Emerging roles of deubiquitinating enzymes in human cancer1
Introduction
The post-translational modification of proteins by the covalent linkage of ubiquitin targets those proteins for degradation by the proteasome, and this process involves the participation of both ubiquitinating enzymes and deubiqui-tinating enzymes. Although deubiquitinating enzymes constitute a large family in the ubiquitin–proteasome system, their biological functions and pathophysiological roles remain largely unknown and have only just begun to be understood. In recent years, more and more studies have revealed the involvement of deubiquitinating enzymes in cancers as well as in other diseases. In this review, we will mainly focus on the emerging roles of this class of enzymes in the development and progression of cancers.
Molecular functions of deubiquitinating enzymes
Ubiquitin-mediated protein degradation and lysosomal protein degradation are the 2 mechanisms for intracellular protein turnover. The ubiquitin-mediated mechanism is further classified into the ubiquitin/proteasome pathway and ubiquitin/aggresome pathway. The ubiquitin/proteasome system is the major non-lysosomal proteolytic mechanism in the cytosol and nucleus of eukaryotic cells, lysosomal, and the ubiquitin/aggresome pathway. An important function of this mechanism is the degradation of abnormal proteins generated under normal and stress conditions. The substrates of this system include many cystolic, nucleic, and integral membrane proteins, such as transcription factors, cell cycle regulators, and signal transducers[1]. The degradation of a protein via the ubiquitin–proteasome pathway involves 2 successive steps: (1) the conjugation of multiple ubiquitin moieties to the substrate; and (2) the degradation of the tagged protein by the 26S proteasome complex.
The enzymatic reactions of the ubiquitin pathway have mostly been elucidated. In these reactions, proteins are targeted for degradation by covalent ligation to ubiquitin, a 76 amino acid polypeptide that is universally distributed among eukaryotes and highly conserved. The ubiquitin–protein conjugation requires the sequential execution of 3 types of enzymes (Figure 1). The C-terminal glycine residue of ubiquitin is activated in an ATP-dependent process by an ubiquitin-activating enzyme, E1. Activated ubiquitin is then transmitted to a cysteine residue of an ubiquitin-conjugating enzyme (ubiquitin carrier protein), E2. In the last step catalyzed by an ubiquitin-protein ligase (E3), ubiquitin is linked by its C-terminus in an amide isopeptide linkage to a e-amino group of the lysine residues of the substrate protein. This conjugation cascade is highly substrate specific.
The modification of proteins by ubiquitin is reversible. Ubiquitinated proteins are not only subject to degradation, but also to deubiquitination. When tagged proteins are subjected to degradation by the 26S proteasome complex, it requires the release of free and reusable ubiquitin. The deubi-quitination reactions, which require accurate proteolytic processing at the C-terminal glycine of ubiquitin, are catalyzed by deubiquitinating enzymes (Figure 2). The substrates for these enzymes include precursor forms of ubiquitin, conjugates resulting from non-specific side reactions of E1-S-ubiquitin/E2-S-ubiquitin with cellular nucleophiles, coval-ently-linked ubiquitin substrates, and poly-ubiquitin chains. Deubiquitinating enzymes are isopeptidases and consist of 2 classes: ubiquitin C-terminal hydrolases and ubiquitin-specific processing proteases. Ubiquitin C-terminal hydrolases are papain-like thiol proteases with a 230 amino acid core catalytic domain and share significant sequence similarity to the neuron-specific human protein PGP9.5 (UCH-L1). These enzymes cleave ubiquitin derivatives with small or disordered C-terminal domains[2,3]. Most of this class of enzymes is relatively small with a molecular weight less than 40 kDa. Ubiquitin C-terminal hydrolases are known to have important roles in development and neural function. For example, during the establishment of long-term synaptic facilitation in Aplysia, the induction of a protein with UCH enzyme activity was observed[4]. Ubiquitin-specific processing proteases are a much larger group of thiol proteases and are very divergent with a molecular weight ranging from 50 to 250 kDa. This class of enzymes is known to be involved in the regulation of signal transduction, growth, and development[3]. Both deubiquitinating enzyme classes have a specific, tight binding site for ubiquitin. Although the exact and specific physiological roles of deubiquitinating enzymes have not been fully established, the following functions are generally accepted: (1) processing of ubiquitin precursors; (2) recycling of adventitiously trapped catalytic intermediates; (3) proofreading of protein ubiquitination; (4) recycling of ubiquitin from polyubiquitinated proteins following commitment to degradation; (5) keeping proteasomes free of ubiquitin chains; and (6) maintaining free ubiquitin concentrations[1]. The functions and molecular basis of deubiquitination and deubiquitinating enzymes are increasingly being understood over the past several years. For example, it is now known that deubiquitination of protein substrates is tightly coupled with degradation in the proteasome[5,6], and the inactivation of the deubiquitinating enzyme can completely prevent protein degradation[5]. However, due to the large number of members in the deubiquitinating enzyme family, it is not surprising to see that different members execute totally different functions. It was found that UBP6, a proteasome-associated cysteine protease, delays protein degradation[7]. More interestingly, a recent study demonstrated that ubiquitin C-terminal hydrolase-L1 possesses ligase activity as well as hydrolase activity, and these 2 opposing enzymatic activities affect the degradation of a-synuclein and susceptibility to Parkinson’s disease[8].
