Pharmacokinetics and biodistribution of polymeric micelles of paclitaxel with Pluronic P1231
Introduction
Paclitaxel (PTX) is a naturally occurring taxane extracted from the needles and bark of the Pacific yew tree, Taxus brevifolia, which has shown significant antitumor activity against various tumors[1]. PTX has been used clinically in the treatment of metastatic breast cancer, ovarian cancer, and several other malignancies[2,3].
Because the water solubility of PTX (approximately 0.3 µg/mL) is very low, the commercial preparation of PTX is formulated in a vehicle composed of a 50:50 (v/v) mixture of Cremophor EL (polyethoxylated castor oil) and dehydrated alcohol, which is diluted with 5–20 fold in normal saline or dextrose solution (5%) before administration. However, serious side effects, such as hypersensitivity reactions, nephrotoxicity and neurotoxicity, attributable to Cremophor EL have been reported[4,5]. Lately, a number of alternative vehicles have been developed for solubilizing PTX, including liposomes, lipid emulsions, mixed micelles, cyclodextrin complexes, and paclitaxel conjugates[6–10], which are safer intravenous formulations devoid of Cremophor EL.
Amphiphilic block copolymer micelles are effective vehicles for the solubilization of hydrophobic drugs[11]. Recently, interests have been raised in the application of polymeric micelles as a novel carrier system because of its high drug-loading capacity of the inner core as well as of the unique disposition characteristics in the body[12].
Pluronics are block copolymers consisting of ethylene oxide (EO) and propylene oxide (PO) blocks with the A-B-A structure. Preliminary studies showed that Pluronic molecules displayed important special biological activities, besides the feature of spontaneously forming micelles in aqueous media. The relatively hydrophobic polymers, such as L61 and P85, are promising agents for use in formulations to treat drug-resistant tumors by energy depletion, cellular membrane fluidization and inhibition of the P-gp ATPase activity[13,14].
Most of previous studies on the application of Pluronic as a carrier focused on hydrophilic block polymers, such as Pluronic F68[15], which do not sensitize multidrug resistant (MDR) cells. Furthermore, most of them have high critical micellar concentration (CMC), and, as a result, do not form stable micelles in the injection solutions. Pluronic P123 is a relatively hydrophobic block copolymer, which has a relatively low CMC (4.4×10-6mol/L)[16]. The hydrophilic-lipophilic balance of P123 (HLB=8) is situated between L61 (HLB=3) and P85 (HLB=16). So it has potential in the sensitization of MDR cells. In this work, we prepared PTX-loaded micelles with Pluronic P123 and evaluated the effect of micellization of PTX on pharmacokinetics and tissue distribution.
Materials and methods
Drugs and reagents Paclitaxel was purchased from Xi’an Sanjiang Bio-Engineering (Xi’an, China). PTX injection (Taxol, Anzatax Injection Concentrate, 30 mg/mL) was produced by FH Faulding trading as David Bull Lab (Australia). Diazepam was obtained from National Institute for the Control of Pharmaceutical and Biological Products (Beijing, China). Pluronic P123 was kindly donated by BASF (Ludwigshafen, Germany). All reagents for high performance liquid chromatography (HPLC) analysis, including acetonitrile and methanol were of HPLC grade. Other reagents were of analytical grade.
Animals Sprague-Dawley rats (220±30 g) and female Kunming strain mice (20±5 g) were supplied by Laboratory Animal Center of Fudan University, Shanghai, China. The animals were used following the guideline of the Ethical Committee for Animal Experiments of Fudan University. The animals were acclimatized at a temperature of 25±2 °C and a relative humidity of 70%±5% under natural light/dark conditions for at least 24 h before dosing.
Preparation of paclitaxel-incorporated micelles The polymeric micelles of PTX with Pluronic P123 were prepared by a solid dispersion method[17,18]. Accurately weighed amounts of PTX and Pluronic P123 were added to acetonitrile. After complete dissolution, the solvents were evaporated under reduced pressures at 37 °C and the residual solvents remaining in the matrix were removed under vacuum at room temperature for 24 h. Dissolution of the solid PTX/copolymer matrix was carried out by preheating the matrix in a warm water bath (60 °C) to obtain a transparent gel-like sample. Adding water at the same temperature to the gel-like matrix and stirring by a glass paddle (600 rpm), the clear PTX-incorporated micellar solution was obtained. The solution was filtered through a 0.22 μm filter membrane and freeze-dried.
Characterization of micelles The mean diameter and particle size distributions of the polymeric micelles were determined by dynamic light scattering measurement using a NICOMP 380 ZLS Zeta Potential/Particle Sizer (PSS Nicomp, Santa Barbara, CA, USA) equipped with a 5 mW helium-neon laser at 632.8 nm. Sample solutions filtered through a 0.22 µm filter membrane were transferred into the light scattering cells. The intensity autocorrelation was measured at a scattering angle of 90° at room temperature.
