Tetramethylpyrazine protected photoreceptor cells of rats by modulating nuclear translocation of NF-κB1
Introduction
Apoptosis has been described as the final common pathway to photoreceptor cell death in many eye diseases, such as age-related macular degeneration (AMD), retinal detachment and retinal light damage[1]. It has also been found to occur in the hereditary retinal degeneration, retinitis pigmentosa (RP)[2]. However, it is not obvious how the initial insult to the retina, which could be genetic, environmental, or both, drives the photoreceptor cell toward cell death. Therefore, an understanding of mechanisms that control apoptosis is integral to the treatment and prevention of retinal diseases. Recent studies have shown that light-induced retinal damage is regulated by the transcriptional factor of nuclear factor-κB (NF-κB)[3,4].
NF-κB was identified in 1986 as a nuclear factor that bound to an enhancer element of the immunoglobulin (Ig)κ light-chain gene and was believed to be specifically expressed in B cells[5]. It has recently been implicated in cellular apoptosis. Subunits of NF-κB, p50, and p65 (RelA) form a heterodimer that exhibits DNA binding potential. NF-κB typically resides in the cytoplasm bound to an inhibitory subunit, IκBα or IκBβ, which prevents its translocation to the nucleus[6]. NF-κB is constitutively active in several cell lines including THP-1 cells[7], thymocytes[8], neurons[9], and photoreceptor cells[3] and thus may be universally important to cell survival.
RP is not only a progressively recessive disease, but also a progressive dystrophia. Thus, we observed in vivo the retinoprotective effects of tetramethylpyrazine (TMP), which has the role of promoting blood circulation, on N-methyl-N-nitrosourea (MNU)-induced retinal damage and elucidated the involvement of NF-κB in the protection mechanism.
Materials and methods
Animals Six-week-old female Sprague-Dawley (SD) rats were purchased from Zhongshan Medical College, Sun Yat-sen University. Animals were maintained on a basal diet with free access to food and water and were housed in plastic cages in an air-conditioned room at 22±2 °C with a 12-h light/dark cycle.
Drugs and reagents MNU was purchased from Sigma (St Louis, MO, USA) and kept at -20 °C in the dark. The MNU solution was freshly prepared, dissolved in physiological saline, just before use. The in situ Cell Death Detection Kit was purchased from Roche Applied Science (Indianapolis, IN, USA). Mouse monoclonal Abs to rat NF-κB p65(sc-8008), IκBα (sc-1643), mouse β-actin (sc-8432) and HRP-conjugated antimouse IgG secondary Ab were purchased from Santa Cruz Biotechnology (Santa Cruz, CA, USA). The gel shift assay systems were purchased from Bio-Rad (Bio-Rad, CA, USA). The TMP injection was provided by Changzhou Pharmaceutical Factory (Changzhou, Jiangsu, China).
Morphometric analysis of retinal layers Animals were classified randomly into five groups as follows: (i), Control group (n=6); 47-day-old rats received a daily ip injection of physiological saline for 4 days. (ii), Model group (n=6); 47-day-old rats received a daily ip injection of physiological saline for 4 days. At 50 days of age, rats also received a single ip injection of 60 mg/kg body weight of MNU. (iii), TMP injection groups (n=18); rats were subdivided into 40 mg/kg, 80 mg/kg and 160 mg/kg groups. Drugs were injected ip into 47-day-old SD rats for 4 days, respectively. At 50 days of age, all rats received a single ip injection of 60 mg/kg MNU after TMP treatment for 12 h. All rats were killed 7 d after MNU or physiological saline treatment. Eyes were quickly removed and fixed overnight in 10% neutral buffered formalin. Tissues were processed routinely for paraffin embedding and stained with hematoxylin and eosin (HE). Each eye section was cut at the central part of the retina which was aligned parallel to the optic axis and nerve. Total retinal thickness (thickness from the internal limiting membrane to the pigment epithelium) as well as outer retinal thickness (thickness of the outer nuclear layer and photoreceptor layer) in the peripheral retina (average of approximately 400 mm from both sides of the ciliary body) and central retina (average of approximately 400 mm from both sides of the optic nerve) were measured. The HE specimens of the retina were prepared in a magnification of 40×10 and examined on an OLYMPUS BX 40 electron microscope (Carl Zeiss, Germany)[10].
