Endothelial progenitor cell differentiation using cryopreserved, umbilical cord blood-derived mononuclear cells1
Introduction
The development of vascular tissues may be considered in several different contexts. Vasculogenesis and angiogenesis are 2 major processes responsible for the formation of new blood vessels. Vasculogenesis refers to the in situ formation of blood vessels from endothelial progenitor cells or angioblasts[1]. In contrast, angiogenesis is a process mediated through the sprouting of new capillaries from pre-existing mature endothelial cells (EC). However, acute stress injury of the vascular endothelium, such as hind-limb ischemia or myocardial ischemia, leads to the loss of the antithrombotic properties of the vessel wall and subsequently leads to an increased number of circulating EC[2]. Thus, regeneration of the vascular endothelium is of particular importance in vascular repair. This endothelial reconstruction can occur by migration and proliferation of surrounding mature EC. However, mature EC are terminally differentiated cells with a low proliferative potential, and their capacity to repair damaged endothelium is limited. Therefore, endothelial repair may need the support of other types of cells[2]. Recent evidence show that the peripheral blood (PB) of adults contains a unique subtype of circulating, bone marrow-derived cells[3] with properties similar to those of embryonic angioblasts[4]. These cells have the potential to proliferate and to differentiate into mature EC and play an important role in vascular repair. Therefore, they were termed endo-thelial progenitor cells (EPC).
EPC populations can be grown from mononuclear cells (MNC), purified populations of CD34-positive, or CD133-positive hematopoietic cells[5,6]. In addition, CD14-positive MNC have been used as the starting population for cultivation of EPC[7]. Although there is convincing evidence for the improvement of neovascularization by EPC transplanta-tion, the origin of the endothelial progenitor lineage and its characterization is not clear. EPC have been identified mainly in whole mononuclear cell fractions of PB, leukapheresis products[8,9], bone marrow, and umbilical cord blood (UCB)[10,11]. Although most studies have been conducted with bone marrow or PB-derived EC populations, only little is known about the capacity of UCB-derived EPC. A recent study suggests that UCB MNC-derived EPC have monocytic characteristics and exhibit a unique functional activity to improve neovascularization after hind-limb ischemia[12]. All the groups that studied UCB-derived EPC, as well as group A, used fresh UCB MNC; however, there are practical limitations in the clinical application of EPC that use fresh UCB.
As the autologous or allogeneic hematopoietic stem cell transplantation for the treatment of various diseases has rapidly grown in recent years, the idea of UCB banking for future use has drawn great interest. More than 100 000 units of UCB have been collected, frozen, and stored worldwide in anticipation of their clinical use. Study in the potential of cryopreserved UCB is of great importance for its future clinical use, and we have attempted to enlarge the clinical applications, especially in the angiogenesis field.
In this investigation, we induced the differentiation of EPC from cryopreserved UCB-derived MNC using a simple culture method and characterized them in terms of EPC marker expression. Moreover, those cells were assessed in their EPC potential in comparison with EPC from fresh PB-derived MNC.
Materials and methods
UCB- and PB-derived MNC This project was approved by the institutional review board (IRB) committee of Ajou University, Suwon, Korea with signed consent from each patient. Human UCB samples (60–120 mL) were collected from a fresh umbilical cord attached to the placenta by gravity flow in sterile blood bags containing CPDA-1 as an anticoagulant. Human PB was collected from healthy volunteers. MNC were isolated from the collected blood using the Histopaque-density centrifugation method (Sigma, St Louis, MO, USA). The collected MNC layers from UCB were washed twice with phosphate buffer saline (PBS) and cryopreserved in liquid nitrogen. PB-derived MNC were directly differentiated EPC without the freezing step.
Cell culture The medium used for cell culture experiments was Medium 199 (Life Technologies, Grand Island, NY, USA) supplemented with 10% fetal bovine serum(FBS), bovine pituitary extract as EC growth supplements (3 mg/L; Sigma, USA) and 100 U/mL penicillin, and streptomycin (Life Technologies, USA). 2×106 MNC from cryopreserved UCB or PB were plated in 6-well trays pre-coated with human fibronectin (Sigma, USA) and were incubated in a humidified atmosphere at 37 °C with 5% CO2. The medium was changed on d 3 after plating and every 3 d subsequently. Human umbilical vein EC (HUVEC) were obtained from Dr Jung Keuk PARK’s laboratory, DongGuk University, Seoul, Korea, and were cultured in EC basal medium-2 (EBM-2) (Clonetics, Walkersville, MD, USA) supplemented with EGM-2 SingleQuots (Clonetics, Walkersville, MD, USA). HUVEC in the passages between 3 and 6 were used in this study.
