AM. Differentiation of pluripotent embryonic stem cells into cardiomyocytes. Circ Res 2002;91:189-201.
- Guan K, Furst DO, Wobus AM. Modulation of sarcomere organization during embryonic stem cell-derived cardiomyocyte differentiation. Eur J Cell Biol 1999;78:813-23.
- Uren AG, Vaux DL. Molecular and clinical aspects of apoptosis. Pharmacol Ther 1996;72:37-50.
- Wobus AM, Guan K. Embryonic stem cell-derived cardiac differentiation: modulation of differentiation and ‘loss of function’ analysis in vitro. Trends Cardiovasc Med 1998;8:64-74.
- Miller-Hance WC, LaCorbiere M, Fuller SJ, Evans SM, Lyons G, Schmidt C, et al. In vitro chamber specification during embryonic stem cell cardiogenesis. Expression of the ventricular myosin light chain-2 gene is independent of heart tube formation. J Biol Chem 1993;268:25244-52.
- Lyons GE, Schiaffino S, Sassoon D, Barton P, Buckingham M. Developmental regulation of myosin gene expression in mouse cardiac muscle. J Cell Biol 1990;111:2427-36.
- Franz W-M, Breves D, Klingel K, Brem G. Heart-specific targeting of firefly luciferase by the myosin light chain-2 promoter and developmental regulation in transgenic mice. Circ Res 1993;73:629-38.
- O’Bruen TX, Kee K, Chien KR. A morphogenetic field of MLC-2v gene expression in the primordial heart tube of normal and MLC-luciferase transgenic mice. Proc Natl Acad Sci USA 1993;90:5157-61.
- Mao H, Zhang L, Wang Y, Li X. Experimental studies of icariin on anticancer mechanism. J Chin Med Mater 2000;23:554-6.
- Di KJ, Zhang JB. An outline of icariin pharmacological study. J Nature 2003;25:191-6. Chinese..