G protein-coupled inwardly rectifying potassium channels in dorsal root ganglion neurons1
Introduction
The G protein-coupled inwardly rectifying potassium channel (GIRK) is a member of the inwardly rectifying potassium (K+) channel family. Five GIRK subunits have been identified in mammals and Xenopus oocytes, named GIRK1–5 (also designated Kir3.1–5)[1]. GIRK channels are homotetramers or heterotetramers. In vivo, GIRK1 has to assemble with the other GIRK family members to form functional channels[1]. GIRK channels are downstream effectors of G protein-coupled receptors, and they can be activated by direct binding of the βγ subunits of G protein to the channels[2–4]. A large number of neuromodulators are capable of opening GIRK channels in a G protein-dependent, membrane-delimited fashion, including adenosine, serotonin, dopamine, adrenalin, acetylcholine, opioids, and somatostatin[5–7]. GIRK channels are distributed in the pancreas, heart and brain, and play pivotal roles in controlling insulin release, vascular tone, heart rate, neuronal signaling, and membrane excitability[8]. For example, the activation of the heart muscarinic m2 receptor slows the heart rate by activating GIRK1/GIRK4 heterotetrameric channels[9,10]. In the hippocampus, baclofen can activate GIRK channels, which produce a reversible reduction of EPSP[11].
Dorsal root ganglion (DRG) neurons are the primary neurons for somatic and visceral afferentation. Their bodies reside in the DRG, and extend axons that innervate both peripheral and central targets. A diverse group of ligand- and voltage-gated ion channels transduce innocuous and noxious (nociceptive) stimuli into depolarizations that are conducted along axons and finally converted into neurotransmitter release[12]. There are many G protein-coupled receptors on DRG neurons, such as the μ opioid receptor[13], the GABAB receptor[14], the oxytocin receptor[15], SNSR4/mrgX1[16], etc. The activation of these receptors can affect the conductance of DRG neurons. For example, the activation of human sensory neuron-specific G protein-coupled receptors (SNSR), expressed solely in small diameter primary sensory neurons, modulates neuronal K+ channels and synaptic transmission[16]; clonidine, an α2-adrenoceptor agonist, inhibits hyperpolarization-activated currents in rat DRG neurons[17].
Although GIRK channels are very important to mediate signaling through G protein-coupled receptors, no data concerning the expression of functional GIRK channels in adult DRG neurons has been reported. In the present report, we show that 4 subtypes of GIRK channel subunits are expressed on DRG neurons, and the GIRK channels are functionally coupled to the GABAB receptor.
Materials and methods
RT-PCR analysis The total RNA from these DRG was extracted using the RNA-Clean kit (TaKaRa, Dalian, China) and treated with DNase I (RNase-free from TaKaRa, Dalian, China). cDNA were synthesized using the M-MLV RTase cDNA Synthesis Kit (TaKaRa, China). PCR was performed using the following primer pairs: GIRK1, forward 5'-CGGCA-GCGGTTCGTGGACAAG-3' and reverse 5'-TGGCTGGTGC-TATTAAAGGGGAAGACAT-3'; GIRK2, forward 5'-AGCC-GAGACAGGACCAAAAGGAAAATC-3' and reverse 5'-ACGGGGTGCTGGTCTCATAGGTCTC-3'; GIRK3, forward 5'-CTACCGCTACCTGACCGACCTGTT-3' and reverse 5'-AGCCCCTTCTTCCTCCACCTTCT-3'; GIRK4, forward 5'-GGAGAAGACCGGCAAGTGTAACG-3' and reverse 5'-GCCCCCAAGCAAAGGAGGAC-3'. For GIRK1, PCR amplification was performed for 35 cycles using Taq polymerase (TaKaRa, China), each cycle consisted of 30 s at 94 °C, 30 s at 60 °C, and 1 min at 72 °C. For GIRK2, PCR amplification was performed for 35 cycles using LA Taq polymerase and buffer I (TaKaRa, China), each cycle consisted of 40 s at 94 °C, 40s at 60 °C, and 1 min at 72 °C. For GIRK3, PCR amplification was performed for 35 cycles using LA Taq polymerase and buffer II (TaKaRa, China), each cycle consisted of 40 s at 94 °C, 40 s at 60 °C, and 1 min at 72 °C. For GIRK4, PCR amplification was performed for 35 cycles using Taq polymerase (TaKaRa, China), each cycle consisted of 40 s at 94 °C, 40 s at 58 °C, and 1 min at 72 °C. The amplified samples were analyzed by standard agarose gel electrophoresis. DL-2000 DNA marker (TaKaRa, China) or BenchTop 1 kb DNA ladder (Promega, Madison, Wisconsin, USA) was used. All the products were further confirmed by DNA sequencing.
