Effect of genistein on mouse blastocyst development in vitro
Introduction
Apoptosis, which is widely observed in different cells of various organisms, is a unique morphological pattern of cell death characterized by chromatin condensation, membrane blebbing, and DNA fragmentation[1]. Apoptosis plays an important role in the homeostasis of multicellular organisms. Moreover, abnormal apoptotic function has been associated with multiple human diseases, including neurodegenerative disorders and cancers[2]. During normal embryogenesis, apoptosis functions to remove abnormal or redundant cells from preimplantation embryos[3,4]; during normal mouse embryonic development, this occurs only after the blastocyst stage[5]. Premature apoptosis, such as that triggered by exposure to teratogens, causes improper embryonic development[6,7]. We recently reported that several natural phytochemical compounds, including curcumin and ginkgolides, were able to prevent or induce apoptosis in embryonic stem cells and/or embryos[8–10]. The effects of genistein on human embryo development are unclear. In the present study, we investigated whether another natural compound, genistein, had cytotoxic effects on embryonic development. Our study will provide important new insights about the effect of dietary genistein on embryogenesis. The effect of genistein on embryo development in vitro must be studied because it is especially in relation to the dietary use of iso-flavones (such as genistein) in pregnant women.
Genistein, a natural isoflavone compound found in soy products, has been shown to inhibit protein tyrosine kinase and topoisomerase-II activity[11,12], leading to numerous effects on diverse cell functions. For example, genistein has been shown to inhibit tumor cell proliferation and induce tumor cell differentiation[13–16], and may also trigger cell cycle arrest and apoptosis in some cell types[17–19], while blocking apoptosis under other circumstances[20–22]. Recent studies have shown that genistein can cause cell cycle arrest and developmental injury in embryos via its function as a tyro-sine kinase inhibitor[23–25]. It is thought that genistein inhibits epidermal growth factor (EGF) receptor tyrosine kinase activity, inhibiting the positive effect of EGF on glucose uptake. Since glucose is the main exogenous energy source for the early development of the preimplantation embryo, the genistein-induced inhibition of glucose uptake would be expected to dramatically affect early embryonic develop-ment[23]. Genistein has also been suggested to inhibit tyrosine phosphorylation of the cadherin-catenin complex, which mediates compaction, adhesive functions, and embryonic cleavage from the 2 to 4 cell stages in the mouse embryo[26]. In addition, mitochondria were observed to form numerous tiny clusters uniformly distributed in the cytoplasm in genistein-treated embryos, causing G2-stage arrest in the 2 cell stage of the embryo, suggesting that genistein may inhibit embryonic development by altering mitochondrial structure and function[24]. However, although multiple studies have addressed the inhibitory effects of genistein on embryonic development due to the inhibition of tyrosine kinases or mitochondria-based mechanisms, no previous work has examined the apoptotic effects of genistein on the early stages of mammalian embryonic development, especially during the blastocyst stage.
In the present study, we exposed mouse blastocysts to genistein and examined apoptosis in the inner cell mass (ICM) and trophectoderm (TE), and also observed early postim-plantation embryonic development on culture dishes in vitro. Finally, the in vivo effects of dietary genistein on cell apopto-sis and proliferation were investigated in an animal model of embryogenesis.
Materials and methods
Chemicals and reagents Pregnant mares’ serum gonadotropin (PMSG), sodium pyruvate, genistein, and daidzein were purchased from Sigma (St Louis, MO, USA). Human chorionic gonadotropin (hCG) was obtained from Serono (NV Organon Oss, the Netherlands). The Annexin-V-FLUOS staining kit and the terminal deoxynucleotidyl transferase-mediated dUTP nick-end labeling (TUNEL) in situ cell death detection kit were obtained from Roche Molecular Biochemi-cals (Mannheim, Germany) and the CMRL-1066 medium was from Gibco Life Technologies (Grand Island, NY, USA).
