Depside salts from Salvia miltiorrhiza improve myocardial microperfusion in rats using laser Doppler flowmetry1
Introduction
Danshen, the dried roots of the medicinal plant Salvia miltiorrhiza, is a traditional Chinese herbal medicine used for the treatment of coronary heart disease. Depside salts from S miltiorrhiza are novel drugs in which magnesium lithospermate B (MLB, Figure 1) and its analogues are the active components. The pharmacological activities of MLB, which is a major aqueous extract ingredient of Danshen, have been extensively investigated. MLB has been shown to reduce the size of myocardial infarction[1] as Danshen does[2]. Further studies investigated the involving mechanisms in several aspects. First, it was speculated that the antioxidative and radical scavenging property of MLB played an essential role in its cardioprotective efficacy[3–6]. Second, MLB was reported to stimulate the production of nitric oxide in endo-thelial cells[7–9], possibly via the induction of constitutive NOS (cNOS) expression[7]. Third, MLB was demonstrated to behave in a Ca2+ antagonistic fashion voltage-dependently[10] and inhibited extracellular calcium influx[8,9]. In addition, MLB suppressed apoptosis induced by myocardial ischemia/reperfusion[11,12], probably through the inhibition of the c-Jun N-terminal kinase 3 (JNK 3) activity[11] and stress-activated protein (SAP) kinase activity[12]. However, there has been no direct evidence on its effect on coronary micro-circulation.
Laser Doppler flowmetry, which offers a continuous real-time measurement of blood cell perfusion in the microcirculatory beds of tissues, is a prospective method to be applied for myocardial microvascular perfusion assessment. Ahn et al[13] demonstrated that their data of the local myocardium perfusion correlated well with coronary sinus blood flow in the empty-beating hearts of pigs. Sidi et al[14], who considered movement artifacts from the contraction of myocardium, found that the local changes in flow measured by laser Doppler flowmetry correlated with the regional myocardial blood flow changes obtained by means of radioactive microspheres, but not with global contractility which was evaluated by first-time derivative of left ventricle pressure (LV dp/dt). Klassen et al[15] proposed a method to overcome the limits in measuring regional phasic myocardial red cell flux during a cardiac cycle using a laser Doppler velocimeter by inserting the probe into the beating heart in various locations. They suggested that the fiber tip moved in concert with the contracting muscle fibers with little tethering of myocardial action, thus only registering movements from the red blood cells. They also confirmed their hypothesis subsequently in the canine myocardium[16]. Therefore, it appears feasible to apply the laser Doppler technique quantitatively in evaluating the responses of drugs to myocardial microperfusion.
In the present study, we employed the laser Doppler flowmetry system to investigate the effect of S miltiorrhiza depside salts on myocardial microperfusion. We found that S miltiorrhiza depside salts improved coronary microcircula-tion, as well as cardiac output (CO).
Materials and methods
Drugs and reagents S miltiorrhiza depside salts which contain MLB (≥80.0%) were provided by the Department of Phytochemistry, Shanghai Institute of Materia Medica (Shanghai, China). Nifedipine (Sigma, USA) was dissolved in a 5% DMSO/physiological saline solution, and the other drugs involved were dissolved in physiological saline only. Drug concentrations were adjusted to yield an injection volume of 0.1 mL/100 g of body weight.
Experimental preparation Male Sprague-Dawley (SD) rats (Certificate N
Determinations of CO A left-sided thoracotomy was performed from the second to the fourth intercostal space to expose the lungs in the thoracic cavity, and the heart was suspended in a pericardial cradle. After the ascending aorta had been gently isolated from the pulmonary artery by blunt dissection, the ultrasonic perivascular flow probe (2SB) of ultrasonic Doppler flowmetry (T206, Transonic Systems Inc, Ithaca, NY, USA) was mounted on the root of the ascending aorta, with the sterile surgilube jelly (E Fougera & Co, USA) filling the probe window to get rid of air bubbles, which permitted the transmission of the ultrasound signal. Additional jelly, loaded by a 20 mL syringe, was injected into the flow probe lumen if necessary.
Determinations of myocardial microperfusion To assess microcirculation, a needle probe (MNP110, length 25 mm, diameter 480 µm) of laser Doppler flowmetry (Powerlab ML191, ADInstruments, Australia) for microcirculation was inserted into a specially-designed holder which was glued to the epicardium of the left ventricular myocardium close to the left anterior descending coronary artery where no obvious large vessels lay. The orientation of probe was appropriately adjusted against the holder until a stable, high-quality signal for laser Doppler flowmetry was obtained. The laser Doppler flowmetry signal was recorded as arbitrary blood perfusion units (BPU), a relative unit scale defined by reference to a controlled motility standard. Due to the flexibility of the optical fiber and the plastic harmonious movement of the light probe accompanying the beating heart, virtually no tethering of the myocardium took place.