Preparation of isolated DRG The animal experiments were carried out in adherence with the National Institutes of Health Guidelines on the Use of Laboratory Animals and were approved by Second Military Medical University Committee on Animal Care. Adult male Sprague-Dawley rats (150–200 g), from the Experimental Animal Center in Second Military Medical University, were housed with access to food and water ad libitum under standard conditions in a 12/12 light dark cycle. The adult rats were deeply anaesthetized with 40 mg/mL sodium pentobarbital and killed by decapitation. The DRG were removed and digested in solution containing 1 mg/mL collagenase type 1A (Sigma, St Louis, MO, USA), 0.4 mg/mL trypsin type I (Sigma, USA), and 0.1 mg/mL Dnase I (Sigma, USA) in DMEM at 37 °C for 40 min, triturated, and plated on coverslips. The cells were observed with an Olympus IX71 inverted microscope (Olympus Optical, Tokyo, Japan).
Whole cell patch-clamp recording The cells were bathed in normal Ringer’s solution containing (in mmol/L): NaCl, 140; KCl, 5; CaCl2, 2; MgCl2, 1; glucose, 10 and HEPES, 10; pH 7.4 adjusted with NaOH. When an increase in [K+]o was needed, NaCl was reduced by equal amounts to keep the osmolarity unchanged. Patch pipettes had a resistance of 6–8 MΩ. The pipette solution consisted of (in mmol/L): KCl, 120; NaCl, 20; HEPES, 10; glucose·H2O, 10; EGTA, 1; Na2ATP·3H2O, 3; MgCl2·6H2O, 3; NaGTP, 0.3; pH 7.2 adjusted with NaOH. Recordings were made with an Axon-patch 200B amplifier and pClampex 9.0 software (Axon Instruments, Sunnyvale, CA, USA). Analogue signals were filtered at 2 kHz, sampled at 10 kHz and stored on a PC hard disk for further analysis. Baclofen and GTPγS used in the experiments were from Sigma. Data analysis was performed using Clampfit 9.0 (Axon Instruments, USA) and Origin 7.0 (OriginLab Corporation, Northampton, MA, USA). Data were all presented as mean±SD.
Results
GIRK channel subunits in rat DRG In the RT-PCR analysis of total RNA extracted from DRG, the oligonucleotide primers for GIRK1−4 showed amplification of products of expected sizes (Figure 1). These results demonstrated that the mRNA for the 4 subunits of GIRK were expressed in the adult DRG.
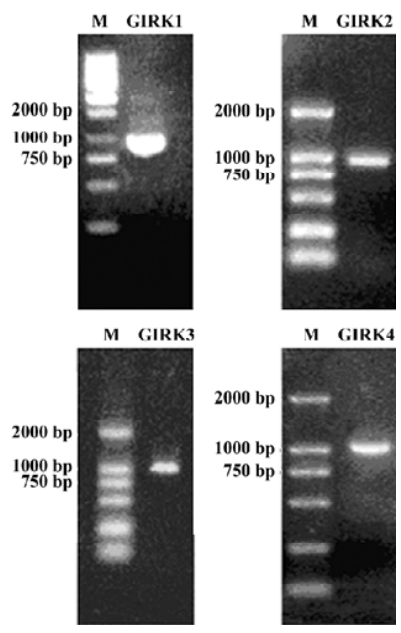
GIRK currents in DRG neurons To determine whether the GIRK subunits in the DRG neurons contributed to form functional channels, voltage-dependent whole-cell currents from isolated DRG neurons were recorded and characterized. After dissociation procedures, DRG neurons dendrites might have been destroyed, and only neurons with a thin axon were selected to be tested. Neurons retained their active properties after isolation as demonstrated by the voltage-clamp protocol. Successive depolarizing voltage steps evoked typical sequences of fast inward (presumed Na+) currents followed by slow outward (presumed K+) currents (Figure 2).