In situ detection of apoptosis The treatment schedule was the same as detailed in the above method. All rats were killed 24 h after MNU or physiological saline treatment and both eyes were quickly removed. The TUNEL method was performed in the formalin-fixed tissue samples by using an apoptosis detection kit according to the manufacturer’s instructions[11]. The apoptotic index of the outer nuclear layer (number of fragmentation nuclei/number of whole photoreceptor cell nuclei×100) of retina was determined at twenty different fields in each section under ×400 magnification.
Preparation of cytoplasmic and nuclear extracts TMP injection at a dose of 160 mg/kg was injected ip into 47-day-old SD rats, once a day. At 50 days of age, rats were given a single ip injection of 60 mg/kg MNU. Control and model groups were the same as above. Each group had 4 rats. Rats were killed 12 and 24 h, and 3 and 7 d after MNU treatment, respectively. Nuclear and cytoplasmic extracts were prepared according to nuclear extract kits[7]. Whole retinas were isolated from the eyes of rats in each group, minced and homogenized. The pelleted cells were resuspended in 600 mL 1´Hypotonic buffer (10 mmol/L Hepes, pH 7.9, 1.5 mmol/L MgCl2, 10 mmol/L KCl, 10% glycerol, 1 mmol/L DTT, 0.5 mmol/L PMSF) and incubated for 15 min on ice. Then 30 mL detergent was added and centrifuged for 30 s at 14 000×g at 4 °C. The supernatants (cytoplasmic fraction) were collected and stored at -80 °C. The nuclear pellets were resuspended in 200 mL complete lysis buffer (20 mmol/L Hepes, pH 7.9, 400 mmol/L NaCl, 1.5 mmol/L MgCl2, 0.2 mmol/L EDTA, 20% glycerol, 1 mmol/L DTT, 0.5 mmol/L PMSF). Following a 30-min incubation on ice, the samples were centrifuged (14 000×g, 10 min, 4 °C), and the resulting supernatants (nuclear extracts) were transferred to a fresh microfuge tube and stored at -80 °C.
Western blot analysis Each protein sample (20 g) was denatured in boiling water for 10 min, loaded onto 10% SDS-PAGE and transferred to nitrocellulose membrane. Membranes were blocked overnight with a 5% (w/v) non-fat dry milk solution containing 10 mmol/L Tris-HCl, pH 8.0, 150 mmol/L NaCl and 0.1% Tween 20 (TBST) before incubation with primary antibodies (1 h for NF-κB p65, IκBα and β-actin anti-bodies). Primary antibodies were diluted in TBST (1:200 for NF-κB p65, IκBα and β-actin). After washing, the membranes were incubated for 1 h with horseradish peroxidase-labeled secondary antibody diluted 1:1000 in TBST, and the labeled proteins were detected using enhanced chemiluminescence reagents as described by the manufacturer (Amersham Pharmacia Biotech, Piscataway, NJ, USA). The results were expressed as AU (Darea·Ddensity). The ratio of arbitrary unit (AU, Darea·Ddensity) of target protein over β-actin was used for expressing the relative level of target protein.
Statistical analysis Data were expressed as mean± standard deviation and analyzed by SPSS 10.0 software. One to one comparisons among multiple-samples were made using one-way analysis of variance (ANOVA). P<0.05 was considered significant.
Results
Effect of TMP injection on retinal thickness of MNU-treated retina in SD rats Compared with retinas from the age-matched control SD rats (Figures 1A, 2A), peripheral or central retinas from MNU-treated animals showed thinning with selective loss of the photoreceptor cells 7 days after MNU injection (Figures 1B, 2B). While in rats pretreated with TMP injections, the total as well as outer retinal thickness of the peripheral retina was significantly greater than that of model rats in a dose-dependent manner (Figure 1C, 1D, 1E). However, no protective effects of TMP injections on MNU-induced central retinal damage were found (Figure 2C,2D, 2E). The results are shown in Table 1.