Aberrant expression of deubiquitinating enzymes in human cancers
Several types of deubiquitinating enzymes were found to be upregulated in cancer cells. Ubiquitin C-terminal hydrolase-L1, identical to the neuron-specific protein gene product PGP9.5 and widely expressed in neuronal tissues (1%–2% of brain proteins) at all stages of neuronal differentiation was originally suggested as a neuroendocrine marker[9]. Two decades ago there were reports that tumors of neuroendocrine origin, such as small cell carcinoma, showed positive staining for PGP9.5[10,11]. Recently, Jen and colleagues examined the expression of PGP9.5 in normal lung epithelium, lung tumor cell lines, and 98 resected primary non-small-cell lung carcinomas and observed that the increased expression of PGP9.5 was specifically associated with cancer development[12]. In primary non-small-cell lung carcinomas, 54% (53/98) of the cases had positive PGP9.5 staining, and this protein was present in 44% (29/66) of stage I and 75% (24/32) of stages II and IIIA non-small-cell lung carcinomas[12]. The high expression of PGP9.5 also occurs in other types of cancer. The evaluation of the expression of PGP9.5 in 69 resected ductal carcinomas of the pancreas and in normal pancreatic tissues revealed a significant negative correlation between the overexpression of PGP9.5 and postoperative survival[13]. PGP9.5-negative pancreatic cancer patients had significantly better survival rates compared with those who were PGP9.5 positive[13]. In colorectal cancer, the same group also examined the expression of PGP9.5 in primary colorectal cancers and correlated the results with the pathological features[14]. In colorectal cancer, 46% (33/74) of the specimens showed positive staining with PGP9.5 in most tumor cells, whereas no PGP9.5 expression was detected in adjacent normal epithelium. A correlation analysis revealed 2 significant differences in maximal tumor size and the extent of the tumor[14]. More recently, the overexpression of ubiquitin C-terminal hydrolase-L1 was reported in human myeloma and medullary thyroid carcinoma cells[15]. These studies indicate that the expression of PGP9.5 is closely associated with cancer progression, and suggest that this protein could be a useful a tumor marker for diagnosis and prognosis. In our studies on the multidrug resistance phenotype, we found that ubiquitin C-terminal hydrolase-L1 is overexpressed in several drug-resistant cancer cell lines. However, the exact role of ubiquitin C-terminal hydrolase-L1 (PGP9.5) and its regulation in cancer remains unknown. Al-Katib et al demonstrated that ubiquitin C-terminal hydrolase-L1 expression could be induced by the phorbol ester, 12-O-tetradecanoylphorbol-13-acetate, and by bryostatin, in acute lymphoblastic leukemia and suggested a role of this enzyme in B-cell differentiation[16,17]. PGP9.5 was shown to interact and colocalize with JAB1, a Jun activation domain-binding protein that can bind to p27kip1[18]. This interaction and colocalization was suggested to contribute to the degradation of p27kip1[18], a cyclin-dependent kinase inhibitor whose loss in epithelial cancers was significantly correlated with the pathological tumor grade and with high-grade, poorly-differentiated tumors showing a significantly lower p27 protein than their well-differentiated counterparts[19]. The decreased level of p27kip1 is frequently observed in human cancers, including breast, lung, prostate, colon, skin, and ovarian cancers[20–22], and has been found to correlate with cancer development and poor survival[19]. These observations also provide a clue to the implication of UCH-L1 in cancer development and progression. In addition, deubiquitinating enzymes are upregulated in Burkitt’s lymphoma, a highly malignant B cell neoplasma, and some other types of malignancies[23,24].