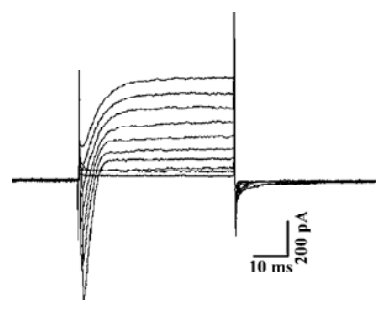
Figure 3A shows the voltage-dependent currents induced from a DRG neuron when the cell membrane potential was changed by a ramp protocol from +90 mV to -190 mV, for 900 ms in Ringer’s solution containing 5, 10, 20, and 45 mmol/L K+. The currents were clearly nonlinear, showing inward rectification. They increased in size in a concentration-dependent manner when [K+]o was raised. Furthermore, the reversal potential was shifted as predicted by the Nernst equation (Figure 3B). These results strongly suggest that K+ was the dominant current carrier.
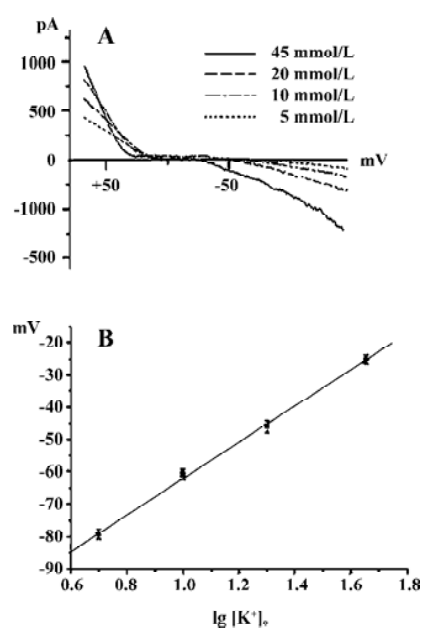
Ba2+ can block Kir channel currents in a dose-dependent manner. Figure 4A shows the effects of 300 µmol/L Ba2+ on the inwardly rectifying currents in a DRG neuron induced by a ramp voltage change from 0 mV to -90 mV in 45 mmol/L K+-containing Ringer’s solution. Ba2+ 300 µmol/L significantly reduced the basal inward currents at 80 mV by 23%±10% (n=6; Figure 4B). This result implies that the inward currents mediated by inwardly rectifying K+ channels existed.
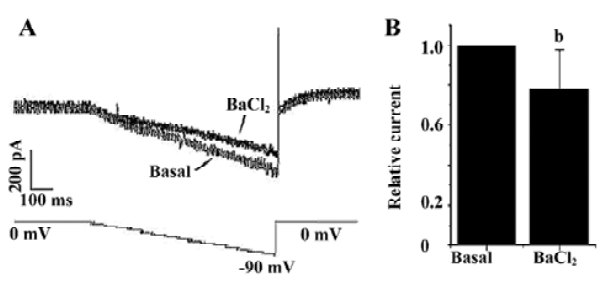
GABAB receptors have been reported to be highly expressed in DRG neurons[14]. To test whether the inwardly rectifying currents in the DRG were regulated by the G protein-coupled receptor, we used the GABAB receptor agonist baclofen to activate the receptor in DRG neurons. Figure 5A, 5B show that the inward currents were markedly increased in magnitude with time following the perfusion of 100 µmol/L baclofen. An increase of 260%±140% (n=6) in the current obtained at -80 mV was found with 100 µmol/L baclofen (Figure 5C). The currents enhanced by baclofen were considerably suppressed by addition of 300 µmol/L Ba2+ (Figure 5A, 5B). The currents obtained in the presence of 300 µmol/L Ba2+ were actually smaller in magnitude than the basal currents (Figure 5A–5C), which may be due to the fact that Ba2+ also suppressed the intrinsic fraction currents mediated by the Kir channels.