Freezing procedures The freezing of MNC was done as previously described[13]. Separated MNC were put into standard 1.8 mL cryotubes (Nalge Nunc, Rochester, NY, USA) at 4×107–6×107 cells per mL with a final concentration of 10% DMSO (Sigma, USA) in autologous plasma while being maintained at 4 °C. These samples were frozen in a controlled rate freezer (Custom Biogenic System, Shelby Township, MI, USA) and were further stored in liquid nitrogen (-196 °C) until further use. UCB samples that had undergone cryostorage for 0.2–5 years were used for the study.
Flow cytometric analysis Cryopreserved UCB- and PB-derived MNC were induced to differentiate into endothelial cells and were detached using 0.25% Trypsin-EDTA (Life Technologies, USA) on d 7 and 14. They were subjected to flow cytometric analysis in order to examine the surface expression of CD31, CD34, CD105, CD45, CD133, and CD146. The cells were labeled with 1 mg/L fluorescein isothiocyanate (FITC)- or phycoerythrin (PE)-conjugated mouse anti-human CD31-PE (Beckman Coulter, Paris, France), CD34-FITC, CD45-FITC, CD146-PE (BD Sciences, Franklin Lakes, NJ, USA), CD105-PE (Ancell, Bayport, MN, USA), and CD133-PE (Miltenyi Biotec, Bergisch Gladbach, Germany) monoclonal antibodies for 30 min at 4 °C in PBS. FITC- or PE-conjugated mouse IgG were used as the control isotype at the same concentration when the specific primary antibodies were used. The fluorescence intensity of the cells was evaluated by flow cytometry using a flow cytometer (BD Sciences, Franklin Lakes, NJ, USA), and the data were analyzed with CELLQUEST software (BD Sciences, USA).
RT-PCR Total RNA was prepared from cultured cells using the TriZol Reagent (Life Technologies, USA). RNA from HUVEC and MNC (before differentiation) was also obtained. cDNA synthesis was performed with AMV Reverse Transcriptase XL (TaKaRa, Otsu, Japan), according to the manufacturer’s protocol. Two μg of the produced DNA was amplified for 30 cycles using the following cycle condition: 94 °C for 30 s, 56 °C for 1 min, and 72 °C for 1 min. Human primers were: 5' GGTCTTACGGAGTATTGCTG 3' (forward) and 5' CTTTCTTTTGGGTCTCTGTG 3' (reverse) for Flt-1/VEGFR-1; 5' GGACCTGGCGGCACGAAATA 3' (forward) and 5' AGGCCGGCTCTTTCGCTTAC 3' (reverse) for kinase insert domain receptor (KDR)/VEGFR-2; 5' AAGA-CATTTTCGGGCTCACGCTGCGCACCC 3' (forward) and 5' TGGGGTAGGCACTTTAGTAGTTCTCCTAAC 3' (reverse) for ecNOS; 5' GATGCAGAGGCTCATGATGC 3' (forward) and 5' CTTGCGACTCACGCTTGACT 3' (reverse) for VE-cadherin; 5' CACCGTTTGCCCACCCTTCG 3' (forward) and 5' GCCCACTGGGAGCCGACACT 3' (reverse) for von Willebrand factor (vWF)[7]; and 5' TGAACCAGGCTTCAG-CATC 3' (forward) and 5' GGACTTCGAGCAAGATATGG 3' (reverse) for β-actin. The amplified cDNA fragments were electrophoresed through a 1% agarose gel and ethidium bromide stained and then photographed under an ultraviolet transilluminator (Core-Bio, Seoul, Korea).
