Curcumin downregulates homeobox gene NKX3.1 in prostate cancer cell LNCaP1
Introduction
Prostate cancer is the most common cancer in males, and the second leading cause of male cancer deaths in developed countries[1]. Current prostate cancer therapies such as surgery, chemotherapy, and radiation therapy are of limited efficacy and may not avoid the significant side-effects. Androgen reduction therapy is commonly used to control hormone-sensitive tumor cells; however, hormone refractory clones often emerge after hormonal therapy[2]. Advanced hormone refractory prostate cancer is almost incurable[3]. Therefore, novel effective therapies, including biotherapy, are urgently needed to be developed.
In a search for alternative and preventive therapies for prostate cancer, attention has been focused on plant poly-phenolic compounds, which are rich in nutritional supplements such as soybeans, garlic, and green tea, and have been used to augment anticancer therapies[4,5]. Curcumin, the active component of turmeric, is a dietary constituent that has received a great deal of attention recently as a chemoprotective agent[6,7]. Several recent observations have shown that curcumin has antioxidant and anti-inflammatory activities, as well as anticarcinogenic activity in colon cancer, breast cancer[8,9], and leukemia[10]. The molecular mechanisms of the actions of curcumin are beginning to be elucidated, including the effects of suppressing tumor initiation and promotion in animal models. Curcumin is a potent inhibitor of cycloxygenase-2, lipoxygenase, ornithine decarboxylase[11–13], c-Jun/AP-1, nuclear factor-κB[14,15], c-Jun N-terminal kinase, and protein kinase C[16,17]. Curcumin inhibited epidermal growth factor receptor activity in various tumors, including prostate carcinoma[18]. Curcumin can cause a marked decrease in cell proliferation and microvessel density, and an increase in apoptosis in prostate tumors[19]. By virtue of its multiple effects, curcumin has potential clinical application in the prevention of prostate cancer.
NKX3.1 has been identified as the androgen-regulated NK-class homeobox gene that is largely specific to prostate for expression, and is thought to play an important role in normal prostate organogenesis and carcinogenesis[20–23]. Human NKX3.1 has been mapped to human chromosome 8p21, a locus that experiences a frequent loss of heterozygosity in human prostate cancer, raising the possibility that NKX3.1 may be a tumor suppresser gene[22–24]. It has been reported that the loss of a single allele may predispose to prostate carcinogenesis.
As a gene regulated by androgen, the maintaining expression of NKX3.1 in the prostatic epithelium is dependent on androgen signaling[20,22–23]. However, it is not very clear whether this regulation occurs directly through the interaction of the androgen receptor (AR) and the NKX3.1 promoter.
In this study, an experimental investigation was undertaken to characterize the effect of curcumin on NKX3.1 expression in the prostate cancer cell LNCaP, and to investigate the mechanisms in which curcumin downregulates the homeobox gene NKX3.1 in the prostate cancer cell.
Materials and methods
Cell culture and treatments The human prostate cancer cell line LNCaP was obtained from the American Type Culture Collection (Manassas, Virginia, USA). This cell line was established from a lymph node metastasis of a prostate cancer patient and expressed mutant, but functional on AR. The LNCaP cells were seeded in 35 mm culture dishes in RPMI- 1640 medium supplemented with 10% fetal bovine serum (FBS) and 5% CO2 at 37 °C until reaching approximately 50%–70% confluence. The cells were maintained in serum-free RPMI 1640 medium for a further 24 h before the experiments. The cells were then treated with designated curcumin, R1881, or flutamide in RPMI 1640 medium containing 5% FBS. Curcumin (N
RT-PCR analysis Total RNA was extracted from the LNCaP cells with Trizol reagent (MBI Fermentas, Burlington, ON, Canada) following the manufacturer’s instructions, and the expression of NKX3.1 mRNA was determined by RT-PCR using M-MuL V reverse transcriptase in the presence of random hexamer primers. The PCR primers for NKX3.1 mRNA were as follows: 5'-GTACCTGTCGGCCCCTGAACG-3' (sense), and 3'-GGACCAGAGGCACATA TTGTCG-5' (antisense). A 500 bp β-actin mRNA was amplified and used to normalize the quantity of the NKX3.1 mRNA in RT-PCR. The primers for the AR were as follows: 5'-TCTCAAGAG-TTTGGATGGCTCC-3' (sense) and 5'-TCACTGGGTGTGG-AAATAGATG -3' (antisense)[25]. The PCR profiles consisted of initial denaturation at 94 °C for 3 min, followed by 28 cycles of denaturation at 94 °C for 40 s, primer-annealing at 61 °C for 40 s, and primer extension at 72 °C for 40 s. The final primer extension was performed at 72 °C for 10 min. The PCR products were analyzed by electrophoresis on a 1.5% agarose gel containing ethidium bromide and photographed under UV light.