Deubiquitinating enzymes: oncogenes or tumor suppressors?
Alterations in the affluence of important regulatory proteins are the frequently observed defects in malignant cells. The gain in the function of oncogenes or loss of tumor suppressor proteins is the umbilical mechanism by which cells escape normal control on proliferation. Because of the broad involvement of the ubiquitin–proteasome proteolysis in the regulation of protein turnover, this pathway may play a vital role in cancer development and progression. In fact, considerable insight into the linkage of the ubiquitin–proteasome pathway with cancer has been gained in recent years. For example, the tumor suppressor p53 is tightly regulated by the ubiquitin–proteasome pathway[25,26]. p53 is an unstable nuclear protein with a half-life of 30 min in normal cells. After cellular stress or DNA damage, p53 is stabilized through the downregulation of its degradation, leading to growth arrest or apoptosis. The ubiquitination-proteasome pathway has also been reported to play a critical role in the pathogenesis of breast cancer by affecting the downregulation of growth factor receptors, such as EGFR/ErbB-1, Neu/ErbB-2, and ErbB-3/HER3[ (27, 28)]. Nuclear factor-kappa B (NF-κB) plays a pivotal role in many aspects of tumor development, progression, and therapy, and its activation relies primarily on the ubiqui-tination-mediated degradation of its inhibitor IκB[29]. The role the deubiquitinating enzymes play in the regulation of those oncogenes or tumor suppressor genes represents an emerging and interesting research area, and novel functions of a variety of deubiquitinating enzymes have begun to be uncovered. Gu et al discovered that herpesvirus-associated ubiquitin-specific protease [HAUSP, or ubiquitin-specific protease (USP)7] possesses ubiquitin hydrolase activity that can stabilize p53 by deubiquitinating the tumor suppressor, suggesting a tumor suppressor function of this deubiqui-tinating enzyme[30]. In contrast, using the genetic disruption approach, Cummins and Vogelstein demonstrated that MDM2, rather than p53, is the HAUSP substrate, and the deubiquitination of MDM2 by HAUSP leads to the destabilization of p53[31]. Gu et al later also found that HAUSP can deubiquitinate and stabilize MDM2 in a p53-independet manner, suggesting that HAUSP plays a dynamic role in the p53–MDM2 pathway[32]. Therefore, HAUSP may have both tumor suppressive and oncogenic effects, depending on different circumstances.
USP2a is a deubiquitinating enzyme that is overexpressed in prostate cancer and plays an important role in regulating tumor cell survival. Recently, it was found that this isopeptidase can deubiquitinate and stabilize fatty acid synthase, an anti-apoptotic protein whose overexpression is often observed in progressive human tumors[33]. Therefore, USP2a may act as an oncogenic protein in tumor formation or cancer progression. USP11 physically associates with and deubiquitinates BRCA2, but its pro-survival function to DNA damage is independent of its effect on the deubiqui-tination of BRCA2[34]. USP28 has also been found to be involved in DNA damage response; however, its role is to promote DNA damage-induced apoptosis through regulating the pro-apoptotic Chk2–p53–PUMA pathway[35]. On the other hand, BAP1, a ubiquitin C-terminal hydrolase, shows growth inhibitory effects on cancer cells, and this tumor suppressor function depends on its interaction with breast cancer susceptibility gene product BRCA1[36]. Although the precise mechanism of this interaction is not clear, the deubiqui-tinating activity of BAP1 is believed to play a key role.