Immunocytochemistry analysis Cells were cultured onto fibronectin-coated, 2-chamber Lab-Tek slides (Nalge NUNC International, Rochester, NY, USA) for 10 d. The cells were fixed and sequentially incubated with primary antibodies against vWF, KDR (Santa Cruz Biotechnology, Santa Cruz, CA, USA), and VE-cadherin (Chemicon, Billerica, MA, USA), and appropriated secondary antibodies. To detect 1,1'-dioctadecyl-3,3,3'-tet-ramethylidocarbocyanine-labeled acetylated LDL (Dil-Ac-LDL) (Biomedical Technologies, Stoughton, MA, USA) uptake, cells were incubated with 10 µg/mL Dil-Ac-LDL in fresh medium for 4 h and were fixed in 2% paraformaldehyde. The cells were stained with FITC-labeled lectin from Ulex europaeus (UEA-1, Sigma, USA). After being washed with PBS, the cells were stained with 1.5 µg/mL of 4',6-diamidino-2-phenylindole dihydrochloride (DAPI, Sigma, USA). The slides were examined and photographed under a fluorescent microscope (Olympus, Tokyo, Japan).
Measurement of VEGF concentration To assess VEGF secretion, cells were switched to growth factor-free basal medium M199 with 10% FBS on d 10 for 72 h and then the supernatant was harvested. VEGF concentration was measured using an ELISA kit (R&D Systems, Minneapolis, MN, USA).
EPC proliferation assay EPC proliferation after differentiation was determined by MTT assay. After being differentiated for 7 d, EPC were detached and cultured in M199 medium with 1% FBS and 30 μg/mL endothelial cell growth supplement (ECGS) in a 96-well plate for 3–4 d. Then FBS was added (to make a series of final concentrations: 10% and 20%) to investigate FBS effects on proliferation. The medium with 1% FBS and 30 µg/mL ECGS served as the control. After being cultured for 24 h, EPC were supplemented with 1 g/L MTT and were incubated for another 2 h. Then the supernatant was discarded by aspiration, and the EPC preparation was shaken with 100 µL DMSO for 15 min. The optical density was measured at 570 nm with the Thermo Max (Molecular Devices, Sunnyvale, CA, USA).
Results
Morphological and immunophenotypical analysis of differentiated EPC The viability of thawed UCB-derived MNC was greater than 90% by trypan blue exclusion assay. When cryopreserved UCB- and PB-derived MNC were cultured on fibronectin-coated wells, a number of round-shaped cells were loosely attached to the bottom after 24 h (Figure 1A, 1D), and numerous spindle-shaped cells began to appear from the round-shaped ones on d 7 (Figure 1B, 1E). Cord-like structures were observed on d 8 (Figure 1C, 1F), and such structures were maintained until d 14.
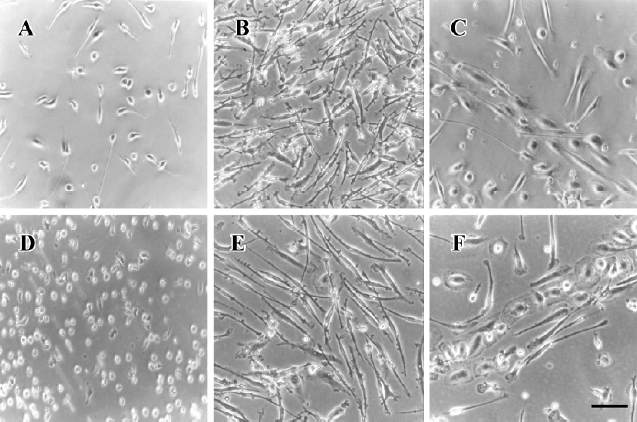
Immunophenotypical analysis was performed on the cells on d 0 (before differentiation), 7, and 14. We analyzed the expression level of CD34 as an endothelial and hematopoietic progenitor marker[13–15]; CD31, CD105, and CD146 as markers for endothelial lineage; CD14 as a monocyte/macrophage antigen; and CD45 as a common leukocyte antigen. HUVEC were included as a positive control. Expression of CD31 and CD105 on UCB-derived MNC was high, which we reported recently[16], and increased after differentiation (Figure 2). Perhaps UCB-derived MNC had the gene with the potential to differentiate into EC. CD34 and CD146 did not present on d 0, but they increased after differentiation. Whereas HUVEC were fully differentiated cells, UCB-derived MNC might need further development, leading to a rather weak expression of CD146. Although CD146 expression increased during the culture, it did not reach the expression levels seen in HUVEC. EPC could still be at an early or incomplete stage of differentiation. The expression level of CD45 was strong before differentiation and did not decline during the culture. We found that both cryopreserved UCB-derived EPC and PB-derived EPC showed expression of endothelial markers after differentiation. Additionally, their expressed patterns did not reveal any striking differences.