Animals and collection of embryos ICR (a strain of mice) virgin albino mice (6–8 weeks old) were induced to superovulate by injection of 5 IU PMSG followed 48 h later by an injection of 5 IU hCG. The mice were then mated overnight with a single fertile male of the same strain. Female mice with vaginal plugs were separated and used for the experiments. All mice were maintained on breeder chow and kept under a 12 h day/12 h night regimen, with food and water available ad libitum. All animals received humane animal care, as outlined in the Guidelines for Care and Use of Experimental Animals (Canadian Council on Animal Care, Ottawa, Canada, 1984). The day a vaginal plug was found was defined as d 0 of pregnancy. Morulas were obtained by flushing the fallopian tubes on the afternoon of d 3, and the blastocysts were obtained by flushing the uterine horn on d 4; in both cases the flushing solution consisted of CMRL-1066 culture medium containing 1 mmol/L glutamine and 1 mmol/L sodium pyruvate.
Genistein treatment The embryos were collected in uncoated plastic 35 mm culture dishes and washed a minimum of 3 times. The expanded blastocysts from different females were pooled and randomly selected for the experiments. The blastocysts were incubated at 37 oC for 24 h in CMRL-1066 medium with and without the indicated concentrations of genistein or daidzein, and were then used for further experiments as described below.
Blastocyst cell counting The blastocysts were incubated with culture medium containing 0–50 µmol/L genistein. Twenty-four hours later, the blastocysts were washed with genistein-free medium, and dual differential staining[27] was used to facilitate counting of cell numbers in the ICM and TE. The blastocysts were incubated in 0.4% pronase in M2 medium containing 0.1% bovine serum albumin (M2-BSA medium) for the removal of the zona pellucida. The denuded blastocysts were exposed to 1 mmol/L trinitrobenzenesul-phonic acid in BSA-free M2 medium containing 0.1% polyvinylpyrrolidone (PVP) at 4 oC for 30 min, and then washed with M2 medium[28]. The blastocysts were further treated with 30 µg/mL anti-dinitrophenol-BSA complex antibody in M2-BSA at 37 oC for 30 min, and then with M2 medium supplemented with 10% whole guinea pig serum as a source of complement, 20 μg/mL bisbenzimide, and 10 µg/mL propidium iodide (PI) at 37 oC for 30 min. The immunolysed blastocysts were gently transferred to slides and protected from light before observation. Under UV light excitation, the ICM cells (which take up bisbenzimide, but exclude PI) appeared blue, whereas the TE cells (which take up both fluorochromes) appeared orange-red. As multinucleated cells are not common in preimplantation embryos[29], the number of nuclei was considered to represent an accurate measure of the cell number.
Detection of cell apoptosis For TUNEL staining, the embryos were washed in genistein-free medium, fixed, permeabilized, and subjected to TUNEL labeling using an in situ cell death detection kit (Roche, Germany) according to the manufacturer’s protocol. Photographic images were taken using a fluorescence microscope under bright light (Olympus BX 51, Tokyo, Japan). For Annexin-V staining, the blastocysts were incubated in 0–50 µmol/L genistein for 24 h, washed with genistein-free culture medium, and then stained with an Annexin-V-FLUOS staining kit (Roche, Germany), according to the manufacturer’s instructions. Briefly, the blastocysts were incubated in M2-BSA for the removal of the zona pellucida, washed well with phosphate buffer saline (PBS) plus 0.3% BSA, and then incubated for 60 min with a mixture of 100 µL binding buffer, 1 µL fluorescein isothiocyanate (FITC)-conjugated Annexin-V and 1 µL PI. After incubation, the embryos were washed and photographed under a fluorescence microscope. Cells staining Annexin-V+/PI– were considered apoptotic, while those staining Annexin-V+/PI+ were considered necrotic.