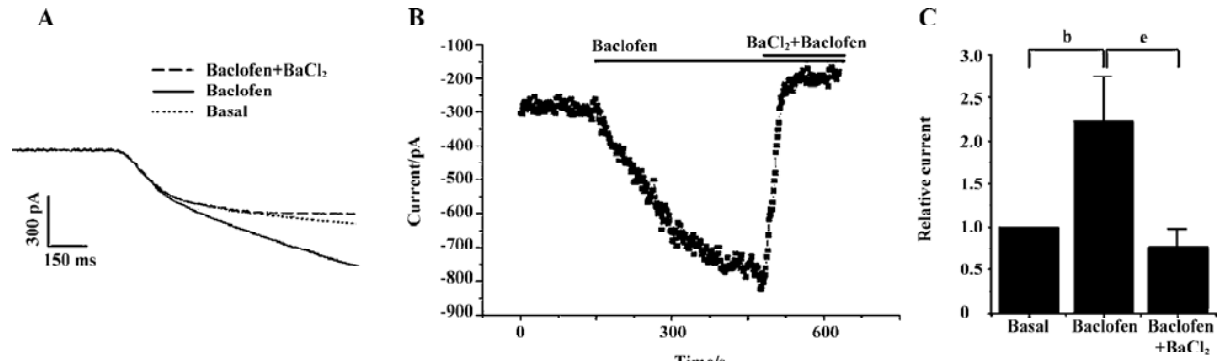
To further confirm that the activation of G protein can activate the inwardly rectifying currents of the DRG neurons, changes in inward currents were studied when guanosine triphosphate (GTP) in the pipette solution was omitted and replaced with 300 µmol/L GTPγS, a nonhydrolysable GTP analogue which can activate the G protein[18]. Figure 6A shows the inward currents markedly increased in magnitude with time following the inclusion of 300 µmol/L GTPγS. An increase of 136%±38% (n=6) in the current obtained at -80 mV was found with 300 µmol/L GTPγS included in the pipette (Figure 6B). The currents enhanced by GTPγS were considerably suppressed by the addition of 300 µmol/L Ba2+ (Figure 6A, 6B). These data demonstrated that the GIRK channels expressed in DRG neurons contributed to the inward currents activated by G proteins.
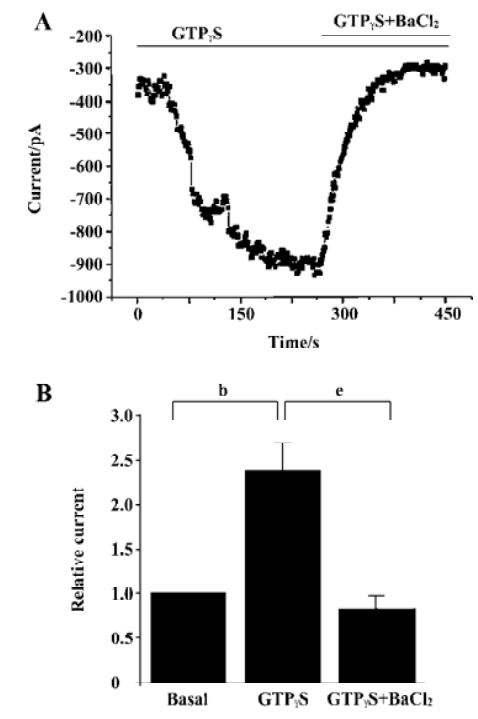
Discussion
Besides the heart and pancreas, GIRK distributes in the nervous system. Earlier studies have demonstrated GIRK1–4 mRNA distribute distinctly in rat brains[19–21]. In adult mice, GIRK2-like immunostaining was detected in lamina II in all levels of the spinal cord, but not in the DRG[22], suggesting that functional GIRK channels of adult mouse DRG neurons were composed of other GIRK subunits except GIRK2. Furthermore, Kanjhan et al reported that no functional GIRK currents were recorded in P0 mouse DRG neurons[23], and they hypothesized that the functional expression of GIRK channels might increase with exposure to sensory of painful stimuli during postnatal development. In rats, it has been shown that GIRK1–3 mRNA are localized in DRG neurons at embryonic d 17[24]. In the present study, we demonstrated the transcription of GIRK1–4 in adult rat DRG neurons. Our results indicate that GIRK1–3 mRNA may continuously transcript from embryonic day to adult time in rat DRG neurons, suggesting that the 4 subunits are all candidates for functional GIRK channels in the DRG neurons of adult rats, and also indicating that the subunit compositions of GIRK channels may be changed with different developmental stages in different species.