Protocol The doses of S miltiorrhiza depside salts were selected based on the pilot study and the literature[1–4]. After thoracotomy with instrumentation, the rats underwent a recovery period of 30 min for hemodynamics stabilization. The test drugs were injected intravenously, and no animal received more than 1 agent, except those who received vehicle control administration. After each drug was intravenously administered within 15 s, the changes of all para-meters, recorded simultaneously by a computer using the chart software version 5.4.1 (ADInstruments, Australia), were measured for 30 min consistently.
Criteria for an acceptable experiment The rats whose MAP was below 80 mmHg after stabilization were excluded, as well as those who failed to maintain stable within 1 h post-operatively. It was essential that arterial blood gases remained within the physiological range, and standardized respiratory monitoring was performed.
Calculation of hemodynamics The flow-probe cable was connected to a model T206 flow meter (Transonic Systems Inc, USA) to measure CO[12], which was equal to the ascending aortic flow and was expressed as flow per kg of body weight (CI). MAP was automatically calculated from femoral artery pressure by the software. Systemic vascular resistance (SVR) was determined using the following formula: SVR=MAP/CI. Cardiac inotropy was estimated using the maximal velocity of flow increase (df/dtmax)[27]. Myocardial microperfusion, detected by laser Doppler flowmetry, was quantified by change percentage due to the temporal and spatial microheterogeneity of the myocardial blood flow[13], as well as the small region of the myocardium under observation. The mean values for each determination were analyzed over a 10 s period.
Data statistical analysis Results were expressed as mean±SD. Parameters at the same time point were compared with the vehicle control by unpaired Student’s t-test. Student’s paired t-test was used for comparison of the parameters with their corresponding baseline values. A value of P<0.05 was considered significant.
Results
Feasibility of laser Doppler flowmetry for myocardial microperfusion assessment Myocardial microperfusion profile was measured for up to 30 min without intervention. Ascending aortic flow, femoral artery pressure, and myocardial microperfusion were recorded for approximately 10 min while no intervention occurred (Figure 2). When a satisfactory positioning of the probe was made, a distinct, regular, consistent pattern of