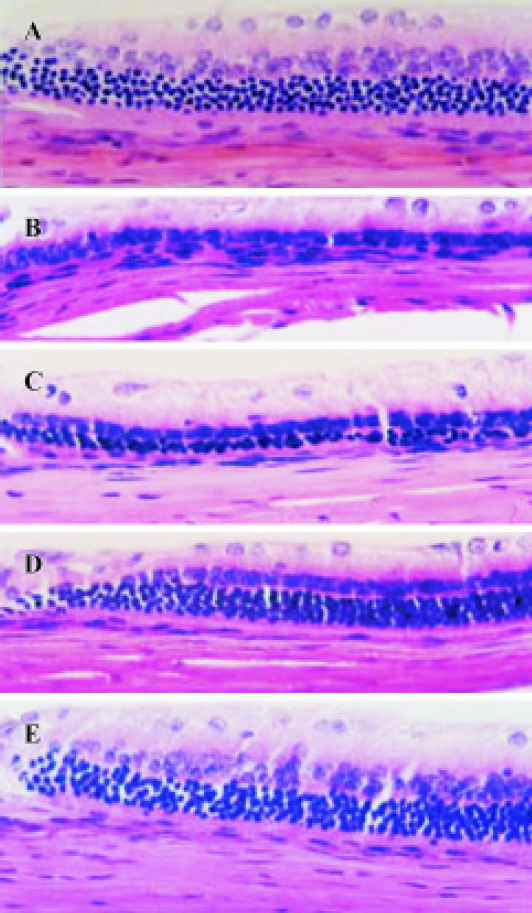
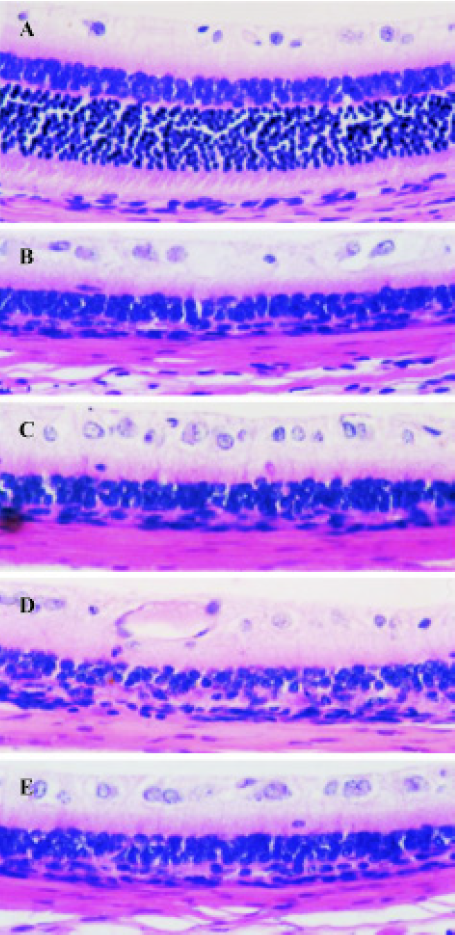
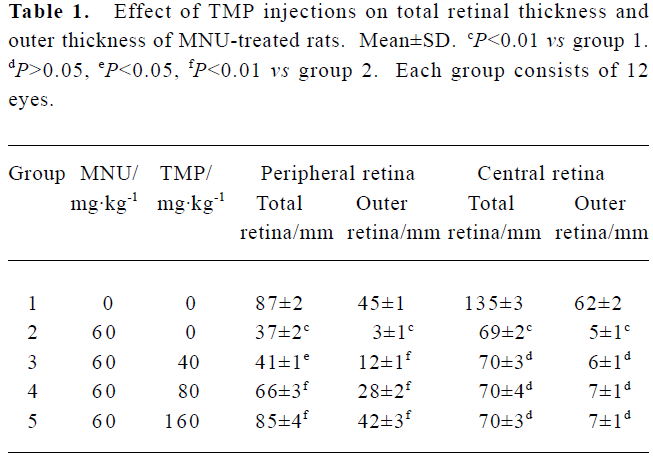
Full table
Effect of TMP injection on apoptotic index of photoreceptor cells induced by MNU in SD rats As TMP injection had no protective effect on damage to the central retina induced by MNU, we just detected the apoptotic index of photoreceptor cells in the peripheral retina. In MNU-treated rats, the 24 h post-MNU was (38.0±3.6)% in the peripheral retina. When TMP at doses of 40, 80 and 160 mg/kg were injected ip into 47-day-old SD rats for 4 d, the apoptotic indexes were significantly decreased to (30.0±3.0)% (P<0.05), (15.6±1.7)% (P<0.01), and (8.4±2.6)% (P<0.01), respectively.