SDS-PAGE and Western blot analysis The LNCaP cells were harvested at designated times, and nuclear extraction was prepared using the protocol described by a nuclear extraction kit (Active Motif, Carlsbad, CA, USA). Protein concentrations of cell nuclear extracts were quantified by the bicinchoninic acid method. For Western blot analysis, 40 µg of protein was separated on 10% SDS-PAGE and then transferred to the nitrocellulose membrane. After being blocked and washed, the membrane was incubated with human specific anti-NKX3.1 antibody or anti-AR antibody (gifts from Dr Charles YOUNG, Department of Urology Research, Mayo Clinic, Rochester, MN, USA) at 4 °C for 12 h, followed by incubation with horseradish peroxidase-labeled second antibody for 1 h at room temperature. Immunoreactive bands were then visualized by enhanced chemiluminescence (Santa Cruz, San Diego, CA, USA). β-tubulin or β-actin (Sigma, St. Louis, MO, USA) was used to normalize the quantity of the protein on the blot.
Electrophoretic mobility shift assay (EMSA) Nuclear extracts were prepared from the LNCaP cells using a nuclear extraction kit (Active Motif, Carlsbad, CA, USA) according to the manufacturer’s instructions. Equal amounts of sense and antisense oligonucleotides were mixed and annealed in a buffer (10 mmol/L Tris-HCl, pH 8.0, 200 mmol/L NaCl, and 1 mmol/L EDTA) by heating to 95 °C for 5 min and slowly cooling to room temperature. The corresponding oligonucleotides were labeled with digoxigenin (DIG). The following oligonucleotides were used for the EMSA experiments: the ARE decoy based on the deduced ARE sequence at the promoter region of the human prostate-specific antigen (PSA) gene[26], and the E2F decoy which contained the 8 bp cis-element (underlined) that was identified in the c-myc promoter[27].
ARE: sense 5'-TGCAGAACAGCAAGTGCTAGC-3',
antisense 5'-GCTAGCACTTGCTGTTCTGCA- 3';
NF-κB: sense 5'-GCCTGGGAAAGTCCCCTCAACT-3',
antisense 5'-AGTTGAGGGGACTTTCCCAGGC-3';
E2F: sense 5'-TGATTTCCCGCGGAT-3',
antisense 5'-ATCCGCGGGAAATCA-3'.
Binding reactions were carried out at room temperature for 30 min in a mixture containing 4% glycerol, 1 mmol/L MgCl2, 0.2 mmol/L EDTA, 0.5 mmol/L dithiothreitol, 50 mmol/L NaCl, 10 mmol/L Tris·HCl, 2 µg poly (dI-dC), 10 µg nuclear extracts, and a DIG-labeled oligonucleotide probe. Then the reaction mixtures were subjected to electrophoresis in 5% non-denaturing polyacrylamide gels in 0.25×Tris/borate/EDTA buffer. Based on the instructions of the DIG Gel Shift Kit (Roche Co, Basel, Switzerland), electroblotting and chemiluminescent detection were performed. The specificity of AR-ARE binding was confirmed by adding 125 fold excess of unlabeled ARE\E2F\NF-κB probe to the assay.
Transient transfection assay The LNCaP cells were plated in a 24 well plate until they reached a confluency of 90%. The cells were transiently transfected with a 1040 bp NKX3.1 promoter-luciferase reporter plasmids pGL3-NKX3.1[28] using LipofectamineTM 2000 reagent (Invitrogen, Carlsbad, CA, USA), while pRL-TK (Promega, Madison, WI, USA) plasmids were used as a internal control. Twenty-four hours after the transfection, the cells were treated with different concentrations of curcumin for an additional 12 h. Whole cell extracts were prepared and a luciferase assay was performed according to the manufacturer’s instructions (Pro-mega, USA) for the NKX3.1 promoter/luciferase transfection. Each transfection was done 3 times and standard deviations were calculated.