The morphological examination of micelles was performed using a transmission electron microscope (TEM, Philips CM120, Netherlands). In practice, a drop of micellar solution containing 0.1% (w:v) phosphotungstic acids was placed on a carbon film coated on a copper grid and observed at 80 kV in the electron microscope.
In vitro release of PTX from micelles The in vitro release properties of PTX from polymeric micelles were investigated in an aqueous medium containing 1 mol/L sodium salicylate by a dialysis method[19]. The freeze-dried PTX-incorporated micelles were redissolved in water, and 1 mL micellar solution was introduced into a dialysis bag (MWCO=5 kDa, Green Bird Science & Technology Develop-ment, Shanghai, China). The end-sealed dialysis bag was immersed into 50 mL 1 mol/L sodium salicylate solution at 37 °C. The release medium was stirred at the speed of 75 rpm for 24 h. While the solubility of PTX in the release medium was 23.1 µg/mL, the maximum concentration of PTX in the medium was 2.0 µg/mL in this release experiment. So the sink condition was assured. Samples 0.5 mL were withdrawn at different time intervals (0, 10, 20, 30, 45 min, and 1, 2, 4, 6, 9, 12, 24 h) and replaced with an equal volume of fresh medium. The concentration of PTX in the samples was determined by the HPLC method described below. PTX release from stock solution and Taxol placed in a dialysis bag was conducted under the same conditions as the control.
Pharmacokinetic studies Twelve Sprague-Dawley rats were used to investigate the effect of formulation on the pharmacokinetics of PTX after intravenous administration. Rats were divided into 2 groups at random, and given a single 3 mg/kg dose of Taxol or PTX micelles by tail-vein injection. As the control, Taxol was diluted 12-fold to 0.5 mg/mL with 5% glucose solution shortly before administration. And the concentration of PTX in Pluronic P123 micelle solution for pharmacokinetic study was 0.3 mg/mL.
Blood samples (0.5 mL) were collected into heparinized tubes from the femoral artery at 0 min (predose), 0.083, 0.25, 0.5, 1, 2, 3, 4, 6, and 8 h after intravenous administration. Blood was immediately processed for plasma by centrifugation at 900×g for 10 min. Plasma samples were frozen and maintained at -20 ºC until analysis.
Tissue biodistribution studies Seventy-two female Kunming strain mice were used in the experiment of accessing the effect of formulation on the tissue distribution of PTX after intravenous administration. All mice were divided into 2 groups at random and the administration protocol of tissue distribution study was the same as in the pharmacokinetic study. At 0.083, 0.25, 0.5, 1, 2, 3, 4, 6, and 8 h after drug injection, each animal (n=4 for each time point) was killed and heart, spleen, lung, liver, kidney, uterus/ovaries, brain as well as blood samples were collected. Tissue samples were washed in ice-cold saline, blotted with paper towel to remove excess fluid, weighed and stored at -50 ºC until assessed for drug concentration by HPLC.
HPLC analysis The analyses of PTX levels in vitro and in vivo were carried out using an RP-HPLC method on a system equipped with an LC-10ATVP pump, a SPD-10AVP UV-Vis detector (Shimadzu, Kyoto, Japan) and a HS2000 interface (Hangzhou Empire Science & Tech, Hangzhou, China) operated at 230 nm. A reversed-phase column (Gemini 5 μm C18, 150×4.6 mm, Phenomenex, California, USA) was used at room temperature. The mobile phase consisted of acetonitrile, and ammonium acetate buffer solution (10 mmol/L, pH 5.0) (50:45, v/v) was freshly prepared for each run and degassed before use. Samples 20 µL were injected into the HPLC column for all the analyses. With a flow rate of 1.0 mL/min for the mobile phase, the retention time of PTX was approximately 8.2 min.
Tissue samples were homogenized in the mixed solution of acetonitrile and water (50:50, v/v). Diazepam (1 µg/mL, 50 µL) as internal standard was added into 200 µL of plasma or tissue samples and vortexed for 1 min. The drug and internal standard were then extracted into 3 mL of diethyl ether anhydrous by vortex mixing for 2 min. After centrifugation at 6000×g for10 min, the clear supernatant was removed and evaporated under a gentle stream of nitrogen. The residue was then dissolved by 100 µL acetonitrile and centrifuged at 1400×g for 5 min before HPLC analysis.