myocardial microperfusion waveform was observed which remained constant during control and changed correspondingly, following an intervention, as that of other parameters. Ventilation played a pivotal role in the behavior of hemodynamics, and myocardial microperfusion varied correspondingly as well (data not shown). No alteration in either the pattern or value of myocardial microperfu-sion was observed as long as ascending aortic flow and femoral artery pressure were constant (Figure 2).
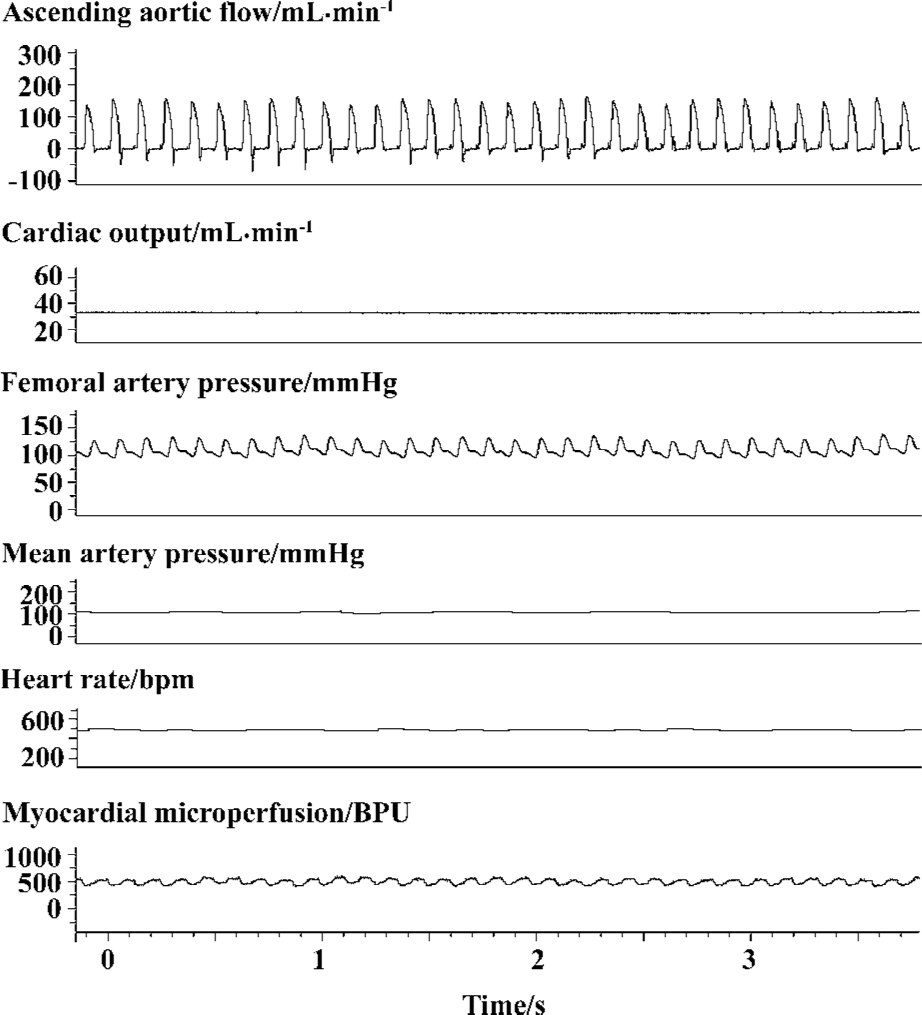
The myocardial microperfusion profile recorded by a laser Doppler flowmetry device behaved in a phasic fashion throughout the cardiac cycle (Figures 2, 3). The relationships between ascending aortic flow, femoral artery pressure, and myocardial microperfusion in cardiac cycles are shown (Figure 3). As we know, diastole originated at the same time point as when the ascending aortic flow returned to the baseline and was indicated by the closure of the aortic valve. The blood perfusion waveform, which increased with the femoral artery pressure and decreased gradually after reaching a peak in diastole, showed a dicrotic notch, as seen in the femoral artery pressure to some extent. The peak of ascending aortic flow from which CO was calculated coincided with the nadir of the myocardial microperfusion waveform where the blood flow started to increase until the early period of diastole (Figure 3). The combined curves showed that the myocardial microperfusion was predominately diastolic and its phase was opposite to the ascending aortic flow.
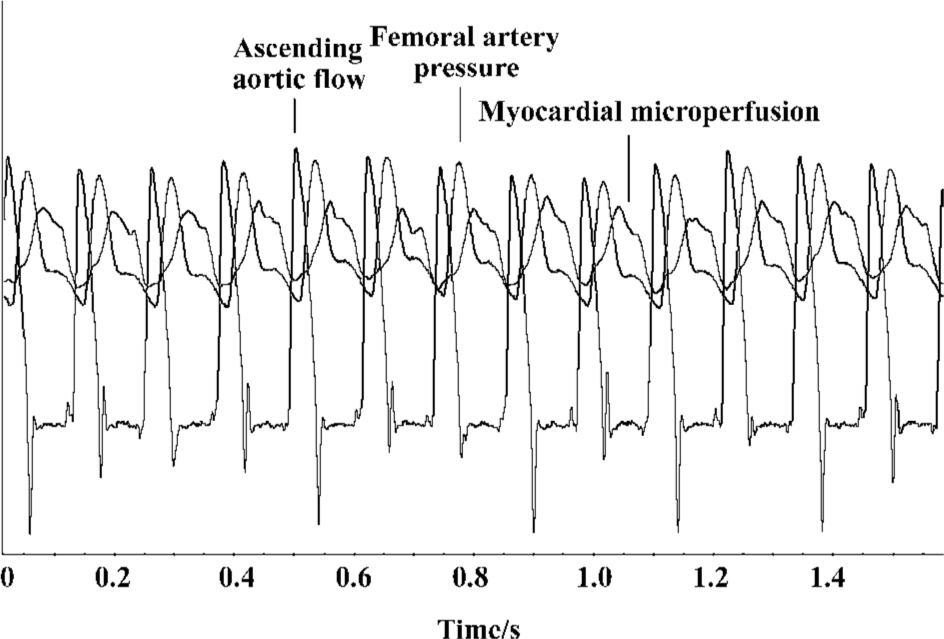
Representative vasodilators, nifedipine and captopril, were selected for system test and confirmation. The mean basic values of myocardial microperfusion observed in each group for 75 µg/kg nifedipine, 5 mg/kg captopril, and vehicle control were 471±58 BPU, 458±74 BPU, and 445±58 BPU, respectively. Our data showed that intravenous administration of both 75 µg/kg nifedipine and 5 mg/kg captopril exerted an increasing effect on myocardial microperfusion, as well as on CO (Table 1). Intravenous administration of 75 µg/kg nifedipine increased myocardial microperfusion by 7.1%±2.9% (P<0.01) maximally, and 5 mg/kg captopril improved myocardial microperfusion 4 min after drug intervention with a peak increase of 9.5%±4.6% (P<0.01). Typical tracings of CO, artery pressure, and myocardial microperfu-sion after the intravenous administration of 75 µg/kg nifedi-pine are shown in Figure 4.