Effect of TMP injection on NF-κB p65 and IκBα protein levels in MNU-induced retinal damage of SD rats As compared with the untreated control, low levels of p65 protein were detected in the nuclear fraction only after MNU treatment for 12 h and 24 h (Figure 3A). While for pretreatment with TMP injection of 160 mg/kg, the nuclear levels of p65 protein were significantly increased after MNU treatment for 24 h and at the 3rd and 7th days (P<0.01) (Figure 3A). The relative level of p65 protein was at its peak at 24 h and was 2.3-fold than that of the MNU-treated group (Figure 3B).
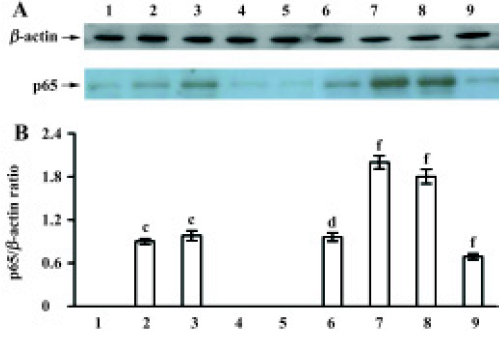
After MNU treatment for 12 h, the IκBα protein levels in the cytoplasm were markedly increased (P<0.05), and at a peak at 7 d (Figure 4A). In contrast to the MNU-treated group, TMP injection at 160 mg/kg time-dependently induced the down-regulation of cytoplasmic IκBα protein levels (Figure 4A). The relative level of IκBα protein in cytoplasm was 0.8-fold than that of the MNU-treated group at the 7th d of MNU treatment (P<0.05) (Figure 4B).
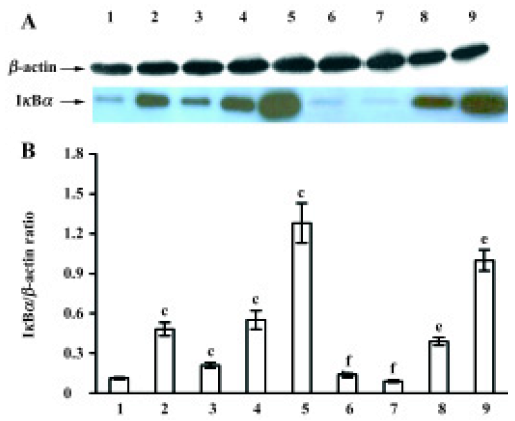
Discussion
The incidence of RP is approximately 1 in 4000, making it one of the major causes of blindness in humans. Although several genetic mutations have been implicated in RP, the final common pathway of the disease is apoptotic cell death of photoreceptor cells. Up to now, available treatments are of limited use in retarding this disease’s progression. RP is not only a progressively recessive disease, but also progressive dystrophia. The change of retinal vessels associated with RP is also a prominent and common phenomenon. It has been reported that the blood volume of choroidal vessels became decreased and the choroidal capillaries became atrophic in RP[12].
According to above viewpoints, inhibition of apoptosis and promoting blood circulation will have common applicability and feasibility. The manipulation of factors that influence the apoptotic pathway and the blood supply of choroidal vessels may be of potential value for RP therapy. Thus, we observed in vivo the retinoprotective effect of Chinese medicines, which have the role of promoting blood circulation by removing blood stasis and activating the collaterals, on MNU-induced photoreceptor cell apoptosis in SD rats. TMP, one of the alkaloids in Ligusticum wallichii Franch (L. wallichii), an active ingredient in Chuanxiong, dilates arterioles, decreases blood and plasma viscosity and improves microcirculation of blood supply of important organs such as the eyes, brain, heart and so on[13,14]. It has been widely used, especially in the treatment of patients with cerebrovascular diseases in China were its therapeutic actions have been manifested. The present study indicates that MNU-induced retinal damage in rats was partially suppressed by the ip administration of TMP injection through the inhibition of photoreceptor cell apoptosis. However, TMP injection had no protective effect on central retina damage induced by MNU. As in MNU-induced retinal injury, photoreceptor cell apoptosis was evident at 12 h and peaked at 24 h, followed by extensive photoreceptor cell loss 7 days later. Moreover, the apoptotic index was higher in central than peripheral retina after MNU treatment[10,11]. It indicated that the damage preceded from the central to peripheral retina, the posterior portion of the retina around the optic nerve was more severely damaged than the peripheral retina. Therefore, the scheme of administration was different from clinical administration. We observed the preventive effect of TMP on MNU-induced retinal damage and found that the rapid and extensive cell loss in the central retina was beyond the rescue capacity of TMP. In contrast to the MNU-induced retinal damage, human disease starts in the equatorial area. The mean duration of visual loss in RP patients was 17 years. While, our study showed that TMP injection had a markedly protective effect on slow cell loss. So, it was possible that TMP could prevent the progress of disease and protect the central vision in RP patients. In order to best provide the clinical basis of TMP injection, we would investigate whether TMP injection could rescue photoreceptor cells in retinal degeneration slow (rds) mice in a similar way to human RP.