Statistical analysis The compartment of model was simulated by 3p87 program (Practical Pharmacokinetic Program, 1987, China) and the parameters of pharmacokinetics were obtained. The calculation of AUC was based on statistical moment theory. The pharmacokinetic parameters were analyzed for statistical significance by unpaired Student’s t-test. For this purpose the level of significance was set at α=0.05. In the tissue distribution studies, the AUC could not be determined in individual mice because of the destructive study design.
Results
Characterization of micelles The mean diameter and particle size distribution of the Pluronic P123 micelle were determined by dynamic light scattering measurement. The mean size of blank micelles, PTX-loaded micelles and freeze-dried PTX-loaded micelles were 20.8±2.9 nm, 25.2±2.9 nm, and 28.5±2.1 nm, respectively. It could be seen that the size distribution was relatively narrow (Figure 1). There was no significant difference in particle size of these three micelles (P>0.05).
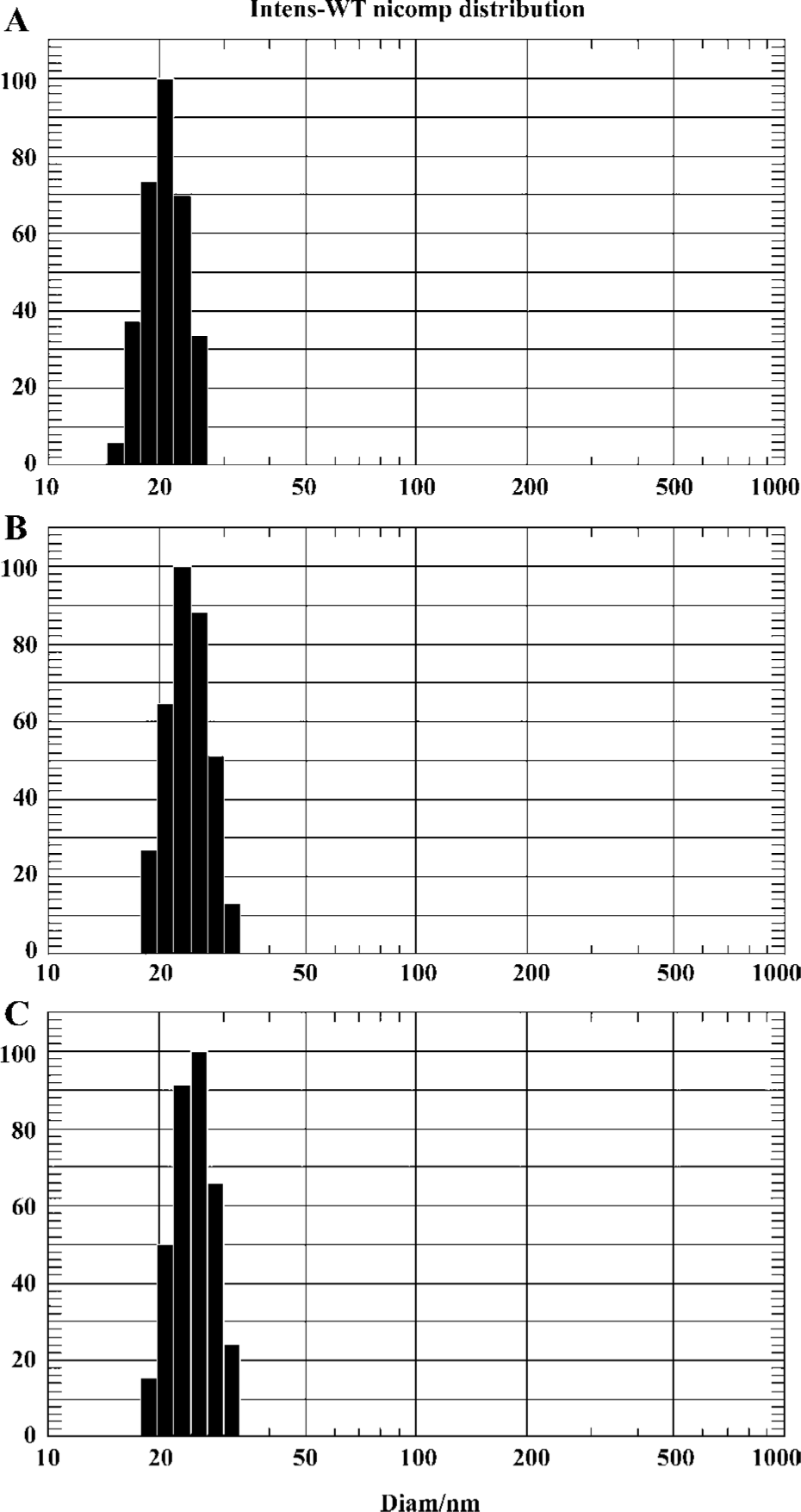
The freeze-dried PTX-incorporated micelles were redissolved in water and the morphology was investigated by TEM. All these micelles had a spherical shape. The particle surface was very smooth and no drug crystal was visible (Figure 2).