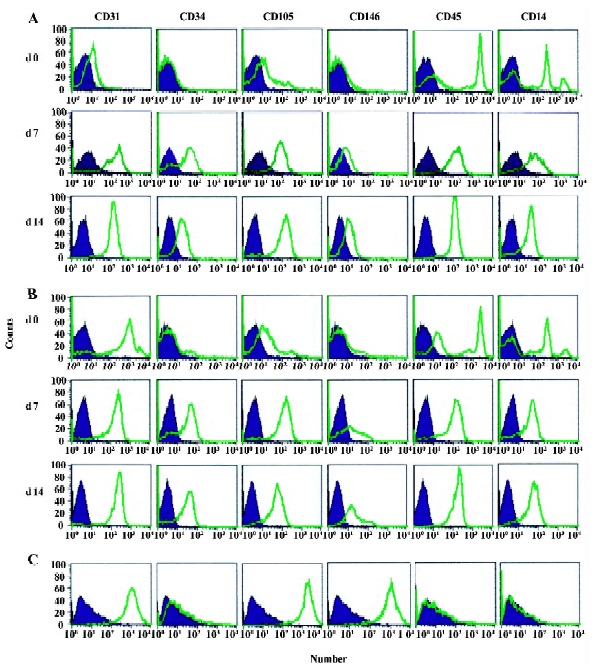
RT-PCR analysis RT-PCR analysis was carried out for Flt-1, KDR, ecNOS, VE-cadherin, and vWF. After a 7 d culture in the differentiation medium, all the EC markers were expressed in cryopreserved UCB-derived EPC (Figure 3). One of the important functions of endothelial cells is to produce nitric oxide (NO)[17], and this production is mediated by NO syntheses (NOS). ecNOS expression in those cells suggests that differentiated EPC (in this study, UCB-derived EPC), are functional in terms of NO production. Additionally, Flt-1 was identified in UCB MNC before the previously-described differentiation[7] and remained during the differentiation process. Subsequently, PB-derived MNC did not express any EC markers at all, and PB-derived EPC also showed the same EC marker expression profiles as the UCB-derived EPC.
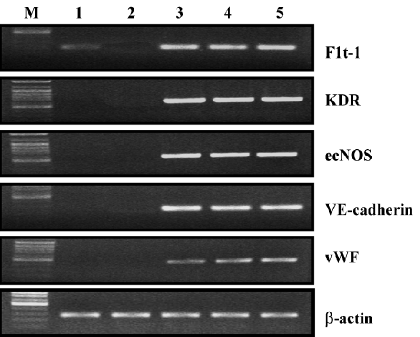
Immunocytochemistry of EPC Immunocytochemistry analysis was performed to further characterize the differentiated EPC. UCB-derived EPC were stained positive for KDR, VE-cadherin, vWF, and UEA-1 on d 10 (Figure 4). In addition, more than 90% of the spindle-shaped cells on d 7 took up DiI-ac-LDL (Figure 4), one of the characteristics of endothelial cells.
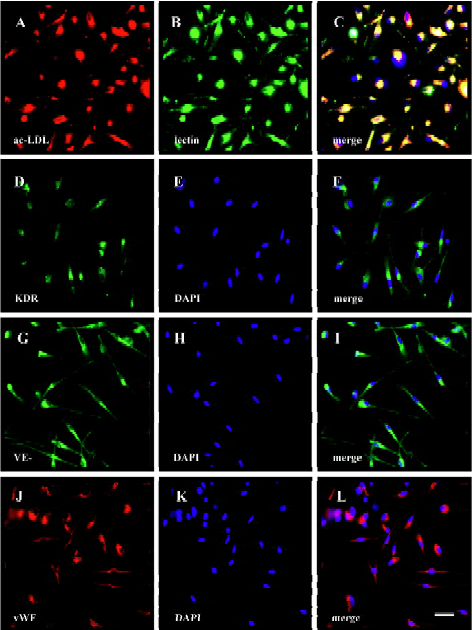
Secretion of VEGF by EPC The concentration of VEGF, one of the well-known angiogenic cytokines, was measured in the medium in which EPC were cultured. Both EPC from UCB and PB secreted a comparable amount of VEGF (Figure 5) in the reports from the YB Park group[18]. The basal medium M199 with 10% FBS did not contain measurable amounts of VEGF.