Observation of in vitro implantation and postimplantation development The blastocysts were cultured according to a modification of the previously reported method[30]. Briefly, the embryos were cultured in 4 well multidishes at 37 °C. For group culture, 3 embryos were cultured per well. The basic medium consisted of CMRL-1066 supplemented with 1 mmol/L glutamine and 1 mmol/L sodium pyruvate, plus 50 IU/mL penicillin and 50 mg/mL streptomycin (hereinafter called culture medium). For the treatments, the embryos were cultured with the indicated doses of genistein for 24 h without serum supplementation. Thereafter, the embryos were cultured for 3 d in culture medium supplemented with 20% fetal calf serum, and for 4 d in culture medium supplemented with 20% heated-inactivated human placental cord serum, for a total culture time of 8 d from the onset of treatment. The embryos were inspected daily under a phase contrast microscope (Olympus IMT-2, Tokyo, Japan), and the developmental stages were classified according to established methods[31]. Developmental parameters, such as hatching through the zona pellucida, attachment to the culture dish, trophoblastic outgrowth, and differentiation of the embryo proper were recorded daily. To decrease observer bias, all data were analyzed using the following previously published criteria[32]: an implanted blastocyst was defined by attachment to the culture dish, followed by outgrowth; an early egg cylinder (EEC) was defined as an embryo that had reached stage 9 or 10 by d 4; a late egg cylinder (LEC) was defined as an embryo that had reached stage 11, 12, or 13 by d 6 of culture; and an early somite (ES) was defined as an embryo that had reached stage 14 or 15 by d 8[32].
Effect of genistein on mouse embryo development in vivo Female mice were fed a standard diet supplemented with or without genistein (10 µmol/L) in the drinking water for a total of 5 d. Twenty-four hours after the initiation of genistein exposure, the mice were mated overnight with a single fertile male of the same strain. The blastocysts were obtained by flushing the uterine horn on d 4 after mating, and cell apopto-sis and proliferation were analyzed as described above.
Statistics The data were analyzed using one-way ANOVA and t-tests, and are presented as the mean±SD. P<0.05 was considered significant.
Results
Effects of genistein on cell apoptosis in mouse blastocysts To study the apoptotic effects of genistein on embryos, we treated mouse blastocysts (150 blastocysts in each group) with 0–50 µmol/L genistein at 37 oC for 24 h, and then measured cell apoptosis. TUNEL staining revealed that treatment with 25 and 50 µmol/L genistein dose-dependently increased apoptosis in the mouse blastocysts (Figure 1A), and quantitative analyses revealed 4 to 6.7 fold more apo-ptotic cells in the genistein-treated blastocysts versus the untreated controls (Figure 1B). For the comparison, we used similar methods to analyze the cytotoxic effects of daidzein, another type of soybean isoflavone, on mouse