In vitro release of PTX from micelles The result of the cumulative PTX release profile from Pluronic P123 micelle is shown in Figure 3. PTX release from stock solution and Taxol were also investigated as control. The maximum concentration of PTX in the medium in this release experiment was 2.0 µg/mL, while the solubility of PTX in the release medium was 23.1 µg/mL. And this system could provide a good sink condition for PTX. PTX was continuously released from the block copolymer micelles in the aqueous medium containing 1 mol/L sodium salicylate for 24 h at 37 ºC. The dialysis bag may become the rate-limiting step in the in vitro release of PTX. In order to examine this possibility, release of PTX from the stock solution across the dialysis membrane was carried out. Only 41.2% PTX was released from the micelles in 4 h, while 95.7% PTX in the stock solution and 90.5% PTX in Taxol were released during the same time period. There was no significant difference in the PTX release from Taxol and stock solution (P>0.05). The Pluronic P123 micelles released approximately 87.8% PTX during 24 h.
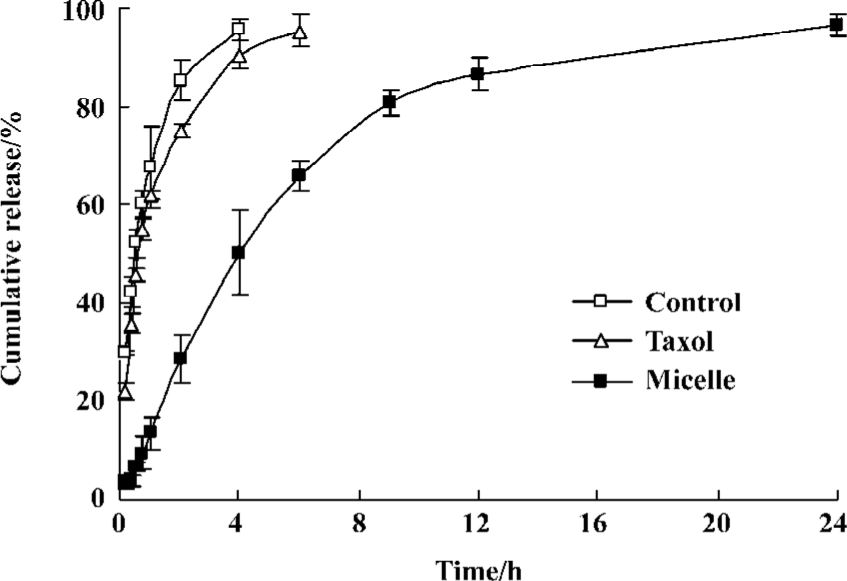
Pharmacokinetics of polymeric micellar paclitaxel The HPLC method for analysis was validated. Linearity in the standard curves was demonstrated over the concentration range studied, and endogenous components had no interference in the chromatograms. The mean plasma concentration-time profiles of PTX after iv administration of PTX micelles and Taxol injection at a single dose of 3 mg/kg are shown in Figure 4. The PTX concentration versus time date was analyzed by two compartmental model analyses with 1/C weighted using computer program 3p87. The pharmacokinetic parameters are shown in Table 1 and analyzed for statistical significance by unpaired Student’s t-test. The concentration showed a rapid decline in distribution phase for the first 1 h after dosing. The t1/2α was less than 0.2 h and there was no significant difference (P>0.05) between the two preparations. The t1/2β of micelles and control was 2.50 h and 5.85 h, respectively. The analysis of variance of the t1/2β, K10, clearance (Cl) showed significant difference among groups (P<0.05). These data indicated that the formulation with micelle increased the systemic circulation time of PTX. And this result was in agreement with the in vitro release of PTX from micelles. Meanwhile, plasma concentrations of PTX in Pluronic P123 micelles were higher than that in Taxol. Pluronic P123 micelles provided significantly higher (2.9-fold) AUC compared to Taxol.