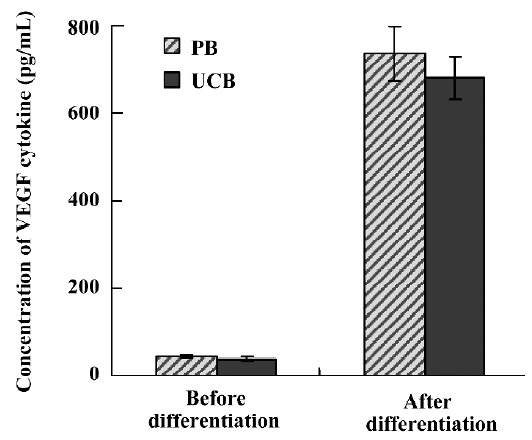
EPC proliferation after differentiation To study the proliferation potentiality of EPC, we performed a MTT assay and compared the result from the UCB-derived EPC with that from PB-derived EPC. The results were expressed as the mean±SD of the relative value of control cells, which were in the medium with 1% FBS+ECGS. Differentiated EPC from both UCB and PB were positively proliferated as compared with the controls (Figure 6).
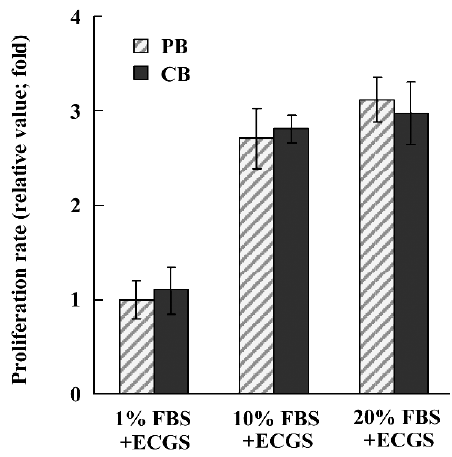
Discussion
Various kinds of clinical trials are taking place using numerous types of stem cell sources such as bone marrow, peripheral blood and cord blood. More than 100 000 units of cord blood that has been collected, frozen, and stored worldwide in anticipation of their clinical use, are of great importance for future clinics. However, there are many limitations to using fresh cord blood for a clinical treatment of cord blood. Many documents have already reported EPC differentiation from fresh UCB[19–21]. When a growth factor cocktail of high density such as VEGF, IGF, EGF, and FGF –– which is used for EPC differentiation using fresh UCB –– is used for EPC differentiation using cryopreserved UCB, there is a lower yield compared to EPC differentiation using fresh UCB. Recently 20% FBS supplemented with crude bovine pituitary extract as an EPC growth supplement was used[11]. Therefore, we used both 20% and 10% FBS with crude bovine pituitary extract as an EPC growth supplement and both outcomes were similarly good, as seen in Figure 6. Thus, we used 10% FBS supplemented with crude bovine pituitary extract as an EPC growth supplement.
Depending on the time-dependent appearance, EPC can be categorized as early EPC and late EPC, and they show different morphology, proliferation rate, survival features, and functions[22]. Although early EPC may be poor in vitro functions, they may be good supporters for endothelial cells forming new vessels[18]. These early EPC-like cells secreted angiogenic cytokines such as VEGF, hepatocyte growth factor (HGF), interleukin-8, and granulocyte-colony stimulating factor (G-CSF), which might result in improved angiogenesis. Therefore, we checked VEGF concentration as angiogenic cytokines from cryopreserved UCB-derived EPC and were able to get results. These cytokines might activate adjacent endothelial cells and enhance angiogenesis. Additionally, to assess the differentiation potential, we differentiated through the method mentioned above, from PB-derived MNC and fresh UCB-derived MNC. Cryopreserved UCB-, fresh UCB-, and PB-derived EPC expressed all the EC markers, and stained positive for KDR, VE-cadherin, vWF, and UEA-1 (data not shown). The results of proliferation and secretion of VEGF were also similar. Moreover, we suggested that cryopreserved UCB-derived EPC have identical differentiation potential with the fresh UCB- and PB-derived EPC.