GIRK channels are widely regarded as postsynaptic effectors in the CNS, primarily because of the clear dissociation of the postsynaptic and presynaptic inhibitory effects of multiple neurotransmitters in hippocampal pyramidal neurons[25]. GIRK1 and GIRK2 were also found almost exclusively in postsynaptic membranes of putative excitatory synapses in the outer layer of the substantia gelatinosa of the dorsal horn[26]. The activation of postsynaptic GIRK1 and/or GIRK2-containing channels in the spinal cord dorsal horn represents a powerful means by which intrathecal µ- and δ-selective opioid agonists evoke analgesia[26]. In the present study, GIRK channels are found functionally expressed in DRG neurons, producing further molecular bases in the regulation of neuronal excitation which modulates sensory information conveying from the periphery to the spinal cord.
GABA can depolarize A- and C-type cells of DRG and decrease input resistance, mainly due to the activation of GABAA receptors and anionic ionotropic receptors expressed in rat DRG neurons[27]. The GABAB receptor has also been reported to be present in DRG neurons and play important physiological roles[14,28,29], and to modulate N-type calcium channels through G(o) pathways[14,28–30]. GABA inhibits peptide secretion through the activation of GABAB receptors that are functionally coupled to pertussis toxin-sensitive G proteins in peripheral sensory neurons from embryonic chick DRG[31]. In the present study, we found the presence of GABAB receptor-GIRK channel complexes on DRG neurons. These findings provide possible mechanism underlying presynaptic inhibition through the activation of GABAB receptors in peripheral sensory neurons.
Conclusion
The mRNA for GIRK1–4 were detected in the DRG of adult rats. GTPγS enhanced inwardly rectifying K+ currents of the DRG neurons, while Ba2+ inhibited such currents. The GIRK channels were shown to couple to GABAB receptors as baclofen increased the inwardly rectifying K+ currents. These results demonstrate that the expression of GIRK channels and the presence of GABAB receptor-GIRK channel complexes in DRG neurons of adult rats.
Acknowledgements
We thank Dr Xu ZHANG and Dr Lan BAO in the Shanghai Institutes for Biological Science for their helpful suggestions and comments.
References
- Mark MD, Herlitze S. G-protein mediated gating of inward-rectifier K+ channels. Eur J Biochem 2000;19:5830-6.
- Logothetis DE, Kurachi Y, Galper J, Neer EJ, Clapham DE. The beta gamma subunits of GTP-binding proteins activate the muscarinic K+ channel in heart. Nature 1987;6102:321-6.
- He C, Zhang H, Mirshahi T, Logothetis DE. Identification of a potassium channel site that interacts with G protein beta gamma subunits to mediate agonist-induced signaling. J Biol Chem 1999;18:1251-74.
- He C, Yan X, Zhang H, Mirshahi T, Jin T, Huang A, et al. Identification of critical residues controlling G protein-gated inwardly rectifying K+ channel activity through interactions with the beta gamma subunits of G proteins. J Biol Chem 2002;8:6088-96.
- Sodickson DL, Bean BP. Neurotransmitter activation of inwardly rectifying potassium current in dissociated hippocampal CA3 neurons: Interactions among multiple receptors. J Neurosci 1998;20:8153-62.
- Yamada M, Inanobe A, Kurachi Y. G protein regulation of potassium ion channels. Pharmacol Rev 1998;4:723-57.
- Karschin A. G protein regulation of inwardly rectifying K+ channels. News Physiol Sci 1999;14:215-20.
- Hille B. Ionic channels of excitable membranes, 2nd ed. Sunderland, MA: Sinauer Associates; 1992.
- Krapivinsky G, Gordon EA, Wickman K, Velimirovic B, Krapivinsky L, Clapham DE. The G-protein-gated atrial K+ channel IKACh is a heteromultimer of two inwardly rectifying K+ channel proteins. Nature 1995;6518:135-41.
- Wickman K, Nemec J, Gendler SJ, Clapham DE. Abnormal heart rate regulation in GIRK4 knockout mice. Neuron 1998;1:103-14.
- Tomoko T, Christian A. Phasic and tonic attenuation of EPSPs by inward rectifier K+ channels in rat hippocampal pyramidal cells. J Physiol 2002;539:67-75.
- McCleskey EW, Gold MS. Ion channels of nociception. Annu Rev Physiol 1999;61:835-56.