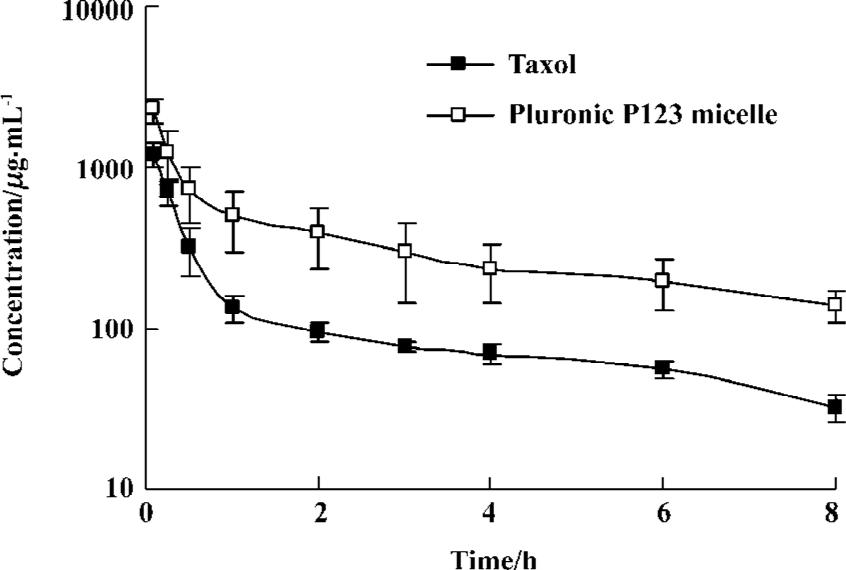
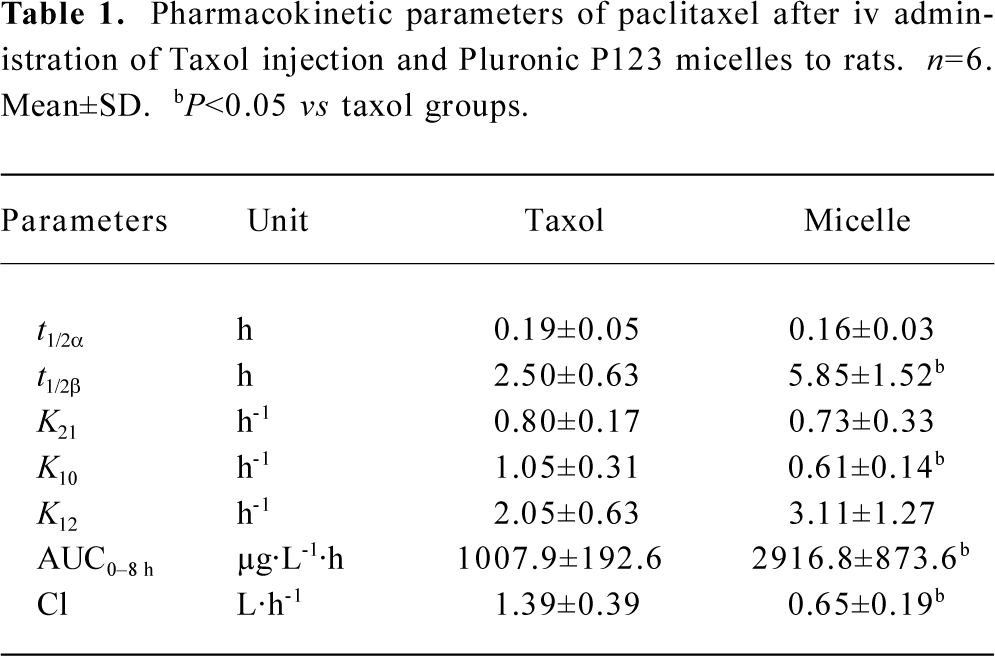
Full table
Tissue distribution of polymeric micellar paclitaxel in healthy animal models In vivo behavior of PTX after intravenous administration of micelles to mice was investigated with Taxol as control (Figure 5). The blood PTX concentration-time profiles observed in mice were similar to the pharmacokinetic study in rats. The time of distribution phase was short and the concentration decreased quickly in this phase. The AUC of two preparations in different tissues, including plasma, heart, spleen, lung, liver, kidney, ovary and uterus, and brain were calculated (Table 2). The PTX AUC of polymeric micelles was lower in liver and higher in plasma, ovary and uterus, lung, and kidney compared to the control. In those tissues, the order in AUC from highest to lowest for Taxol was liver>lung>spleen>kidney>ovary and uterus>heart>plasma>brain. In contrast, the corresponding order for the Pluronic P123 micelles was liver>ovary and uterus>kidney>lung>spleen>heart>plasma>brain.