In this report, we investigated the in vitro differentiation of EPC using cryopreserved UCB-derived MNC in order to establish a culture system for EPC. To confirm the EPC characteristics, we used the previously-reported EC markers. Cell surface molecules such as CD34, KDR, Tie-2/Tek, CD146, and VE-cadherin are known to be expressed by EC at the early stage of vasculogenesis[23–25]. Flt-1, one of the receptors for VEGF, is a marker expressed on primitive angioblasts during vasculogenesis in embryogenesis, as well as on EC during tumor angiogenesis in adults[25]. vWF, expressed on mature endothelium and platelets, has been well utilized to identify EC[23]. Despite the large number of molecules reported in vasculogenesis/angiogenesis, there has been no exclusive marker for EPC yet[26]. We observed that cryopreserved, UCB-derived EPC expressed all the makers of endothelial cells using the RT-PCR analysis. In a flow cytometric analysis, these cells were strongly positive for CD31 and CD105, and expressed CD146 that is found on circulating EPC[22], but at a low level. Although CD146 expression increased during the culture, it did not reach the expression levels seen in HUVEC. The CD34 that was present on endothelial progenitors and hematopoietic progeni-tors[6,13,15] was not present on undifferentiated MNC, but was expressed after differentiation. Most mature endothelial cells, except for microvascular endothelial cells, no longer express CD34[27]. The EPC that were differentiated with the culture method that we have established could still be at an early or incomplete stage of differentiation. The surface marker expression that was reported by the Rehman group[28] and their angiogenic effects are most likely mediated by growth factor secretion.
It was reported that expression of CD14 and CD45 on undifferentiated UCB MNC decreases over time, but remains strong after differentiation until 28 d. In the case of CD34+ culture-dish-non-adherent cells, expression of CD45 and CD14 decreased, while CD34 and the progenitor cell marker CD133 remained strong. In CD34+ culture-dish-adherent cells, however, CD14 decreased, and CD45 did not change[19]. From our results, CD45 and CD14 remained strong until d 14 as seen in Figure 2 and gradually decreased afterwards until d 28 after differentiation, which was similar to the result of unselected MNC.
We were able to produce EPC through the method mentioned above, which is a simpler culture method for a short period of time. It has been widely reported through in vivo tests that those cultured EC can be used for clinical trials of ischemic diseases[14,15]. However, the amount of cells that can be obtained through the culture is not sufficient for a clinical application. The previous method[10] uses fresh bone marrow[27], peripheral blood[18], and cord blood[20], while we successfully cultured the cells kept at -196 °C, frozen from UCB-derived MNC, into cells with the quality of EPC. We also obtained the same results when we used stored MNC in -196 °C for a long period of time. The clinical trials using cord blood will significantly increase in the future due to the clinical importance of cord blood. Our method enhances the utility of cord blood banking for a differentiation of endothelial cells as well as a clinical application. Our results also suggest that cryopreserved UCB can be used as a potential source of endothelial cells for cellular angiogenic therapies.
References
- Rosenzweig A. Endothelial progenitor cells. N Engl J Med 2003;348:581-2.
- Hristov M, Erl W, Weber PC. Endothelial progenitor cells: mobilization, differentiation, and homing. Arterioscler Thromb Vasc Biol 2003;23:1185-9.
- Harraz M, Jiao C, Hanlon HD, Hartley RS, Schatteman GC. CD34- blood-derived human endothelial cell progenitors. Stem Cells 2001;19:304-12.
- Kamihata H, Matsubara H, Nishiue T, Fujiyama S, Tsutsumi Y, Ozono R, et al. Implantation of bone marrow mononuclear cells into ischemic myocardium enhances collateral perfusion and regional function via side supply of angioblasts, angiogenic ligands, and cytokines. Circulation 2001;104:1046-52.
- Arras M, Ito WD, Scholz D, Winkler B, Schaper J, Schaper W. Monocyte activation in angiogenesis and collateral growth in the rabbit hindlimb. J Clin Invest 1998;101:40-50.
- Asahara T, Murohara T, Sullivan A, Silver M, van der Zee R, Li T, et al. Isolation of putative progenitor endothelial cells for angiogenesis. Science 1997;275:964-7.
- Fernandez Pujol B, Lucibello FC, Gehling UM, Lindemann K, Weidner N, Zuzarte ML, et al. Endothelial-like cells derived from human CD14 positive monocytes. Differentiation 2000;65:287-300.
- Gehling UM, Ergun S, Schumacher U, Wagener C, Pantel K, Otte M, et al. In vitro differentiation of endothelial cells from AC133-positive progenitor cells. Blood 2000;95:3106-12.
- Gill M, Dias S, Hattori K, Rivera ML, Hicklin D, Witte L, et al. Vascular trauma induces rapid but transient mobilization of VEGFR2(+)AC133(+) endothelial precursor cells. Circ Res 2001;88:167-74.