Statistical analysis All the measurement data were analyzed and expressed as the mean±SEM. Results were considered significant if P<0.05 was obtained by an appropriate ANOVA procedure and Student’s t-test.
Results
Curcumin inhibited the expression of the NKX3.1 gene To determine whether NKX3.1 expression levels changed with curcumin treatment in the androgen-sensitive prostate cancer cell LNCaP, expression of NKX3.1 was determined in serum-starved LNCaP cells exposed to varying concentrations of curcumin for 24 h by RT-PCR and Western blotting. As shown in Figure 1A, NKX3.1 mRNA expression was significantly downregulated by 40 µmol/L of curcumin treatment (by ~5 fold), and less effect was observed in the cells treated with 10 and 20 µmol/L curcumin.
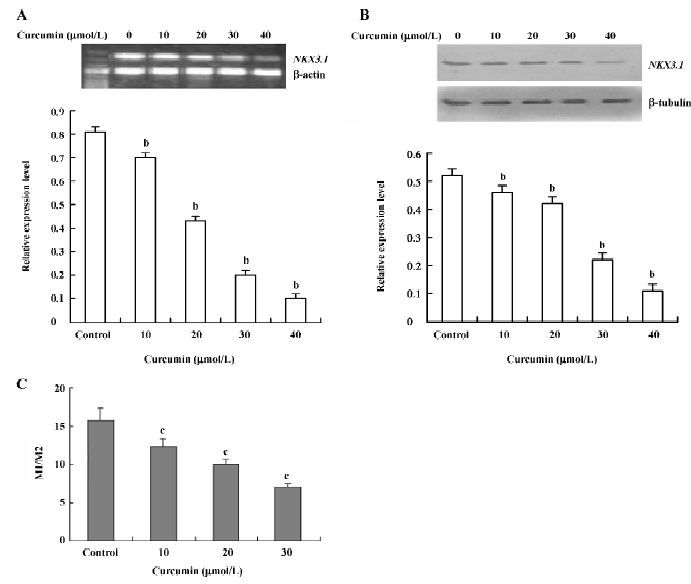
To further confirm the inhibitory effect of curcumin on NKX3.1 protein expression, Western blotting was carried out. Since NKX3.1 is a nuclear protein and functions in the nucleus, we prepared the nuclear extracts for Western blotting after the LNCaP cells were exposed to curcumin for 24 h. The results in Figure 1B show that the expression of the NKX3.1 protein dramatically decreased by curcumin in a dose-dependent manner, similar to its mRNA as shown in Figure 1A.
To test whether the inhibitory effect of curcumin on NKX3.1 expression occurs at the transcription level, we transfected a vector containing a 1040 bp NKX3.1 promoter fragment in the upstream of a luciferase reporter gene into the LNCaP cells. As seen in Figure 1C, for the LNCaP cells exposed to varying amounts of curcumin for 24 h, the NKX3.1 promoter gave a gradual inhibition of the luciferase activity (represent-ing NKX3.1 promoter activity) in a dose-dependent manner.
Taken together, curcumin could inhibit the expression of NKX3.1 at the promoter, mRNA, and protein levels.
Curcumin inhibited androgen-mediated induction of NKX3.1 expression Because NKX3.1 is known as an androgen upregulated gene, we examined the nonmetabolizable androgen R1881 and the AR antagonist flutamide to see if they can influence expression of the NKX3.1 protein in LNCaP cells exposed to curcumin. In Figure 2, we show that the expression of NKX3.1 could be enhanced by R1881 (lane 2), which was partially inhibited by the AR antagonist flutamide (lane 3) without curcumin treatment of LNCaP cells. The results demonstrated that NKX3.1 protein expression was upregulated by androgen and AR activity without curcumin treatment. When the LNCaP cells were exposed to curcumin (30 µmol/L), the NKX3.1 protein had a notable decrease (lane 4), and R1881 no longer stimulated NKX3.1 protein expression significantly (lane 5), which suggests that curcumin may have disrupted the AR function so that R1881 lost its stimulation on NKX3.1 expression. Furthermore, curcumin and flutamide together could further decrease the NKX3.1 protein (lane 6). Our data suggests that the expression of NKX3.1 was partially dependent on the AR signaling pathway, and curcumin could repress androgen and AR-mediated induction of NKX3.1 expression.