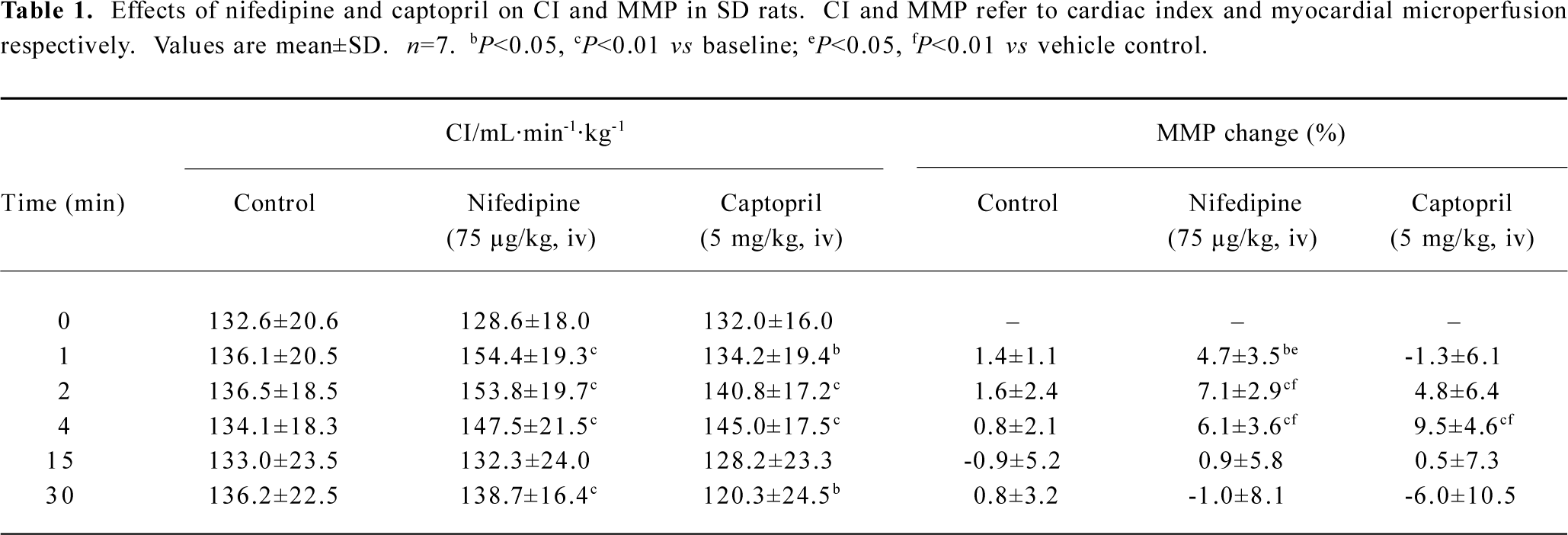
Full table
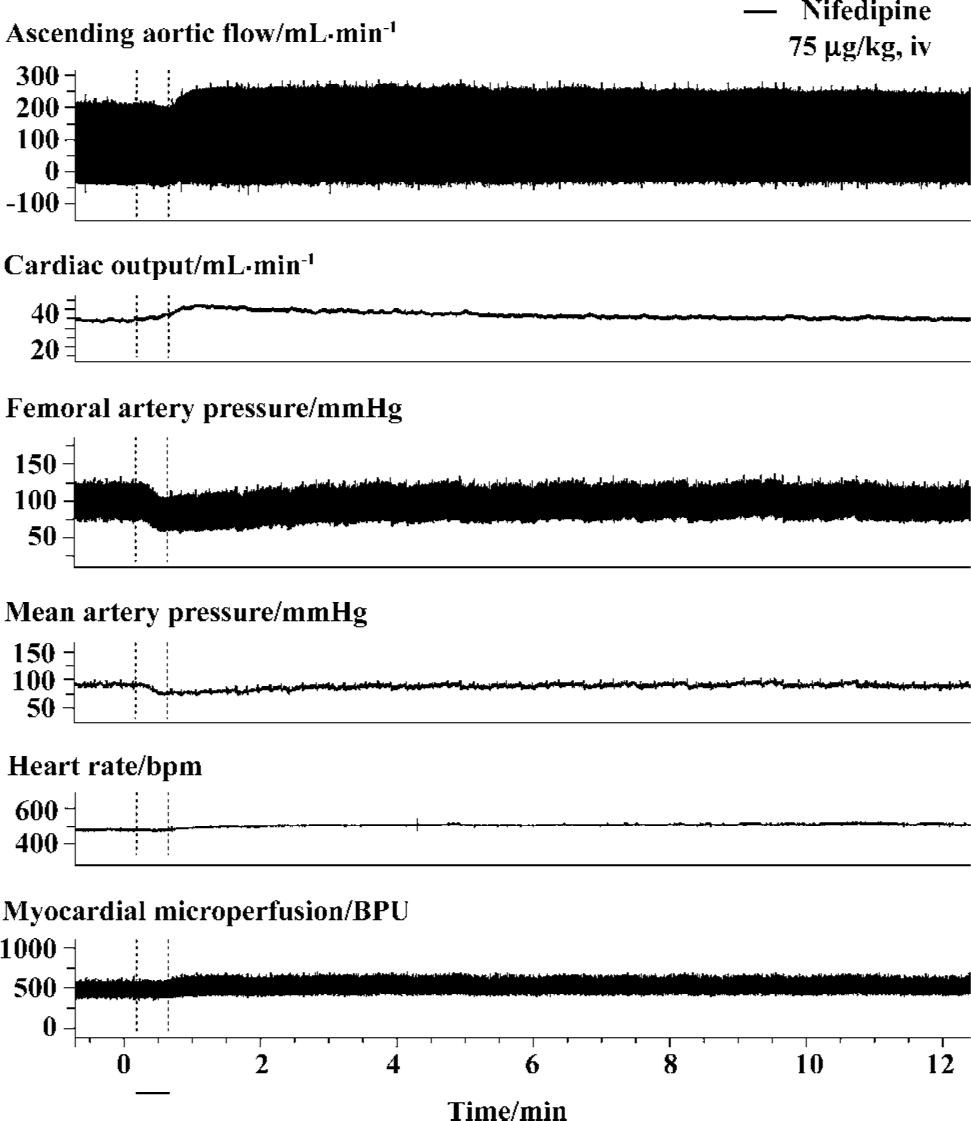
Effects of S miltiorrhiza depside salts on hemodynamics Intravenous administration of S miltiorrhiza depside salts resulted in a significant immediate increase in CO and cardiac inotropy, as well as a fall in SVR (Figure 5). S miltiorrhiza depside salts (30 mg/kg and 60 mg/kg) increased CI by 12.2%±6.3% (P<0.01) and 20.1%±3.5% (P<0.01) from the baseline, respectively, which was in a dose-dependent manner. S miltiorrhiza depside salts (30 mg/kg and 60 mg/kg) caused an increase in cardiac inotropy with a peak value of 21.7%±6.8% (P<0.01) and 50.5%±18.9% (P<0.01) 0.5 min after injection, respectively. However, 30 mg/kg S miltior-rhiza depside salts showed a depressant effect 10–20 min after injection. A slight fall in MAP appeared 