In a high-throughput RNA interference screen to identify deubiquitinating enzymes involved in cancer, Brummelkamp et al found that the cylindromatosis tumor suppressor (CYLD), which is mutated in familial cylindromatosis, is a USP[37]. CYLD can bind to NEMO (IKKg), deubiquitinate, and inactivate TNF-receptor-associated factors, leading to the inhibition of NF-κB activation. The suppression of CYLD results in the activation of NF-κB and resistance to apoptosis. The deubiquitinating activity of CYLD, its interaction with NEMO, and the inhibitory effect on NF-kB activation, were also discovered in another 2 independent studies[38,39]. Therefore, deubiquitinating enzymes can be either tumor suppressor genes depending on their molecular functions and their roles in diverse signaling pathways. In addition, deubiquitinating enzymes have been reported to be involved in the regulation of cell differentiation, apoptosis, and other physiological or pathological processes[40–42] (Table 1). With further investigations on deubiquitinating enzymes, it can be expected that more insight of the roles and functions of this class of enzymes will be uncovered.
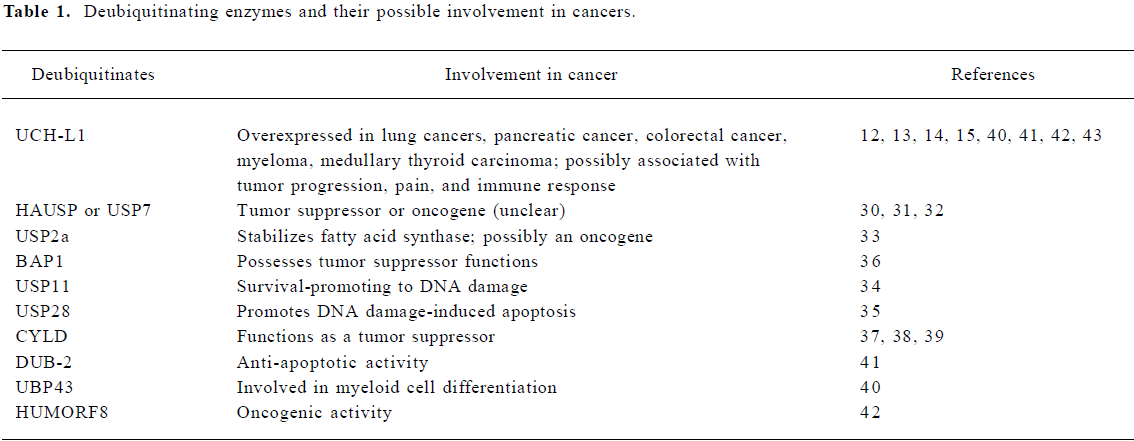
Full table
Deubiquitinating enzymes and other cancer-related problems
Pain occurs in approximately 60%–80% of cancer patients with terminal disease, particularly in patients with metastatic cancer. Although pain in cancer patients can be attributed to many factors, direct tumor involvement is the most common cause. There is evidence that ubiquitin hydrolases might contribute to the occurrence of cancer pain. Angioleiomyoma is a solitary subcutaneous tumor characterized by pain in about half of patients with this tumor. In angioleiomyoma patients suffering from pain, small nerve fiber immunoreactivity for ubiquitin C-terminal hydrolase-L1 was observed within the capsule (77%) and tumor stroma (69%)[43]. Similar observations were reported in other tumors, such as gastrointestinal stromal tumors, fibrosarcoma, and melanoma[44]. These observations suggest that ubiquitin C-terminal hydrolase-L1 as a part of peripheral components plays a role, although as yet unclear yet in cancer-associated pain.
Tumor antigens, which are mostly proteins or peptides expressed or presented by tumor cells, act as signals for immune effectors (T cells and antibodies) to differentiate them from normal tissues. Several deubiquitinating enzymes have been reported to participate in the immune response to cancer. For instance, the expression of ubiquitin C-terminal hydrolase-L1 was found to correlate with T-status in non-small-cell lung cancer[45]. In a proteomic analysis of sera from lung cancer patients, ubiquitin C-terminal hydrolase-L1 was identified as a tumor antigen that induces a humoral immune response[46]. More recently, another ubiquitin hydrolase, ubiquitin C-terminal hydrolase-L3, was found to be involved in the humoral immune response in human colon cancer. Yet the exact roles that these ubiqutin hydrolases play in immune response remain to be elucidated. Because of the structural and functional similarity, one might expect that the involvement of more deubiquitinating enzymes in the immune response to cancer will be uncovered.