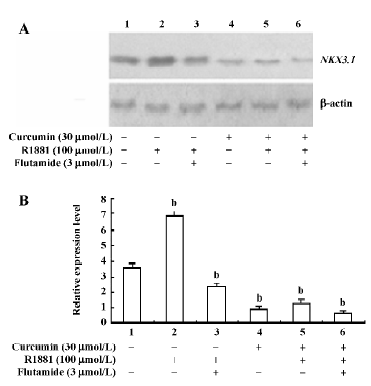
Curcumin inhibited expression of the AR gene To further elucidate the role of AR in curcumin-induced NKX3.1 depression, we evaluated the effect of curcumin on AR expression in LNCaP cells directly. Both RT-PCR and Western blot analysis gave very similar results (Figure 3), indicating that AR expression significantly decreased in mRNA (Figure 3A) and protein (Figure 3B) levels by curcumin in a dose-dependent manner. Remarkably, the effect of curcumin on AR expression was almost identical to that on NKX3.1 expression, as shown in Figure 1.
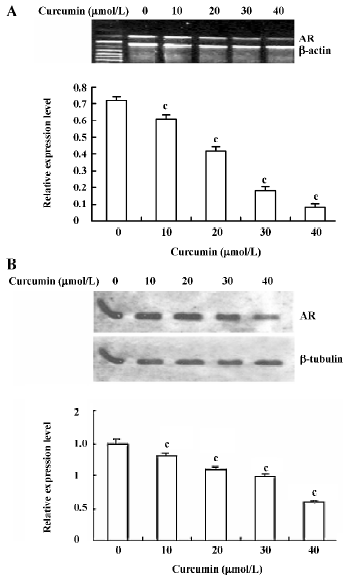
Curcumin decreased ARE binding activity The above results led us to further investigate whether the function of the AR could be affected by curcumin. We used the gel band-shift technique as an in vitro functional assay to determine the AR DNA binding activity. The results in Figure 4 show that ARE binding activity was dramatically inhibited after 24 h of treatment with 30 and 40 µmol/L of curcumin when compared to the control. The bands were confirmed to be a result of specific binding for ARE, because the DNA-protein complex was competed out by a 125 fold molar excess of unlabeled ARE and could not be blocked by a 125 fold excess of unlabeled E2F and NF-κB oligonucleotides.
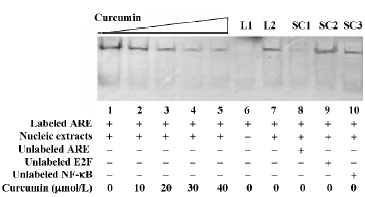
Discussion
Curcumin, used as a food additive and a herbal medicine in Asia, is associated with a plethora of beneficial effects on human health, predominant among which are the anti-inflammatory and cancer chemoprophylaxis activities. Early works have shown that curcumin could suppress the activation of novel eukaryotic transcriptional factors, such as AP-1, NF-κB and AR in prostate cancer[29,30], and significantly inhibit the growth of prostate cancer cells in vivo[19] and in vitro[18]. The AR is a member of the nuclear receptor superfamily of transcription factors[31]. It is activated by its androgen ligand or by a ligand-independent manner[32–34]. Subsequently, the activated receptor homodimerizes and interacts with specific androgen response elements in the regulatory regions of androgen target genes, resulting in the stimulation of gene expression. NKX3.1 is an androgen-regulated homeobox gene in the prostate[22]. In the present study, we first demonstrated the inhibitory effects of curcumin on NKX3.1 expression in LNCaP cells and investigated the mechanisms.