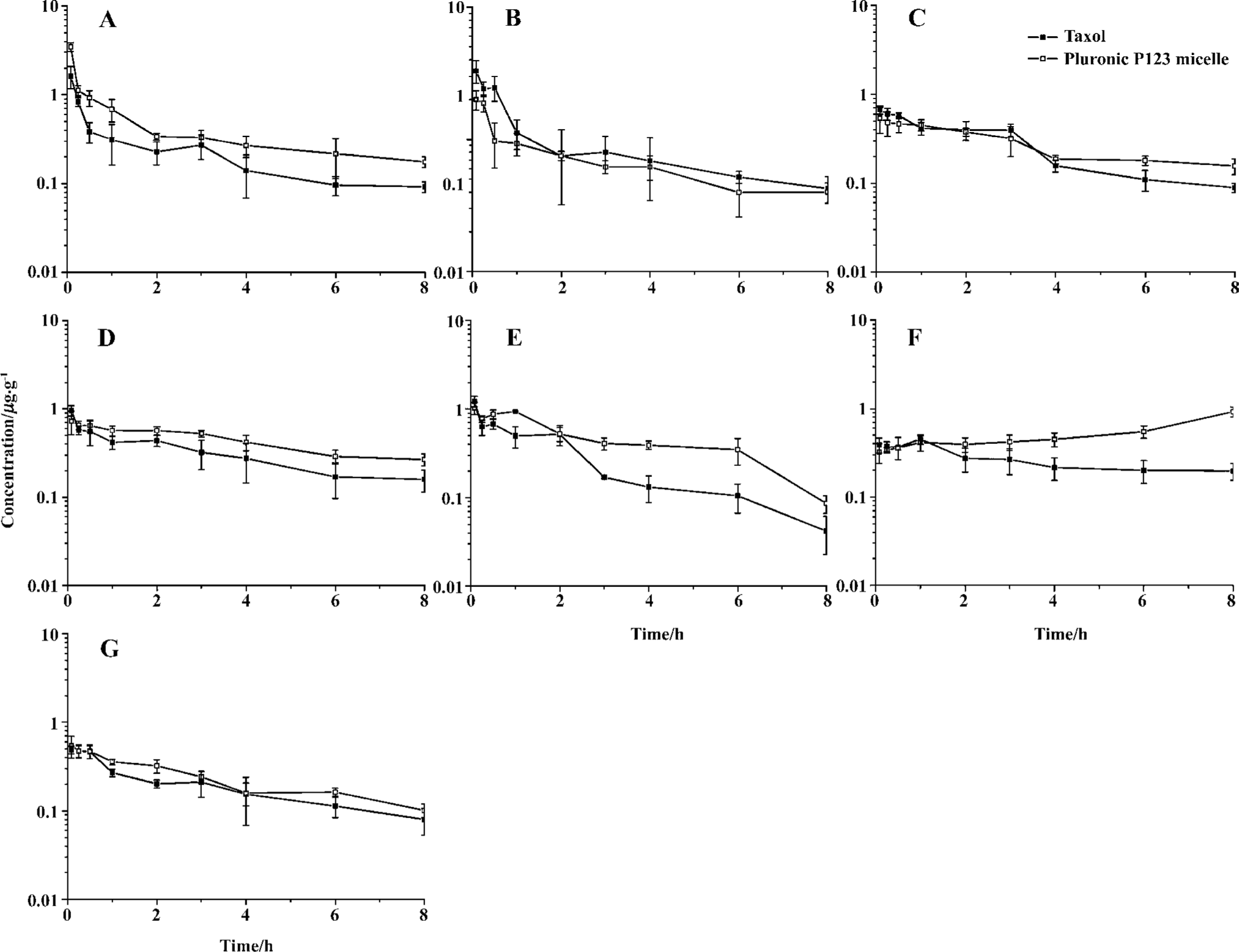
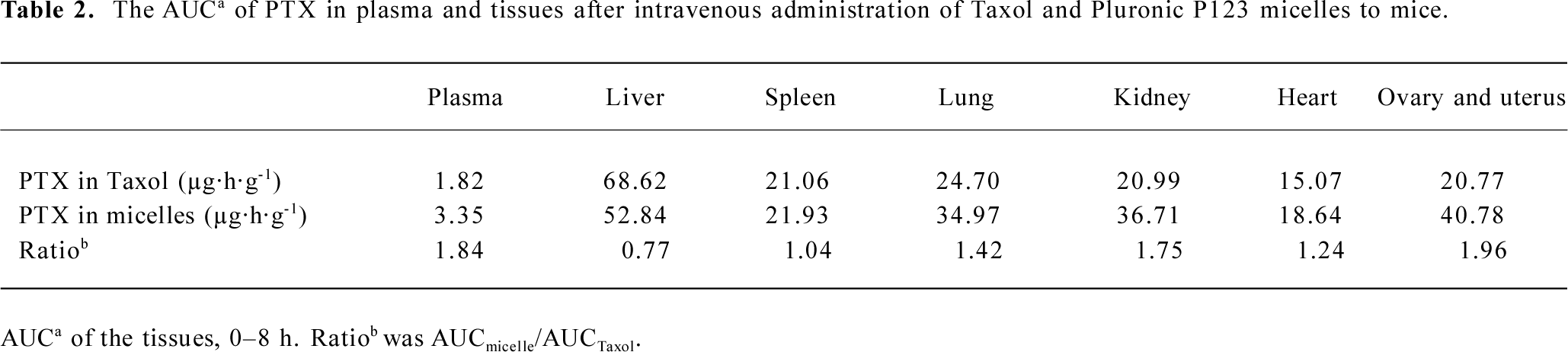
Full table
Discussion
By a solid dispersion method, PTX was effectively loaded in Pluronic P123 micelles. The PTX-loaded micelles had a narrow particle size distribution and a spherical shape.