Future perspective
Although deubiquitinating enzymes comprise the largest known family of enzymes in the ubiquitin system, at present, little is known about the physiological or pathological roles of those proteins. While deubiquitinating enzymes share a common fundamental biochemical function, they nevertheless serve as controllers of highly diverse cellular processes. In particular, a large number of ubiquitin ligases have been identified and their association with diseases, such as cancer, has begun to be uncovered. For example, the oncogene MDM2 is known to serve as a ubiquitin ligase for p53 and promotes its degradation. The drugs targeting MDM2 have already been developed[47]. With increasing understanding of the functions and roles of the enzymes involved in protein ubiquitination and deubiquitination, we can expect to know more about what these ubiquitin-specific processing proteases and ubiquitin C-terminal hydrolases exactly do during cancer development and progression. This knowledge will certainly provide insight into whether some of those enzymes can serve as prognostic tumor markers or therapeutic targets for cancer. Drugs targeting the ubiquitin–proteasome pathway (such as velcade) have already been proven to be very effective against some types of cancers[48–52]. Compounds that inhibit the activity of some ubiqutin C-terminal hydrolases have also been reported[53], but their implications in the treatment of cancer or other diseases remain to be investigated. It is conceivable that drugs targeting deubiquitinating enzymes will sooner or later be used in treating cancers and other human diseases.
References
- Peters JM, Harris JR, Finley D. Ubiquitin and the biology of the cell. New York and London: Plenum, 1998.
- Wilkinson KD. Regulation of ubiquitin-dependent processes by deubiquitinating enzymes. FASEB J 1997;11:1245-56.
- Wilkinson KD. Ubiquitination and deubiquitination: targeting of proteins for degradation by the proteasome. Semin Cell Dev Biol 2000;11:141-8.
- Chain DG, Casadio A, Schacher S, Hegde AN, Valbrun M, Yamamoto N, et al. Mechanisms for generating the autonomous cAMP-dependent protein kinase required for long-term facilitation in Aplysia. Neuron 1999;22:147-56.
- Verma R, Aravind L, Oania R, McDonald WH, Yates JR III, Koonin EV, et al. Role of Rpn11 metalloprotease in deubiqui-tination and degradation by the 26S proteasome. Science 2002;298:611-5.
- Yao T, Cohen RE. A cryptic protease couples deubiquitination and degradation by the proteasome. Nature 2002;419:403-7.
- Hanna J, Hathaway NA, Tone Y, Crosas B, Elsasser S, Kirkpatrick DS, et al. Deubiquitinating enzyme Ubp6 functions noncataly-tically to delay proteasomal degradation. Cell 2006;127:99-111.
- Liu Y, Fallon L, Lashuel HA, Liu Z, Lansbury PT Jr. The UCH-L1 gene encodes two opposing enzymatic activities that affect alpha-synuclein degradation and Parkinson’s disease susceptibility. Cell 2002;111:209-18.
- Wilkinson KD, Lee KM, Deshpande S, Duerksen-Hughes P, Boss JM, Pohl J. The neuron-specific protein PGP 9.5 is a ubiquitin carboxyl-terminal hydrolase. Science 1989;246:670-3.
- Dhillon AP, Rode J, Dhillon DP, Moss E, Thompson RJ, Spiro SG, et al. Neural markers in carcinoma of the lung. Br J Cancer 1985;51:645-52.
- Rode J, Dhillon AP, Doran JF, Jackson P, Thompson RJ. PGP 9.5, a new marker for human neuroendocrine tumours. Histopathology 1985;9:147-58.
- Hibi K, Westra WH, Borges M, Goodman S, Sidransky D, Jen J. PGP9.5 as a candidate tumor marker for non-small-cell lung cancer. Am J Pathol 1999;155:711-5.
- Tezel E, Hibi K, Nagasaka T, Nakao A. PGP9.5 as a prognostic factor in pancreatic cancer. Clin Cancer Res 2000;6:4764-7.
- Yamazaki T, Hibi K, Takase T, Tezel E, Nakayama H, Kasai Y, et al. PGP9.5 as a marker for invasive colorectal cancer. Clin Cancer Res 2002;8:192-5.
- Otsuki T, Yata K, Takata-Tomokuni A, Hyodoh F, Miura Y, Sakaguchi H, et al. Expression of protein gene product 9.5 (PGP9.5)/ubiquitin-C-terminal hydrolase 1 (UCHL-1) in human myeloma cells. Br J Haematol 2004;127:292-8.