- Shaqura MA, Zollner C, Mousa SA, Stein C, Schafer M. Characterization of µ opioid receptor binding and G protein coupling in rat hypothalamus, spinal cord, and primary afferent neurons during inflammatory pain. J Pharmacol Exp Ther 2004;2:7121-8.
- Towers S, Princivalle A, Billinton A, Edmunds M, Bettler B, Urban L, et al. GABAB receptor protein and mRNA distribution in rat spinal cord and dorsal root ganglia. Eur J Neurosci 2000;9:3201-10.
- Yang Q, Wu ZZ, Li X, Li ZW, Wei JB, Hu QS. Modulation by oxytocin of ATP-activated currents in rat dorsal root ganglion neurons. Neuropharmacology 2002;5:910-6.
- Chen H, Ikeda SR. Modulation of ion channels and synaptic transmission by a human sensory neuron-specific G-protein-coupled receptor, SNSR4/mrgX1, heterologously expressed in cultured rat neurons. J Neurosci 2004;21:5044-53.
- Yagi J, Sumino R. Inhibition of a hyperpolarization-activated current by clonidine in rat dorsal root ganglion neurons. J Neurophysiol 1998;3:1094-104.
- Andrade R, Malenka RC, Nicoll RA. A. G protein couples serotonin and GABAB receptors to the same channels in hippocampus. Science 1986;4781:1261-5.
- Karschin CW, Schreibmayer N, Dascal H, Lester N, Davidson A. Karschin. Distribution and localization of a G protein-coupled inwardly rectifying K+ channel in the rat. FEBS Lett 1994;348:139-44.
- Li JH, You ZD, Song CY, Lu CL, He C. The expression of G-protein-gated inwardly rectifying K+ channels GIRK1 and GIRK2 mRNAs in the supraoptic nucleus of the rat and possible role involved. Neuroreport 2001;5:1007-10.
- Karschin C, Dissmann E, Stuhmer W, Karschin A. IRK(1–3) and GIRK(1–4) inwardly rectifying K+ channel mRNAs are differentially expressed in the adult rat brain. J Neurosci 1996;11:3559-70.
- Mitrovic I, Margeta-Mitrovic M, Bader S, Stoffel M, Jan LY, Basbaum AI. Contribution of GIRK2-mediated postsynaptic signaling to opiate and alpha2-adrenergic analgesia and analgesic sex differences. Proc Natl Acad Sci USA 2003;100:271-6.
- Kanjhan R, Coulson EJ, Adams DJ, Bellingham MC. Tertiapin-Q blocks recombinant and native large conductance K+ channels in a use-dependent manner. J Pharmacol Exp Ther 2005;314:1353-61.
- Karschin C, Karschin A. Ontogeny of gene expression of Kir channel subunits in the rat. Mol Cell Neurosci 1997;10:131-48.
- Luscher C, Jan LY, Stoffel M, Malenka RC, Nicoll RA. G protein coupled inwardly rectifying K+ channels (GIRKs) mediate postsynaptic but not presynaptic transmitter actions in hippocampal neurons. Neuron 1997;19:687-95.
- Marker CL, Lujan R, Loh HH, Wickman K. Spinal G-protein-gated potassium channels contribute in a dose-dependent manner to the analgesic effect of mu- and delta- but not kappa-opioids. J Neurosci 2005;25:3551-9.
- Williams J, Zieglgansberger W. Mature spinal ganglion cells are not sensitive to opiate receptor mediated actions. Neurosci Lett 1981;21:211-6.
- Menon-Johansson AS, Berrow N, Dolphin AC. G. (o) transduces GABAB-receptor modulation of N-type calcium channels in cultured dorsal root ganglion neurons. Pflugers Arch 1993;425:335-43.
- Tatebayashi H, Ogata N. Kinetic analysis of the GABAB-mediated inhibition of the high-threshold Ca2+ current in cultured rat sensory neurons. J Physiol 1992;447:391-407.
- Green KA, Cottrell GA. Actions of baclofen on components of the Ca-current in rat and mouse DRG neurons in culture. Br J Pharmacol 1988;94:235-45.
- Holz GG IV, Kream RM, Spiegel A, Dunlap K. G proteins couple alpha-adrenergic and GABAb receptors to inhibition of peptide secretion from peripheral sensory neurons. J Neurosci 1989;9:657-66.