PTX is a poorly water-soluble drug. Its solubility in water is approximately 0.3 µg/mL. Inclusion of surfactants in release media is the most popular method for in vitro PTX release. But the addition of surfactants in release media might have a significant effect on the micellar structure and distort the release profiles. So maintaining a good sink condition for PTX is one of the challenges in designing in vitro release experiments of polymeric micelles. Hydrotropic agents, sodium salicylate solution, which can solubilize PTX, were used to maintain the sink condition of the aqueous release media by Huh et al[20]. Their work showed that sodium salicylate did not significantly affect the physical stability of micelles up to a concentration of 1.0 mol/L. So in this experiment we used 1 mol/L sodium salicylate solution for drug release. In the in vitro release experiment, almost all PTX in stock solution was rapidly released in 4 h, which indicates that the hindrance effect of the dialysis bag was minimal. The release profile of PTX from Taxol was similar with the stock solution. The cumulative release amount of PTX from Taxol in 4 h was around 90%. Pluronic P123 micelles released only 41.2% PTX in 4 h and 87.8% PTX in 24 h, which was much slower than Taxol. This result showed that the micellar carrier can not only solubilize the water-insoluble drug but also sustain the release of it.
With a EO20-PO70-EO20 structure, Pluronic P123 spontaneously form micelles in aqueous media with the hydrophobic core of poly(propylene oxide) (PPO) and hydrophilic shell of poly(ethylene oxide) (PEO) at concentrations of the block copolymer above the CMC (4.4×10-6 mol/L). The water insoluble drug, PTX, was entrapped in the hydrophobic core. The hydrophilic shell of PEO made the micelles avoid the recognition and uptake by the reticuloendothelial systems and prolong the time of blood circulation of PTX. This effect of Pluronic P123 micelles resulted in an increase in t1/2β, AUC and a reduction of Cl of PTX compared to Taxol. The higher AUC in plasma and lower AUC in liver of PTX-loaded micelles than the control in biodistribution investigation were also a result of this effect.
In general, amphiphilic polymeric micelles can reduce PTX uptake by the kidney and liver. But in our work, the AUC of PTX in kidney of Pluronic P123 micelles was higher than the control. This result may be explained by the biological property of Pluronic P123. It was reported earlier that Pluronics were excreted primarily through the kidneys[21]. The clearance in rats, dogs, and humans was shown to be almost entirely by renal excretion. Studies also showed that the formation of micelles had no effect on the elimination clearance of the block copolymer[22]. The biodistribution study of Pluronic P 105 micelles also showed that the concentration of this material accumulated in kidney cells was higher than the other organs in mice after ip injections[23]. It is acceptable that Pluronic P123 micelles leading to an increase in AUC of PTX in kidney because PTX had no toxic side effect to the kidney in the range of clinical dosage[24].
In tissue distribution studies, the higher concentrations of PTX following iv administration in mice were found in the liver, lung, kidney, and spleen than in plasma. The PTX concentration in the brain was very low; many brain samples couldn’t be accurately measured because the concentration was lower than the limit of quantitation. These results were consistent with the work reported by Rowinsky and Donehower[25].
Both the ovaries and uterus are too small to determine singlely, so in this study, we placed them together. An interesting result in this work was that Pluronic P123 micelles significantly increased the distribution of PTX in the ovaries and uterus, while PTX had been proved effective in the treatment of ovarian cancer.
In summary, these studies have shown that Pluronic P123 micelles may efficiently load, protect and retain PTX in the biological environment. As a result, the pharmacokinetics and tissue distribution parameters of PTX are changed. Incorporating PTX in Pluronic P123 micelles can increase the drug residence time in the blood circulation and the distribution in blood, kidney, ovaries and uterus, and lung. At the same time they can reduce the distribution in the liver. These results suggest that polymeric micellar formulation can provide useful alternative dosage forms for intravenous administration of PTX. A study on the potential of sensitization of MDR cells of Pluronic P123 is in progress in our lab.
References
- Rowinsky EK, Donehower RC. Paclitaxel (Taxol). N Engl J Med 1995;332:1004-14.
- Spencer CM, Faulds D. Paclitaxel. A review of its pharmacodynamic and pharmacokinetic properties and therapeutic potential in the treatment of cancer. Drugs 1994;48:794-847.