- Al-Katib AM, Mohammad RM, Maki A, Smith MR. Induced expression of a ubiquitin COOH-terminal hydrolase in acute lymphoblastic leukemia. Cell Growth Differ 1995;6:211-7.
- Mohammad RM, Maki A, Pettit GR, Al-Katib AM. Bryostatin 1 induces ubiquitin COOH-terminal hydrolase in acute lymphoblastic leukemia cells. Enzyme Protein 1996;49:262-72.
- Caballero OL, Resto V, Patturajan M, Meerzaman D, Guo MZ, Engles J, et al. Interaction and colocalization of PGP9.5 with JAB1 and p27(Kip1). Oncogene 2002;21:3003-10.
- Slingerland J, Pagano M. Regulation of the cdk inhibitor p27 and its deregulation in cancer. J Cell Physiol 2000;183:10-17.
- Hommura F, Dosaka-Akita H, Mishina T, Nishi M, Kojima T, Hiroumi H, et al. Prognostic significance of p27KIP1 protein and ki-67 growth fraction in non-small cell lung cancers. Clin Cancer Res 2000;6:4073-81.
- Yao J, Eu KW, Seow-Choen F, Cheah PY. Down-regulation of p27 is a significant predictor of poor overall survival and may facilitate metastasis in colorectal carcinomas. Int J Cancer 2000;89:213-6.
- Hirano K, Minamoto T. Altered expression of p53 and p27 proteins, alone or combined, as a predictor of metastatic potential in early invasive carcinoma of colon and rectum—a comparative clinicopathologic and molecular analysis. Cancer Detect Prev 2000;24:343-55.
- Gavioli R, Frisan T, Vertuani S, Bornkamm GW, Masucci MG. c-myc overexpression activates alternative pathways for intracellular proteolysis in lymphoma cells. Nat Cell Biol 2001;3:283-8.
- Rolen U, Kobzeva V, Gasparjan N, Ovaa H, Winberg G, Kisseljov F, et al. Activity profiling of deubiquitinating enzymes in cervical carcinoma biopsies and cell lines. Mol Carcinog 2006;45:260-9.
- Ciechanover A, DiGiuseppe JA, Bercovich B, Orian A, Richter JD, Schwartz AL, et al. Degradation of nuclear oncoproteins by the ubiquitin system in vitro. Proc Natl Acad Sci USA 1991;88:139-43.
- Maki CG, Huibregtse JM, Howley PM. In vivo ubiquitination and proteasome-mediated degradation of p53(1). Cancer Res 1996;56:2649-54.
- Lipkowitz S. The role of the ubiquitination-proteasome pathway in breast cancer: ubiquitin mediated degradation of growth factor receptors in the pathogenesis and treatment of cancer. Breast Cancer Res 2003;5:8-15.
- Shtiegman K, Yarden Y. The role of ubiquitylation in signaling by growth factors: implications to cancer. Semin Cancer Biol 2003;13:29-40.
- Amit S, Ben-Neriah Y. NF-kappaB activation in cancer: a challenge for ubiquitination- and proteasome-based therapeutic approach. Semin Cancer Biol 2003;13:15-28.
- Li M, Chen D, Shiloh A, Luo J, Nikolaev AY, Qin J, et al. Deubiquitination of p53 by HAUSP is an important pathway for p53 stabilization. Nature 2002;416:648-53.
- Cummins JM, Vogelstein B. HAUSP is required for p53 destabilization. Cell Cycle 2004;3:689-92.
- Li M, Brooks CL, Kon N, Gu W. A dynamic role of HAUSP in the p53-Mdm2 pathway. Mol Cell 2004;13:879-86.
- Graner E, Tang D, Rossi S, Baron A, Migita T, Weinstein LJ, et al. The isopeptidase USP2a regulates the stability of fatty acid synthase in prostate cancer. Cancer Cell 2004;5:253-61.
- Schoenfeld AR, Apgar S, Dolios G, Wang R, Aaronson SA. BRCA2 is ubiquitinated in vivo and interacts with USP11, a deubiquitinating enzyme that exhibits prosurvival function in the cellular response to DNA damage. Mol Cell Biol 2004;24:7444-55.
- Zhang D, Zaugg K, Mak TW, Elledge SJ. A role for the deubiquitinating enzyme USP28 in control of the DNA-damage response. Cell 2006;126:529-42.