blastocysts. Interestingly, TUNEL staining revealed that daidzein did not induce cell apoptosis in mouse blastocysts under our experimental conditions (Figure 1A, 1B). Additional TUNEL staining experiments revealed that the effect of genistein on cell apoptosis was more pronounced in the ICM than in the TE (Figure 1C), and Annexin-V staining revealed a higher rate of Annexin-V-positive cells in the ICM of treated blastocysts versus the controls, but similar rates of positivity in the TE (Figure 1D). These results indicate that genistein is a potent inducer of apoptosis in mouse blastocysts, primarily in the ICM, whereas daidzein does not appear to induce apoptosis in mouse blastocysts.
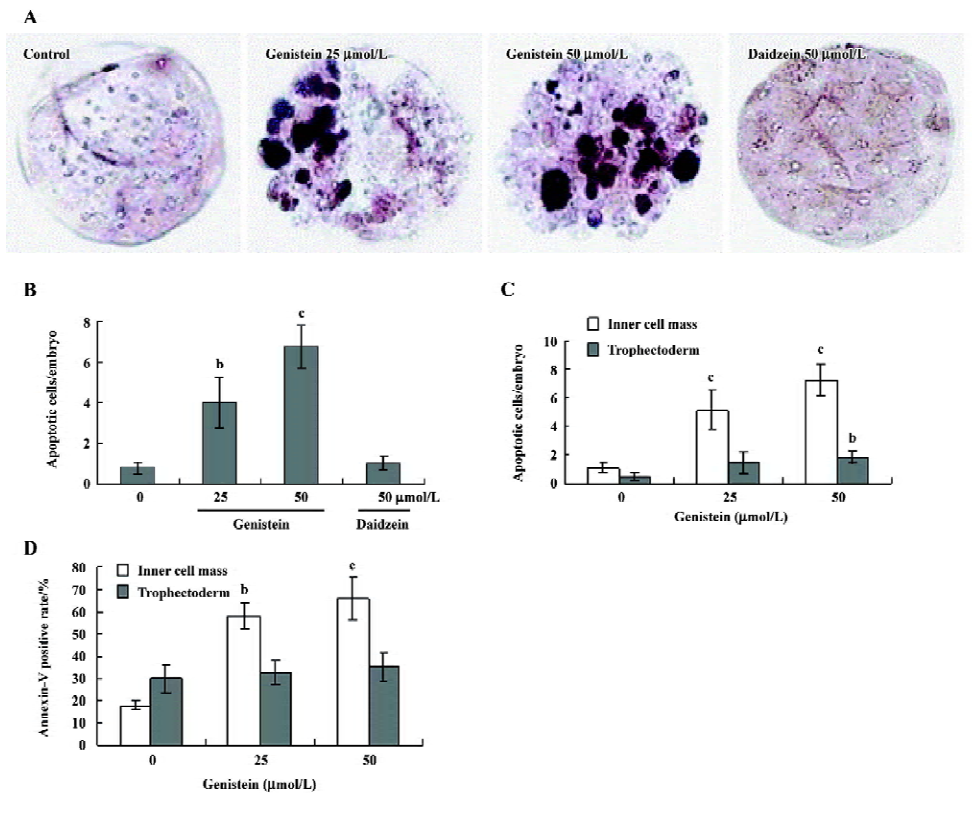
Effects of genistein on the blastocyst cell number To investigate the effect of genistein on cell proliferation in embryos, we treated mouse blastocysts (120 blastocysts in each group) with or without genistein (25–50 µmol/L) for 24 h, and then analyzed proliferation using differential staining followed by cell counting. Our results revealed that the blastocysts treated with 25 or 50 µmol/L of genistein contained fewer cells than the control blastocysts; this effect was more pronounced in the ICM than in the TE (Figure 2).
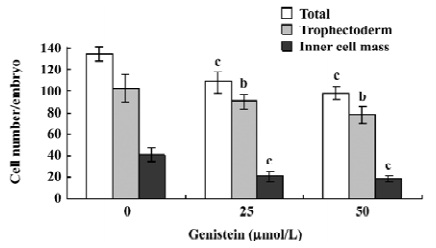
Effects of genistein on implantation and postimplantation development The blastocysts treated with 25 or 50 µmol/L of genistein (148–173 blastocysts, as indicated) and 148 control blastocysts were assessed for in vitro implantation and development. Implantation was similar in both the treatment and control groups (Figure 3A). However, the genistein-treated blastocysts showed reduced formation of the 2 layer ICM and ectoplacental cones, and fewer instances of embryos developing to the advanced egg cylinder stages (LEC and ES stages) when compared with the control group (Figure 3A). Morulas exposed to 25 or 50 µmol/L genistein showed 55%–65% development into blastocysts, whereas 86% of control morulas developed into blastocysts under our experimental conditions (Figure 3B).
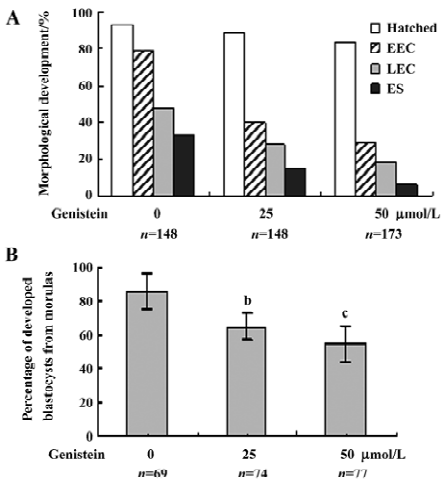
Effect of dietary genistein on mouse blastocyst development in vivo The ability of genistein to trigger apoptosis and developmental injury in vitro led us to suspect that it might have the same activity in vivo. To evaluate this possibility in an animal model, we fed female mice a standard diet supplemented with or without genistein (10 µmol/L) in the drinking water, 24 h prior to mating and during the first 4 d of embryonic development. An analysis of flushed blastocysts (280 per group) revealed that dietary genistein significantly induced apoptosis (Figure 4A) and decreased cell proliferation (Figure 4B) in mouse blastocysts. Collectively, these studies suggest that genistein can induce apoptosis and inhibit the proliferation of embryonic cells, and appears to cause embryonic developmental injury in vitro and in vivo, indicating that genistein may have a hazardous effect on embryonic development.