10 min after 30 mg/kg S miltiorrhiza depside salts were intravenously administered, with a significant decrease at the 15 min time point (Figure 5C). Conversely, 60 mg/kg S miltiorrhiza depside salts exerted an increasing effect on MAP in the initial period by 17.1%±9.4% (P<0.01 vs baseline) maximally. Cardiac inotropy dropped significantly and reverted to the baseline soon after.
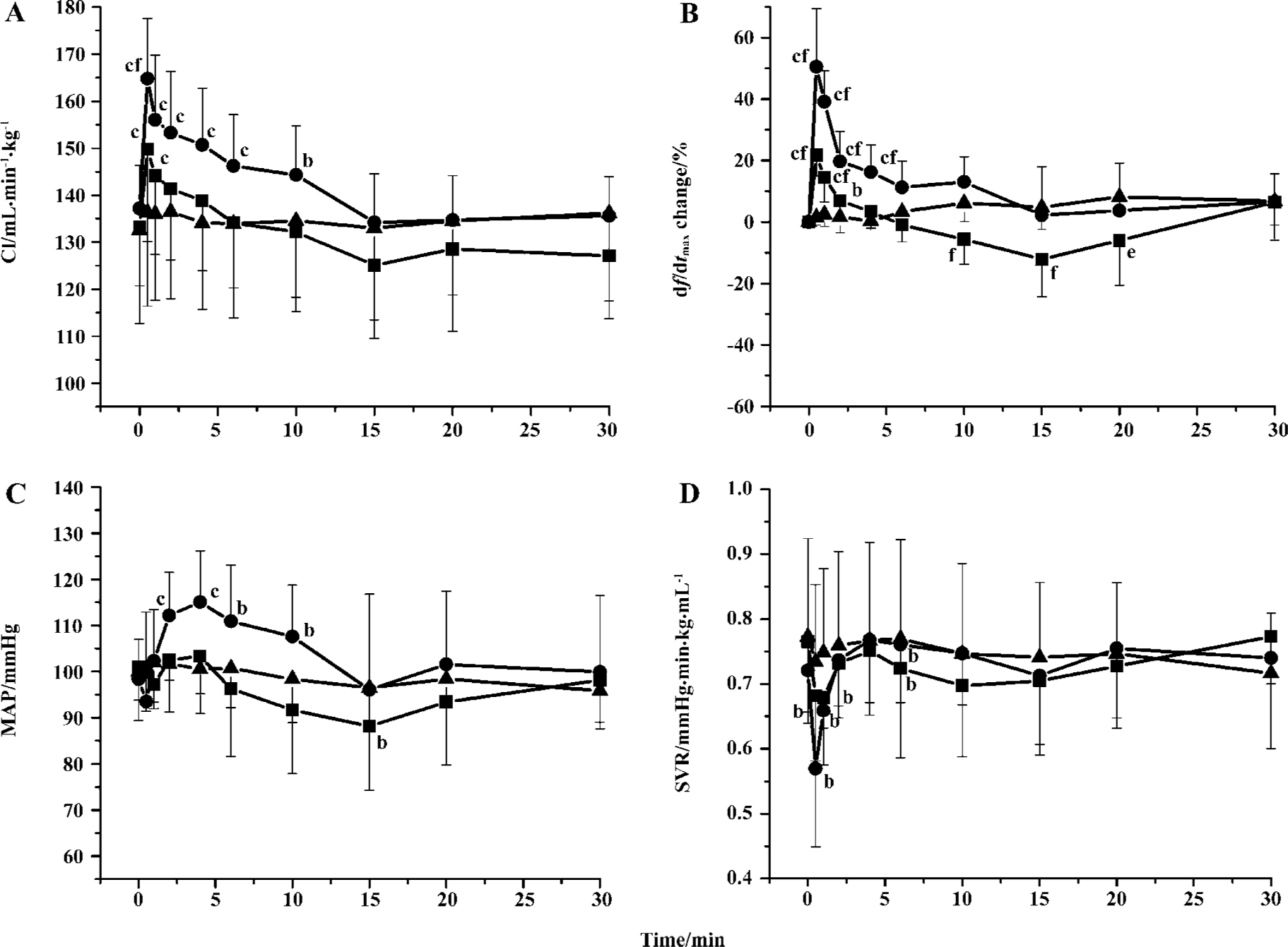
Effects of S miltiorrhiza depside salts on myocardial microperfusion The effect of S miltiorrhiza depside salts (30 mg/kg and 60 mg/kg) on myocardial microperfusion in the LV was measured in 7 open-chest anesthetized rats (Figure 6). Myocardial microperfusion maximally increas-ed by 6.3%±2.9% (P<0.01) and 9.6%±4.0% (P<0.01) for 30 mg/kg and 60 mg/kg S miltiorrhiza depside salts, respectively. The profile