- Murohara T. Therapeutic vasculogenesis using human cord blood-derived endothelial progenitors. Trends Cardiovasc Med 2001;11:303-7.
- Murohara T, Ikeda H, Duan J, Shintani S, Sasaki K, Eguchi H, et al. Transplanted cord blood-derived endothelial precursor cells augment postnatal neovascularization. J Clin Invest 2000;105:1527-36.
- Urbich C, Heeschen C, Aicher A, Dernbach E, Zeiher AM, Dimmeler S. Relevance of monocytic features for neovascularization capacity of circulating endothelial progenitor cells. Circulation 2003;108:2511-6.
- Peichev M, Naiyer AJ, Pereira D, Zhu Z, Lane WJ, Williams M, et al. Expression of VEGFR-2 and AC133 by circulating human CD34(+) cells identifies a population of functional endothelial precursors. Blood 2000;95:952-8.
- Pesce M, Orlandi A, Iachininoto MG, Straino S, Torella AR, Rizzuti V, et al. Myoendothelial differentiation of human umbilical cord blood-derived stem cells in ischemic limb tissues. Circ Res 2003;93:e51-62.
- Rafii S, Shapiro F, Rimarachin J, Nachman RL, Ferris B, Weksler B, et al. Isolation and characterization of human bone marrow microvascular endothelial cells: hematopoietic progenitor cell adhesion. Blood 1994;84:10-9.
- Lee MW, Choi J, Yang MS, Moon YJ, Park JS, Kim HC, et al. Mesenchymal stem cells from cryopreserved human umbilical cord blood. Biochem Biophys Res Commun 2004;320:273-8.
- Bompais H, Chagraoui J, Canron X, Crisan M, Liu XH, Anjo A, et al. Human endothelial cells derived from circulating progenitors display specific functional properties compared with mature vessel wall endothelial cells. Blood 2004;103:2577-84.
- Hur J, Yoon CH, Kim HS, Choi JH, Kang HJ, Hwang KK, et al. Characterization of two types of endothelial progenitor cells and their different contributions to neovasculogenesis. Arterioscler Thromb Vasc Biol 2004;24:288-93.
- Eggermann J, Kliche S, Jarmy G, Hoffmann K, Mayr-Beyrle U, Debatin KM, et al. Endothelial progenitor cell culture and differentiation in vitro: a methodological comparison using human umbilical cord blood. Cardiovasc Res 2003;58:478-86.
- Fan CL, Li Y, Gao PJ, Liu JJ, Zhang XJ, Zhu DL. Differentiation of endothelial progenitor cells from human umbilical cord blood CD 34+ cells in vitro. Acta Pharmacol Sin 2003;24:212-8.
- Kim SY, Park SY, Kim JM, Kim JW, Kim MY, Yang JH, et al. Differentiation of endothelial cells from human umbilical cord blood AC133-CD14+ cells. Ann Hematol 2005;84:417-22.
- Lin Y, Weisdorf DJ, Solovey A, Hebbel RP. Origins of circulating endothelial cells and endothelial outgrowth from blood. J Clin Invest 2000;105:71-7.
- Eichmann A, Corbel C, Nataf V, Vaigot P, Breant C, Le Douarin NM. Ligand-dependent development of the endothelial and hemopoietic lineages from embryonic mesodermal cells expressing vascular endothelial growth factor receptor 2. Proc Natl Acad Sci USA 1997;94:5141-6.
- Nishikawa SI, Nishikawa S, Hirashima M, Matsuyoshi N, Kodama H. Progressive lineage analysis by cell sorting and culture identifies FLK1+VE-cadherin+ cells at a diverging point of endothelial and hemopoietic lineages. Development 1998;125:1747-57.
- Yamaguchi TP, Dumont DJ, Conlon RA, Breitman ML, Rossant J. flk-1, an flt-related receptor tyrosine kinase is an early marker for endothelial cell precursors. Development 1993;118:489-98.
- Rafii S. Circulating endothelial precursors: mystery, reality, and promise. J Clin Invest 2000;105:17-9.
- Reyes M, Dudek A, Jahagirdar B, Koodie L, Marker PH, Verfaillie CM. Origin of endothelial progenitors in human postnatal bone marrow. J Clin Invest 2002;109:337-46.
- Rehman J, Li J, Orschell CM, March KL. Peripheral blood “endothelial progenitor cells” are derived from monocyte/macrophages and secrete angiogenic growth factors. Circulation 2003;107:1164-9.