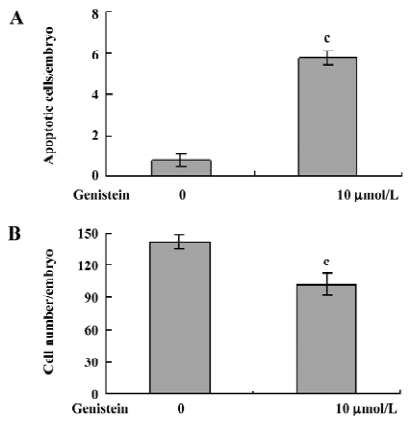
Discussion
Embryonic development is a complex process during which chemical injury can lead to abortion or embryonic malformation. Thus, it is important to examine the possible teratogenic effects of commonly used plant extracts. We herein show that genistein can decrease the viability of mammalian blastocysts via the increased induction of apoptosis (Figure 1). Our results revealed that treatment of mouse blastocysts with 25 and 50 µmol/L genistein dose-depen-dently induced 4 to 6.7 fold increases in apoptosis, as assessed by TUNEL staining (Figure 1A, 1B). In contrast, another soy isoflavone, daidzein, had no such effect (Figure 1A, 1B). Annexin-V staining revealed that genistein-induced cell apoptosis occurred more commonly in the ICM than in the TE (Figure 1C, 1D). Although the treated blastocysts could implant on culture dishes in vitro, postimplantation development was retarded, leading to embryonic death.
Previous reports have shown that genistein can either induce or prevent apoptosis, depending on the cell type or experimental conditions[33,34]. For example, genistein induced apoptosis in immature human thymocytes[19], leukemia cell lines[18], human stomach cancer cells[14], and bladder carcinoma cell lines[35], but inhibited ionization radiation-induced apoptosis of human B lymphocyte precursors[36], taxol-induced apoptosis of a human ovarian tumor cell line[37], anti-CD3-induced apoptosis of mouse thymocytes[20], didemnin B-induced apoptosis of human HL-60 cells[22], and UV irradiation-induced apoptosis of a human epidermal carcinoma cell line[38]. In addition, other studies have demonstrated that genistein can inhibit proliferation and induce differentiation in tumor cells[39–41]. In the present work, we used TUNEL and Annexin-V staining to show that genistein promotes apoptosis in mouse blastocysts (Figure 1).
During embryonic development, cells are often poised between proliferation and apoptosis. Studies by several other groups and our laboratory have demonstrated that genistein can modulate cell apoptosis, but no previous work has examined the apoptotic effects of genistein on embryonic cell death. We herein report that genistein treatment of mouse blastocysts decreased cell numbers, increased apoptosis, and triggered developmental delays in postimplantation mouse embryos in vitro, and further report that dietary genistein appeared to have a teratogenic effect in vivo. Taken together, our novel observations in conjunction with the previous reports suggest that the actions of genistein depend on the cell type and treatment duration/dosage. Clearly, additional studies will be required to assess the molecular mechanism of action by which genistein affects cell apoptosis.
Although the effect was more pronounced in the ICM, some genistein-induced cell loss and apoptosis was observed in the TE as well (Figures 1C, 2). The TE arises from the trophoblast at the blastocyst stage, and subsequently develops into a sphere of epithelial cells surrounding the ICM and the blastocoel; these cells contribute to the placenta and are required for the development of the mammalian conceptus[42]. Although we observed that genistein treatment slightly reduced the cell number and increased apoptosis in the TE of mouse blastocysts in this study (Figures 1C, 1D, 2), embryonic implantation did not appear to be affected in vitro (Figure 3A). Future work will be required to assess the effects of genistein on differentiation and giant-cell formation in vitro and in vivo.