- Onetto N, Canett R, Winograd B, Catane R, Dougan M, Grechko J, et al. Overview of taxol safety. J Natl Cancer Inst Monogr 1993;15:131-9.
- Weiss RB, Donehower RC, Wiernik PH, Ohnuma T, Gralla RJ, Trump DL, et al. Hypersensitivity reactions from taxol. J Clin Oncol 1990;8:1263-8.
- Dorr RT. Pharmacology and toxicology of Cremophor EL diluent. Ann Pharmacother 1994;28 Suppl:S11-4.
- Crosasso P, Ceruti M, Brusa P, Arpicco S, Dosio F, Cattel L. Preparation, characterization and properties of sterically stabilized paclitaxel-containing liposomes. J Control Release 2000;63:19-30.
- Lundberg BB, Risovic V, Ramaswamy M, Wasan KM. A lipophilic paclitaxel derivative incorporated in a lipid emulsion for parenteral administration. J Control Release 2003;86:93-100.
- Alkan-Onyuksel H, Ramakrishnan S, Chai HB, Pezzuto JM. A mixed micellar formulation suitable for the parenteral administration of taxol. Pharm Res 1994;11:206-12.
- Liu Y, Chen GS, Chen Y, Cao DX, Ge ZQ, Yuan YJ. Inclusion complexes of paclitaxel and oligo(ethylenediamino) bridged bis(beta-cyclodextrin)s: solubilization and antitumor activity. Bioorg Med Chem 2004;12:5767-75.
- Rodrigues P, Scheuermann K, Stockmar C, Maier G, Fiebig H, Unger C, et al. Synthesis and in vitro efficacy of acid-sensitive poly(ethylene glycol) paclitaxel conjugates. Bioorg Med Chem Lett 2003;13:355-60.
- Kataoka K, Kwon GS, Yokoyama M, Okano T, Sakurai Y. Block copolymer micelles as vehicles for drug delivery. J Control Release 1993;24:119-32.
- Kataoka K, Harada A, Nagasaki Y. Block copolymer micelles for drug delivery: design, characterization and biological significance. Adv Drug Del Rev 2001;47:113-31.
- Kabanov A, Batrakova E, Alakhov V. Pluronic block copolymers for overcoming drug resistance in cancer. Adv Drug Del Rev 2002;54:759-79.
- Kabanov A, Batrakova E, Miller DW. Pluronic block copolymers as modulators of drug efflux transporter activity in the blood-brain barrier. Adv Drug Del Rev 2003;55:151-64.
- Croy SR, Kwon GS. The effects of Pluronic block copolymers on the aggregation state of nystatin. J Control Release 2004;95:161-71.
- Kabanov A, Batrakova E, Alakhov V. Pluronic block copolymers as novel polymer therapeutics for drug and gene delivery. J Control Release 2002;82:189-212.
- Kim SC, Kim DW, Shim YH, Bang JS, Oh HS, Kim SW, et al. In vivo evaluation of polymeric micellar paclitaxel formulation: toxicity and efficacy. J Control Release 2001;72:191-202.
- Zhang X, Jackson J, Burt H. Development of amphiphilic diblock copolymers as micellar carriers of taxol. Int J Pharm 1996;132:195-206.
- Cho YW, Lee J, Lee SC, Huh KM, Park K. Hydrotropic agents for study of in vitro paclitaxel release from polymeric micelles. J Control Release 2004;97:249-57.
- Huh KM, Lee SC, Cho YW, Lee J, Jeong JH, Park K. Hydrotropic polymer micelle system for delivery of paclitaxel. J Control Release 2005;101:59-68.
- Grindel JM, Jaworski T, Piraner O, Emanuele RM, Balasubra-manian M. Distribution, metabolism, and excretion of a novel surface-active agent, purified poloxamer 188, in rats, dogs, and humans. J Pharm Sci 2002;91:1936-47.
- Batrakova EV, Li S, Li Y, Alakhov VY, Elmquist WF, Kabanov AV. Distribution kinetics of a micelle-forming block copolymer Pluronic P85. J Control Release 2004;100:389-97.
- Gao Z, Fain HD, Rapoport N. Ultrasound-enhanced tumor targeting of polymeric micellar drug carriers. Molecular Pharmaceutics 2004;1:317-30.
- Rowinsky EK, Eisenhauer EA, Chaudhry V, Arbuck SG, Done-hower RC. Clinical toxicities encountered with paclitaxel (Taxol). Semin Oncol 1993;20 Suppl 3:1-15.
- Rowinsky EK, Donehower RC. The clinical pharmacology of paclitaxel (Taxol). Semin Oncol 1993;20 Suppl 2:16-25.