of myocardial microperfusion with S miltiorrhiza depside salts intervention returned to the baseline in no more than 15 min.
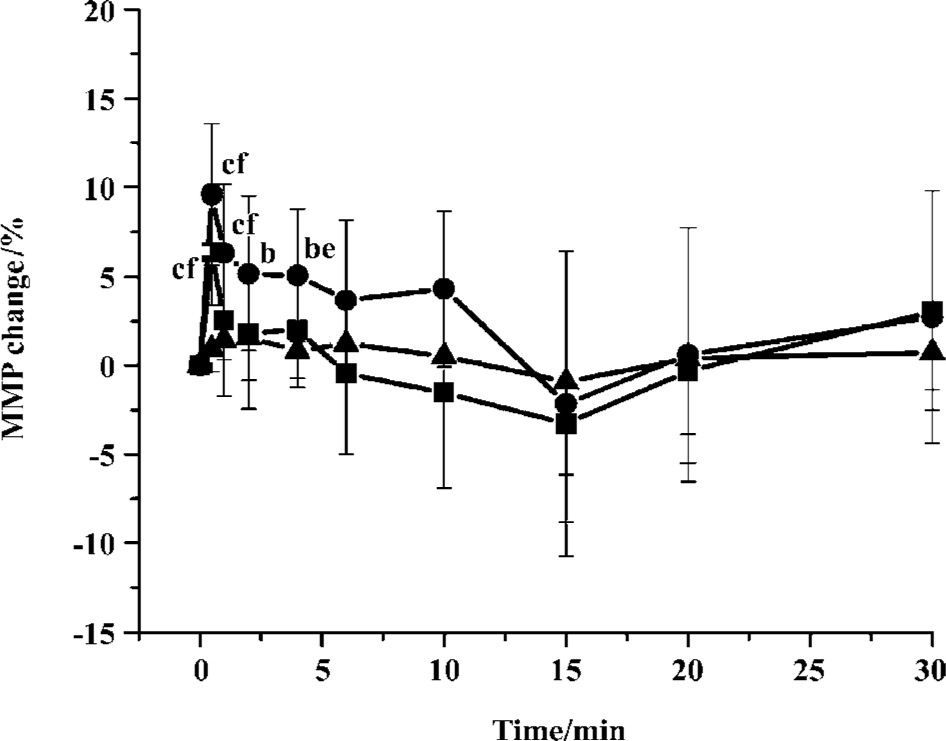
Discussion
Coronary microcirculation plays a pivotal role in determining the supply of oxygen and nutrients to the myocardium. Analyses of coronary microcirculation are important for understanding the pathophysiology of ischemic hearts and the effect of cardioprotective agents. Although the cardio-protec-tive property of MLB has been studied extensively, as well as those of S miltiorrhiza depside salts, there is still no report concerning their effect on coronary micro-circulation. To our knowledge, we demonstrated here for the first time that S miltiorrhiza depside salts could improve myocardial microperfusion using laser Doppler flowmetry, as well as CO.