Genistein has been shown to act as an agonist for estrogen receptor (ER)α and ERβ[43]. The ability of genistein to protect against mammary carcinomas has been attributed to its anti-estrogenic effects[44], while other studies demonstrated that the estrogenic and/or anti-estrogenic activities of genistein may reduce or enhance estrogen-dependent tumor growth, depending on the dose and timing of exposure[45,46]. To test whether genistein treatment could affect ER expression, we used real time PCR to investigate the effect of genistein treatment on the expression levels of ERα and ERβ in mouse blastocysts. Our results revealed no significant differences in ERα and ERβ expression levels between the genistein-treated and the control blastocysts (data not shown), suggesting that genistein-induced cell apoptosis does not act via the alteration of ERα and ERβ expression levels in the studied mouse blastocysts.
Researchers recently investigated the effects of phyto-estrogens such as genistein on the production of the protein hormone hCG in trophoblasts. Their results revealed that hCG production was decreased by genistein treatment in the trophoblasts of term placentas[47]. Another study showed that genistein affected the production of hCG and the steroid hormone progesterone in the BeWo and Jeg3 trophoblast cell lines[48]. Importantly, the report noted that different treatment dosages of genistein could cause different effects on the production of progestrogen and hCG. The result showed that a correlation between the effects on the proliferation and the production of progesterone and hCG at high concentrations of genistein (>1 mmol/mL). With low concentrations of genistein treatment, the study found that a stimulation of the production of hCG and a weak inhibition of proliferation in both cell lines BeWo and Jeg3[48]. These results seem to imply that exposure to genistein during sensitive periods of development may alter reproductive functions and influence fertility. These reports, in conjunction with the present findings, suggest that genistein acts as a teratogen, negatively impacting embryonic development via the inhibition of trophoblast cell proliferation and hCG production, the inactivation of tyrosine kinases, and the induction of cell apoptosis.
In summary, we show for the first time that genistein can decrease the viability of mammalian blastocysts by inducing apoptosis in the ICM. Although additional work will be required to fully elucidate the action mechanisms of genistein, especially in relation to the dietary use of isoflavones (such as genistein) in pregnant women, this study provides the first evidence that genistein could have teratogenic effects through the induction of apoptosis.
References
- Ellis RE, Yuan JY, Horvitz HR. Mechanisms and functions of cell death. Annu Rev Cell Biol 1991;7:663-98.
- Thompson CB. Apoptosis in the pathogenesis and treatment of disease. Science 1995;267:1456-62.
- Hardy K. Cell death in the mammalian blastocyst. Mol Hum Reprod 1997;3:919-25.
- Hardy K, Stark J, Winston RM. Maintenance of the inner cell mass in human blastocysts from fragmented embryos. Biol Reprod 2003;68:1165-9.
- Byrne AT, Southgate J, Brison DR, Leese HJ. Analysis of apoptosis in the preimplantation bovine embryo using TUNEL. J Reprod Fertil 1999;117:97-105.
- Dong W, Teraoka H, Yamazaki K, Tsukiyama S, Imani S, Imagawa T, et al. 2,3,7,8-tetrachlorodibenzo-p-dioxin toxicity in the zebrafish embryo: local circulation failure in the dorsal midbrain is associated with increased apoptosis. Toxicol Sci 2002;69:191-201.
- Little SA, Kim WK, Mirkes PE. Teratogen-induced activation of caspase-6 and caspase-7 in early postimplantation mouse embryos. Cell Biol Toxicol 2003;19:215-26.
- Hsuuw YD, Chang CK, Chan WH, Yu JS. Curcumin prevents methylglyoxal-induced oxidative stress and apoptosis in mouse embryonic stem cells and blastocysts. J Cell Physiol 2005;205:379-86.
- Chan WH. Ginkgolides induce apoptosis and decrease cell numbers in mouse blastocysts. Biochem Biophys Res Commun 2005;338:1263-7.
- Chan WH. Ginkgolide B induces apoptosis and developmental injury in mouse embryonic stem cells and blastocysts. Hum Reprod 2006;21:2985-95.
- Akiyama T, Ishida J, Nakagawa S, Ogawara H, Watanabe S, Itoh N, et al. Genistein, a specific inhibitor of tyrosine-specific protein kinases. J Biol Chem 1987;262:5592-5.
- Markovits J, Linassier C, Fosse P, Couprie J, Pierre J, Jacquemin-Sablon A, et al. Inhibitory effects of the tyrosine kinase inhibitor genistein on mammalian DNA topoisomerase II. Cancer Res 1989;49:5111-7.