In this study, we used 10–40 µmol/L of curcumin to treat the prostate cancer cell LNCaP that expresses both NKX3.1 and the AR. With RT-PCR, Western blotting, and luciferase reporter analysis, we found that curcumin downregulated the expression of the NKX3.1 gene at both mRNA and protein levels in a dose-dependent manner. To further investigate whether or not this inhibitory effect is androgen-AR mediated, the LNCaP cells were treated with curcumin only or curcumin and synthetic androgen analog R1881. The results in Figure 2 show that curcumin could decrease the NKX3.1 protein and inhibit the stimulation of NKX3.1 expression by androgen, suggesting that curcumin could repress the androgen-mediated induction of NKX3.1 expression. Furthermore, the effects of curcumin on AR expression and its DNA binding activity were detected by Western blotting and EMSA to demonstrate the mechanisms in which curcumin inhibits androgen-mediated induction of NKX3.1 expression. The results show that curcumin decreased AR expression significantly at both mRNA and protein levels, and blocked AR-ARE binding activity in EMSA. Our data suggests that curcumin can repress the androgen-mediated induction of NKX3.1 expression by inhibiting AR gene expression and blocking its DNA binding activity.
In the EMSA experiment, we used the known ARE sequence in the upstream of the PSA gene to show that curcumin could block AR DNA binding activity and make AR lose its function, disrupting the androgen-AR signaling pathway. NKX3.1 is proved to be a prostate-specific and androgen-regulated gene, and it was reported that there are several potential AR binding sites in the regulatory region of the NKX3.1 gene[35,36], but so far, there is no identification of functional ARE in NKX3.1 to be reported. Further investigation will be required to detect functional ARE in the regulatory regions of the NKX3.1 gene and to determine if the ARE is the actual function target of curcumin.
The inhibition of NKX3.1 gene expression by curcumin seems to be inconsistent with the classical idea that the antitumor agent increases the expression of the tumor suppressor gene. NKX3.1 is supposed to be a tumor suppressor gene; however, its function is mainly related to the development and differentiation of normal prostate. Evidence of NKX3.1 being involved in the prostate tumor and progression mainly come from animal models[37–41]. The reports on the roles of NKX3.1 in human prostate cancer are pendent, and some reports are inconsistent. Bowen et al showed that loss of NKX3.1 expression in human prostate cancers correlated with tumor progression[27], while Aslant et al reported that the decline of NKX3.1 expression was not correlated with prostate cancer progression and was not associated with advanced stage. Thus, NKX3.1 expression is not a clinically-valuable prognostic factor[42]. However, overexpression of NKX3.1 in prostate cancer also has been reported[43]. So the roles of NKX3.1 on prostate cancer in clinics needs to be further studied.
In summary, our results demonstrate that curcumin, as an anticancer agent, can downregulate the expression of NKX3.1 which is a prostate-specific and androgen-regulated homeobox gene, and can intervene the AR signaling pathway as well as repress the androgen-mediated induction of NKX3.1 expression by inhibiting AR gene expression and blocking its DNA binding activity.
Acknowledgement
The authors are grateful to Dr Charles YOUNG (Depart-ment of Urology Research, Mayo Clinic, Rochester, MN, USA) for providing the anti-NKX3.1 antibody and anti-AR antibody.
References
- Greenlee RT, Murray T, Bolden S, Wingo PA. The prostate: an increasing medical problem. Prostate 1990;16:39-48.
- Hanks GE. Long-term control of prostate cancer with radiation. Urol Clin North Am 1996;23:605-16.
- Garnick MB. Hormonal therapy in the management of prostate cancer: from Higgins to the present. Urology 1997;49:5-15.
- Sommerkamp HW, Khauli RB. Role of dietary modification and vitamins in prostate cancer. J Med Liban 2005;53:103-6.
- Li Y, Che M, Bhagat S, Ellis KL, Kucuk O, Doerge DR, et al. Regulation of gene expression and inhibition of experimental prostate cancer bone metastasis by dietary genistein. Neoplasia 2004;6:354-63.
- Duvoix A, Morceau F, Delhalle S, Schmitz M, Schnekenburger M, Galteau MM, et al. Induction of apoptosis by curcumin: mediation by glutathione S-transferase P1-1 inhibition. Biochem Pharmacol 2003;66:1475-83.
- Shishu, Singla AK, Kaur IP. Inhibitory effect of curcumin and its natural analogues on genotoxicity of heterocyclic amines from cooked food. Indian J Exp Biol 2002;40:1365-72.