A number of strategies have been previously employed to optimize the laser Doppler system to overcome movement artifacts so that it can be used for myocardial microvascular measurement[13–19]. In the present study, we proposed that the probe was satisfactorily positioned when a distinct and regular waveform pattern was observed during baseline measurements and was altered following intervention, as suggested by Klassen et al[15]. Kiyooka et al[20] reported that red blood cell flow in the epimyocardium capillaries was predominant during either systole or diastole, acting as the watershed between diastolic arterial and systolic venous flows. Besides, the diameters of subepicardial arterioles remained basically constant during the entire cardiac cycle[17]. In our research, data showed that the phasic profile of myocardial microperfusion by laser Doppler flowmetry, which reflected both arterial inflow and venous outflow, was predominately diastole, which was identical to previous studies[17,20]. Furthermore, the representative vasodilator nifedipine was studied in our system and showed significant increasing effects on myocardial microperfusion as reported[21,22], so did captopril as predicted, which in turn convinced us that the laser Doppler system was sensitive enough to the responses of coronary microvascular to drugs for measure-ment.
In our study, the improving effect of intravenously administered S miltiorrhiza depside salts on CI was in a dose-related manner. After a bolus injection of S miltiorrhiza depside salts, we observed significant increases in CI and myocardial microperfusion prior to minor changes of MAP, which suggested that S miltiorrhiza depside salts might improve cardiac function directly.
Yokozawa et al[23] reported that oral administration of MLB produced a significant reduction in blood pressure in rats with sodium-induced hypertension. The studies by Kamata et al[24,25] also showed that intravenous injection of lithospermic acid B (10–30 mg/kg) decreased the blood pressure in a dose-dependent manner and behaved as an endothelium-dependent vasodilator. In the current study, the effect of 30 mg/kg S miltiorrhiza depside salts on MAP was identical to previous studies, however, 60 mg/kg S miltior-rhiza depside salts resulted in a marked increase in MAP in the initial period of observation when CI was promoted significantly. We presumed that it resulted from the overwhelming CO increasing effect which consequently concealed the vasodilation property of S miltiorrhiza depside salts.
In conclusion, S miltiorrhiza depside salts improve myocardial microperfusion, as well as CO, which provides further evidence for the treatment of S miltiorrhiza depside salts on coronary heart diseases.
References
- Fung KP, Zeng LH, Wu J, Wong HNC, Lee CM, Hon PM, et al. Demonstration of the myocardial salvage effect of lithospermic acid B isolated from the aqueous extract of Salvia miltiorrhiza. Life Sci 1993;52:PL239-44.
- Zhou W, Ruigrok TJC. Protective effect of Danshen during myocardial ischemia and reperfusion: an isolated rat heart study. Am J Chin Med 1990;18:19-24.
- Fung KP, Wu J, Zeng LH, Wong HN, Lee CM, Hon PM, et al. Lithospermic acid B as an antioxidant-based protector of cultured ventricular myocytes and aortic endothelial cells of rabbits. Life Sci 1993;53:PL189-93.
- Yokozawa T, Chung HY, Dong E, Oura H. Confirmation that magnesium lithospermate B has a hydroxyl radical-scavenging action. Exp Toxicol Pathol 1995;47:341-4.
- Wu XJ, Wang YP, Wang W. Free radical scavenging and inhibition of lipid peroxidation by magnesium lithospermate B. Acta Pharmacol Sin 2000;21:855-8.
- Soung do Y. Peroxynitrite scavenging activity of lithospermate B from Salvia miltiorrhiza. J Pharm Pharmacol 2003;55:1427-32.