- Jensen DE, Proctor M, Marquis ST, Gardner HP, Ha SI, Chodosh LA, et al. BAP1: a novel ubiquitin hydrolase which binds to the BRCA1 RING finger and enhances BRCA1-mediated cell growth suppression. Oncogene 1998;16:1097-112.
- Brummelkamp TR, Nijman SM, Dirac AM, Bernards R. Loss of the cylindromatosis tumour suppressor inhibits apoptosis by activating NF-kappaB. Nature 2003;424:797-801.
- Trompouki E, Hatzivassiliou E, Tsichritzis T, Farmer H, Ashworth A, Mosialos G. CYLD is a deubiquitinating enzyme that negatively regulates NF-kappaB activation by TNFR family members. Nature 2003;424:793-6.
- Kovalenko A, Chable-Bessia C, Cantarella G, Israel A, Wallach D, Courtois G. The tumour suppressor CYLD negatively regulates NF-kappaB signalling by deubiquitination. Nature 2003;424:801-5.
- Liu LQ, Ilaria R Jr, Kingsley PD, Iwama A, van Etten RA, Palis J, et al. A novel ubiquitin-specific protease, UBP43, cloned from leukemia fusion protein AML1-ETO-expressing mice, functions in hematopoietic cell differentiation. Mol Cell Biol 1999;19:3029-38.
- Migone TS, Humbert M, Rascle A, Sanden D, D’Andrea A, Johnston JA. The deubiquitinating enzyme DUB-2 prolongs cytokine-induced signal transducers and activators of transcription activation and suppresses apoptosis following cytokine withdrawal. Blood 2001;98:1935-41.
- Janssen JW, Schleithoff L, Bartram CR, Schulz AS. An oncogenic fusion product of the phosphatidylinositol 3-kinase p85beta subunit and HUMORF8, a putative deubiquitinating enzyme. Oncogene 1998;16:1767-72.
- Geddy PM, Gray S, Reid WA. Mast cell density and PGP 9.5-immunostained nerves in angioleiomyoma: their relationship to painful symptoms. Histopathology 1993;22:387-90.
- Zhang HW, Iida Y, Andoh T, Nojima H, Murata J, Saiki I, et al. Mechanical hypersensitivity and alterations in cutaneous nerve fibers in a mouse model of skin cancer pain. J Pharmacol Sci 2003.167-70.
- Sasaki H, Yukiue H, Moriyama S, Kobayashi Y, Nakashima Y, Kaji M, et al. Expression of the protein gene product 9.5, PGP9.5, is correlated with T-status in non-small cell lung cancer. Jpn J Clin Oncol 2001;31:532-5.
- Brichory F, Beer D, Le Naour F, Giordano T, Hanash S. Proteomics-based identification of protein gene product 9.5 as a tumor antigen that induces a humoral immune response in lung cancer. Cancer Res 2001;61:7908-12.
- Vassilev LT, Vu BT, Graves B, Carvajal D, Podlaski F, Filipovic Z, et al. In vivo activation of the p53 pathway by small-molecule antagonists of MDM2. Science 2004;303:844-8.
- Adams J. Proteasome inhibitors as new anticancer drugs. Curr Opin Oncol 2002.628-34.
- Lenz HJ. Clinical update: proteasome inhibitors in solid tumors. Cancer Treat Rev 2003;29 Suppl 1:41-8.
- Richardson P. Clinical update: proteasome inhibitors in hematologic malignancies. Cancer Treat Rev 2003;29 Suppl 1:33-9.
- Orlowski RZ, Dees EC. The role of the ubiquitination-proteasome pathway in breast cancer: applying drugs that affect the ubiquitin-proteasome pathway to the therapy of breast cancer. Breast Cancer Res 2003;5:1-7.
- Mack PC, Davies AM, Lara PN, Gumerlock PH, Gandara DR. Integration of the proteasome inhibitor PS-341 (Velcade) into the therapeutic approach to lung cancer. Lung Cancer 2003;41 Suppl 1:S89-96.
- Liu Y, Lashuel HA, Choi S, Xing X, Case A, Ni J, et al. Discovery of inhibitors that elucidate the role of UCH-L1 activity in the H1299 lung cancer cell line. Chem Biol 2003;10:837-46.