- Aggarwal BB, Shishodia S, Takada Y, Banerjee S, Newman RA, Bueso-Ramos CE. Curcumin suppresses the paclitaxel-induced nuclear factor-kappaB pathway in breast cancer cells and inhibits lung metastasis of human breast cancer in nude mice. Clin Cancer Res 2005;11:7490-8.
- Choudhuri T, Pal S, Das T, Sa G. Curcumin selectively induces apoptosis in deregulated cyclin D1-expressed cells at G2 phase of cell cycle in a p53-dependent manner. J Biol Chem 2005;280:20059-68.
- Gautam SC, Xu YX, Janakiraman N, Chapman RA. Resveratrol selectively inhibits leukemia cells: a prospective agent for ex vivo bone marrow purging. Bone Marrow Transplant 2000;25:639-45.
- Okazaki Y, Iqbal M, Okada S. Suppressive effects of dietary curcumin on the increased activity of renal ornithine decarboxylase in mice treated with a renal carcinogen, ferric nitrilotriacetate. Biochim Biophys Acta 2005;40:357-66.
- Gafner S, Lee SK, Cuendet M, Barthelemy S, Vergnes L, Labidalle S, et al. Biologic evaluation of curcumin and structural derivatives in cancer chemoprevention model systems. Phytochemistry 2004;65:2849-59.
- Leu TH, Maa MC. The molecular mechanisms for the antitumo-rigenic effect of curcumin. Curr Med Chem Anticancer Agents 2002;2:357-70.
- Dikshit P, Goswami A, Mishra A, Chatterjee M, Jana NR. Curcumin induces stress response, neurite outgrowth and prevent NF-kappaB activation by inhibiting the proteasome function. Neurotox Res 2006;9:29-37.
- Lee KW, Kim JH, Lee HJ, Surh YJ. Curcumin inhibits phorbol ester-induced up-regulation of cyclooxygenase-2 and matrix metalloproteinase-9 by blocking ERK1/2 phosphorylation and NF-kappaB transcriptional activity in MCF10A human breast epithelial cells. Antioxid Redox Signal 2005;7:1612-20.
- Woo MS, Jung SH, Kim SY, Hyun JW, Ko KH, Kim WK, et al. Curcumin suppresses phorbol ester-induced matrix metallopro-teinase-9 expression by inhibiting the PKC to MAPK signaling pathways in human astroglioma cells. Biochem Biophys Res Commun 2005;335:1017-25.
- Cho JW, Park K, Kweon GR, Jang BC, Baek WK, Suh MH, et al. Curcumin inhibits the expression of COX-2 in UVB-irradiated human keratinocytes (HaCaT) by inhibiting activation of AP-1: p38 MAP kinase and JNK as potential upstream targets. Exp Mol Med 2005;37:186-92.
- Dorai T, Gehani N, Katz A. Therapeutic potential of curcumin in human prostate cancer. II. Curcumin inhibits tyrosine kinase activity of epidermal growth factor receptor and depletes the protein. Mol Urol 2000;4:1-6.
- Dorai T, Cao YC, Dorai B, Buttyan R, Katz AE. Therapeutic potential of curcumin in human prostate cancer. III. Curcumin inhibits proliferation, induces apoptosis, and inhibits angiogenesis of LNCaP prostate cancer cells in vivo. The Prostate 2001;47:293-303.
- Bieberich CJ, Fujita K, He WW, Jay G. Prostate-specific and androgen-dependent expression of a novel homeobox gene. J Biol Chem 1996; 271: 31 779–82.
- Jiang AL, Zhang PJ, Hu XY, Chen WW, Kong F, Liu ZF, et al. Identification of a positive Cis-element upstream of human NKX3.1 gene. Acta Biochim Biophys Sin 2005;37:773-8.
- He WW, Sciavolino PJ, Wing J, Augustus M, Hudson P, Meissner PS, et al. A novel human prostate-specific, androgen-regulated homeobox gene (NKX3.1) that maps to 8p21, a region frequently deleted in prostate cancer. Genomics 1997;43:69-77.
- Jiang AL, Hu XY, Zhang PJ, He ML, Kong F, Liu ZF, et al. Up-regulation of NKX3.1 expression and inhibition of LNCaP cell proliferation induced by an inhibitory element decoy. Acta Biochim Biophys Sin 2005;37:335-40.