- Constantinou A, Kiguchi K, Huberman E. Induction of differentiation and DNA strand breakage in human HL-60 and K-562 leukemia cells by genistein. Cancer Res 1990;50:2618-24.
- Yanagihara K, Ito A, Toge T, Numoto M. Antiproliferative effects of isoflavones on human cancer cell lines established from the gastrointestinal tract. Cancer Res 1993;53:5815-21.
- Rauth S, Kichina J, Green A. Inhibition of growth and induction of differentiation of metastatic melanoma cells in vitro by genistein: chemosensitivity is regulated by cellular p53. Br J Cancer 1997;75:1559-66.
- Brown A, Jolly P, Wei H. Genistein modulates neuroblastoma cell proliferation and differentiation through induction of apoptosis and regulation of tyrosine kinase activity and N-myc expression. Carcinogenesis 1998;19:991-7.
- Azuma Y, Onishi Y, Sato Y, Kizaki H. Induction of mouse thymocyte apoptosis by inhibitors of tyrosine kinases is associated with dephosphorylation of nuclear proteins. Cell Immunol 1993;152:271-8.
- Bergamaschi G, Rosti V, Danova M, Ponchio L, Lucotti C, Cazzola M. Inhibitors of tyrosine phosphorylation induce apoptosis in human leukemic cell lines. Leukemia 1993;7:2012-8.
- McCabe MJ Jr, Orrenius S. Genistein induces apoptosis in immature human thymocytes by inhibiting topoisomerase-II. Biochem Biophys Res Commun 1993;194:944-50.
- Migita K, Eguchi K, Kawabe Y, Mizokami A, Tsukada T, Nagataki S. Prevention of anti-CD3 monoclonal antibody-induced thymic apoptosis by protein tyrosine kinase inhibitors. J Immunol 1994;153:3457-65.
- Bronte V, Macino B, Zambon A, Rosato A, Mandruzzato S, Zanovello P, et al. Protein tyrosine kinases and phosphatases control apoptosis induced by extracellular adenosine 5'-triphos-phate. Biochem Biophys Res Commun 1996;218:344-51.
- Johnson KL, Vaillant F, Lawen A. Protein tyrosine kinase inhibitors prevent didemnin B-induced apoptosis in HL-60 cells. FEBS Lett 1996;383:1-5.
- Heo JS, Han HJ. PKC and MAPKs pathways mediate EGF-induced stimulation of 2-deoxyglucose uptake in mouse embryonic stem cells. Cell Physiol Biochem 2006;17:145-58.
- Bogoliubova NA, Bogoliubova IO, Parfenov VN, Sekirina GG. Characteristic of structural and functional organization of two-cell mouse embryos exposed to inhibitors of cell proliferation. Tsitologiia 1999;41:698-706.
- Jacquet P, de Saint-Georges L, Barrio S, Baugnet-Mahieu L. Mor-phological effects of caffeine, okadaic acid and genistein in one-cell mouse embryos blocked in G2 by X-irradiation. Int J Radiat Biol 1995;67:347-58.
- Goval JJ, Alexandre H. Effect of genistein on the temporal coordination of cleavage and compaction in mouse preimplantation embryos. Eur J Morphol 2000;38:88-96.
- Pampfer S, de Hertogh R, Vanderheyden I, Michiels B, Vercheval M. Decreased inner cell mass proportion in blastocysts from diabetic rats. Diabetes 1990;39:471-6.
- Hardy K, Handyside AH, Winston RM. The human blastocyst: cell number, death and allocation during late preimplantation development in vitro. Development 1989;107:597-604.
- Gardner RL, Davies TJ. Lack of coupling between onset of giant transformation and genome endoreduplication in the mural trophectoderm of the mouse blastocyst. J Exp Zool 1993;265:54-60.
- Huang FJ, Wu TC, Tsai MY. Effect of retinoic acid on implantation and post-implantation development of mouse embryos in vitro. Hum Reprod 2001;16:2171-6.
- Witschi E. Characterization of developmental stages. Part II. Rat. In: Biology Data Book, 2nd ed. Washington, DC: Federation of American Societies of Experimental Biologies; 1972. p 178–80.