- O K. Cheung F, Sung FL, Zhu DY, Siow YL. Effect of magnesium tanshinoate B on the production of nitric oxide in endothelial cells. Mol Cell Biochem 2000;207:35-9.
- Luo WB, Wang YP. Magnesium lithospermate B inhibits hypoxia-induced calcium influx and nitric oxide release in endothelial cells. Acta Pharmacol Sin 2001;22:1135-42.
- Luo WB, Dong L, Wang YP. Effect of magnesium lithospermate B on calcium and nitric oxide in endothelial cells upon hypoxia/reoxygenation. Acta Pharmacol Sin 2002;23:930-6.
- Wang W, Hu GY, Wang YP. Selective modulation of L-type calcium current by magnesium lithospermate B in guinea-pig ventricular myocytes. Life Sci 2006;78:2989-97.
- Yang LM, Xiao YL, Ou-Yang JH. Inhibition of magnesium lithospermate B on the c-Jun N-terminal kinase 3 mRNA expression in cardiomyocytes encountered ischemia/reperfusion injury. Acta Pharm Sin 2003;38:487-91. Chinese..
- Au-Yeung KK, Zhu DY. O K, Siow YL. Inhibition of stress-activated protein kinase in the ischemic/reperfused heart: role of magnesium tanshinoate B in preventing apoptosis. Biochem Pharmacol 2001;62:483-93.
- Ahn HC, Ekroth R, Hedenmark J, Nilsson GE, Svedjeholm R. Assessment of myocardial perfusion in the empty beating porcine heart with laser Doppler flowmetry. Cardiovasc Res 1988;22:719-25.
- Sidi A, Rush W. An alternative to radioactive microsphere for measuring regional myocardial blood flow, Part 2: Laser-Doppler perfusion monitor. J Cardiothorac Vasc Anesth 1996;10:374-7.
- Klassen GA, Barclay KD, Wong R, Paton B, Wong AY. Red cell flux during the cardiac cycle in the rabbit myocardial microcirculation. Cardiovasc Res 1997;34:504-14.
- Barclay KD, Klassen GA, Wong RW, Wong AY. A method for measuring systolic and diastolic microcirculatory red cell flux within the canine myocardium. Ital Heart J 2001;2:740-50.
- Ashikawa K, Kanatsuka H, Suzuki T, Takishima T. Phasic blood flow velocity pattern in epimyocardial microvessels in the beating canine left ventricle. Circ Res 1986;59:704-11.
- Mizutani T, Takao M, Onoda K, Katayama Y, Yada I, Yuasa H, et al. Laser measurement of myocardial blood flow. J Clin Laser Med Surg 1991;9:439-43.
- Karlsson MG, Casimir-Ahn H, Lonn U, Wardell K. Analysis and processing of laser Doppler perfusion monitoring signals recorded from the beating heart. Med Biol Eng Comput 2003;41:255-62.
- Kiyooka T, Hiramatsu O, Shigeto F, Nakamoto H, Tachibana H, Yada T, et al. Direct observation of epicardial coronary capillary hemodynamics during reactive hyperemia and during adenosine administration by intravital video microscopy. Am J Physiol Heart Circ Physiol 2005;288:H1437-43.
- Tillmanns H, Neumann FJ, Parekh N, Waas W, Moller P, Zimmermann R, et al. Calcium antagonists and myocardial microperfusion. Drugs 1991;42 Suppl 1:1-6.
- Weis M, Pehlivanli S, von Scheidt W. Vasodilator response to nifedipine in human coronary arteries with endothelial dysfunction. J Cardiovasc Pharmacol 2002;39:172-80.
- Yokozawa T, Lee TW, Oura H, Nonaka G, Nishioka I. Effect of magnesium lithospermate B in rats with sodium-induced hypertension and renal failure. Nephron 1992;60:460-5.
- Kamata K, Noguchi M, Nagai M. Hypotensive effects of lithospermic acid B isolated from the extract of Salviae miltior-rhizae Radix in the rat. Gen Pharmacol 1994;25:69-73.
- Kamata K, Iizuka T, Nagai M, Kasuya Y. Endothelium-dependent vasodilator effects of the extract from Salviae miltiorrhizae radix: a study on the identification of lithospermic acid B in the extracts. Gen Pharmacol 1993;24:977-81.
- Maturi MF, Martin SE, Markle D, Maxwell M, Burruss CR, Speir E, et al. Coronary vasoconstriction induced by vasopressin. Production of myocardial ischemia in dogs by constriction of nondiseased small vessels. Circulation 1991;83:2111-21.
- Thoren P, Sjolander M, Nordlander M. Hemodynamic effects of calcium antagonists with high or low vascular selectivity. J Cardiovasc Pharmacol 1990;15 Suppl 4:S21-4.