- Vocke CD, Pozzatti RO, Bostwick DG, Florence CD, Jennings SB, Strup SE, et al. Analysis of 99 microdissected prostate carcinomas reveals a high frequency of allelic loss on chromosome 8p12-21. Cancer Res 1996;56:2411-6.
- Dong JT. Chromosomal deletions and tumor suppressor genes in prostate cancer. Cancer Metastasis Rev 2001;20:173-93.
- Voeller HJ, Augustus M, Madike V, Bova GS, Carter KC, Gelmann EP. Coding region of NKX3.1, a prostate-specific homeobox gene on 8p21, is not mutated in human prostate cancers. Cancer Res 1997;57:4455-9.
- Bowen C, Bubendorf L, Voeller HJ, Slack R, Willi N, Sauter G, et al. Loss of NKX3.1 expression in human prostate cancers correlates with tumor progression. Cancer Res 2000;60:6111-5.
- Bhatia-Gaur R, Donjacour AA, Sciavolino PJ, Kim M, Desai N, Young P, et al. Roles for Nkx3.1 in prostate development and cancer. Genes Dev 1999;13:966-77.
- Sadar MD, Hussain M, Bruchovsky N. Prostate cancer: molecular biology of early progression to androgen independence. Endocr Relat Cancer 1999;6:487-502.
- Nakamura K, Yasunaga Y, Segawa T, Ko D, Moul JW, Srivastava S, et al. Curcumin down-regulates AR gene expression and activation in prostate cancer cell lines. Int J Oncol 2002;21:825-30.
- O’Malley B. The steroid receptor superfamily: more excitement predicted for the future. Mol Endocrinol 1990;4:363-9.
- Brinkmann AO, Blok LJ, de Ruiter PE, Doesburg P, Steketee K, Berrevoets CA, et al. Mechanisms of androgen receptor activation and function. J Steroid Biochem Mol Biol 1999;69:307-13.
- Grossmann ME, Huang H, Tindall DJ. Androgen receptor signaling in androgen-refractory prostate cancer. J Nat Cancer Ins 2001;93:1687-97.
- Culig Z. Androgen receptor cross-talk with cell signalling pathways. Growth Factors 2004;22:179-84.
- Korkmaz KS, Korkmaz CG, Ragnhildstveit E, Kizildag S, Pretlow TG, Saatcioglu F. Full-length cDNA sequence and genomic organization of human NKX3A – alternative forms and regulation by both androgens and estrogens. Gene 2000;260:25-36.
- Prescott JL, Blok L, Tindall DJ. Isolation and androgen regulation of the human homeobox cDNA, NKX3.1. Prostate 1998;35:71-80.
- Gao H, Ouyang X, Banach-Petrosky WA, Shen MM, Abate-Shen C. Emergence of androgen independence at early stages of prostate cancer progression in nkx3.1; pten mice. Cancer Res 2006;66:7929-33.
- Abdulkadir SA. Mechanisms of prostate tumorigenesis: roles for transcription factors Nkx3.1 and Egr1. Ann N Y Acad Sci 2005;1059:33-40.
- Kim MJ, Bhatia-Gaur R, Banach-Petrosky WA, Desai N, Wang Y, Hayward SW, et al. Nkx3.1 mutant mice recapitulate early stages of prostate carcinogenesis. Cancer Res 2002;62:2999-3004.
- Shen MM, Abate-Shen C. Roles of the Nkx3.1 homeobox gene in prostate organogenesis and carcinogenesis. Dev Dyn 2003;228:767-78.
- Abate-Shen C, Banach-Petrosky WA, Sun X, Economides KD, Desai N, Gregg JP, et al. Nkx3.1; Pten mutant mice develop invasive prostate adenocarcinoma and lymph node metastases. Cancer Res 2003;63:3886-90.
- Aslan G, Irer B, Tuna B, Yorukoglu K, Saatcioglu F, Celebi I. Analysis of NKX3.1 expression in prostate cancer tissues and correlation with clinicopathologic features. Pathol Res Pract 2006;202:93-8.
- Xu LL, Srikantan V, Sesterhenn IA, Augustus M, Dean R, Moul JW, et al. Expression profile of an androgen regulated prostate specific homeobox gene NKX3.1 in primary prostate cancer. J Urol 2000;163:972-9.