- Huang FJ, Shen CC, Chang SY, Wu TC, Hsuuw YD. Retinoic acid decreases the viability of mouse blastocysts in vitro. Hum Reprod 2003;18:130-6.
- Gu Y, Zhu CF, Iwamoto H, Chen JS. Genistein inhibits invasive potential of human hepatocellular carcinoma by altering cell cycle, apoptosis, and angiogenesis. World J Gastroenterol 2005;11:6512-7.
- Raffoul JJ, Wang Y, Kucuk O, Forman JD, Sarkar FH, Hillman GG. Genistein inhibits radiation-induced activation of NF-kappaB in prostate cancer cells promoting apoptosis and G2/M cell cycle arrest. BMC Cancer 2006;6:107.
- Zhou JR, Mukherjee P, Gugger ET, Tanaka T, Blackburn GL, Clinton SK. Inhibition of murine bladder tumorigenesis by soy isoflavones via alterations in the cell cycle, apoptosis, and angiogenesis. Cancer Res 1998;58:5231-8.
- Uckun FM, Tuel-Ahlgren L, Song CW, Waddick K, Myers DE, Kirihara J, et al. Ionizing radiation stimulates unidentified tyrosine-specific protein kinases in human B-lymphocyte pre-cursors, triggering apoptosis and clonogenic cell death. Proc Natl Acad Sci USA 1992;89:9005-9.
- Liu Y, Bhalla K, Hill C, Priest DG. Evidence for involvement of tyrosine phosphorylation in taxol-induced apoptosis in a human ovarian tumor cell line. Biochem Pharmacol 1994;48:1265-72.
- Chan WH, Yu JS. Inhibition of UV irradiation-induced oxidative stress and apoptotic biochemical changes in human epidermal carcinoma A431 cells by genistein. J Cell Biochem 2000;78:73-84.
- Cao F, Jin TY, Zhou YF. Inhibitory effect of isoflavones on prostate cancer cells and PTEN gene. Biomed Environ Sci 2006;19:35-41.
- Pinski J, Wang Q, Quek ML, Cole A, Cooc J, Danenberg K, et al. Genistein-induced neuroendocrine differentiation of prostate cancer cells. Prostate 2006;66:1136-43.
- Morris C, Thorpe J, Ambrosio L, Santin M. The soybean isoflavone genistein induces differentiation of MG63 human osteosarcoma osteoblasts. J Nutr 2006;136:1166-70.
- Cross JC, Werb Z, Fisher SJ. Implantation and the placenta: key pieces of the development puzzle. Science 1994;266:1508-18.
- Mueller SO, Simon S, Chae K, Metzler M, Korach KS. Phyto-estrogens and their human metabolites show distinct agonistic and antagonistic properties on estrogen receptor alpha (ERalpha) and ERbeta in human cells. Toxicol Sci 2004;80:14-25.
- Murrill WB, Brown NM, Zhang JX, Manzolillo PA, Barnes S, Lamartiniere CA. Prepubertal genistein exposure suppresses mammary cancer and enhances gland differentiation in rats. Carcinogenesis 1996;17:1451-7.
- Allred CD, Ju YH, Allred KF, Chang J, Helferich WG. Dietary genistein stimulates growth of estrogen-dependent breast cancer tumors similar to that observed with genistein. Carcinogenesis 2001;22:1667-73.
- Cotroneo MS, Wang J, Fritz WA, Eltoum IE, Lamartiniere CA. Genistein action in the prepubertal mammary gland in a chemo-prevention model. Carcinogenesis 2002;23:1467-74.
- Jeschke U, Briese V, Richter DU, Bruer G, Plessow D, Waldschlager J, et al. Effects of phytoestrogens genistein and daidzein on production of human chorionic gonadotropin in term trophoblast cells in vitro. Gynecol Endocrinol 2005;21:180-4.
- Plessow D, Waldschlager J, Richter DU, Jeschke U, Bruer G, Briese V, et al. Effects of phytoestrogens on the trophoblast tumour cell lines BeWo and Jeg3. Anticancer Res 2003;23:1081-6.