In 1999, Krishnamoorthy et al reported that the presence of NF-κB RelA subunit in the nucleus was essential for protection of photoreceptor cells against apoptosis[3]. Crawford et al also reported that bcl-2 overexpression protected photooxidative stress-induced apoptosis of photoreceptor cells through NF-κB preservation[4]. So, we investigated NF-κB family members in the present study to elucidate the molecular mechanisms of the protective effect of TMP injection on MNU-induced photoreceptor cell apoptosis in SD rats. In the classical model of NF-κB activation, NF-κB p65 exists in the cytoplasm of unstimulated cells in an inactive form associated with the inhibitory protein, IκBα. Following cell stimulation by extracellular stimuli, IκBα is phosphorylated through a cascade of inducible protein kinase, and selectively degraded in cytoplasm by the proteasome. Rapid degradation of IκBα allows for nuclear translocation of NF-κB where it binds specific DNA motifs in the promoter/enhancer regions of target genes and activates transcription[15,16].
Under our experimental conditions, the results showed that the amounts of IκBα in the cytoplasm were significantly accumulated in a time-dependent manner in the MNU-treated retina. It indicated that increases of IκBα would block the nuclear translocation of NF-κB. Very low levels of p65 protein in the nuclear extracts were only detectable post-MNU for 12 and 24 h, and no p65 protein was found on the 3rd and 7th days after MNU treatment. But using pretreatment with TMP injection, the cytoplasmic IκBα protein levels were time-dependently down-regulated. So, more cytoplasmic p65 protein was in a free condition and then moved to the nucleus to regulate the anti- and pro-apoptotic gene expression. It was in accordance with our results that the nuclear levels of p65 protein were significantly increased in the TMP-treated group, and TMP injection markedly increased photoreceptor cells compared with the MNU-treated group. It was further demonstrated that the p65 subunit of NF-κB may be important for photoreceptor cell survival. Meanwhile, the severe damage of central retina induced by MNU was beyond the protective effect of TMP injection, we also found that the cytoplasmic IκBα protein was increased and nuclear p65 protein was decreased at the 7th day after MNU treatment.
TMP exerts a variety of effects through antilipid per-oxidation, inhibiting calcium overload, decreasing the activity of nitric oxide synthase and the expression of Fas protein, raising the ratio of Bcl-2/Fas protein and so on[17,18]. Therefore, TMP may also provide retinoprotection by multiple mechanisms. This needs to be further demonstrated. Although the factors that trigger or impede programmed cell death in photoreceptor cells have yet to be clarified, and the apoptotic cascade may differ between rats and humans, the present findings indicate that TMP can prevent MNU-induced photoreceptor cell apoptosis and may provide a basis for the clinical use of TMP to protect or rescue human RP patients.
References
- Abler AS, Chang CJ, Ful J, Tso MO, Lam TT. Photic injury triggers apoptosis of photoreceptor cells. Res Commun Mol Pathol Pharmacol 1996;92:177-89.
- van-Soest S, Westerveld A, de-Jong PT, Bleeker-Wagemakers EM, Bergen AA. Retinitis pigmentosa: defined from a molecular point of view. Surv Ophthalmol 1999;43:321-34.
- Krishnamoorthy RR, Crawford MJ, Chaturvedi MM, Jain SK, Aggarwal BB, Al-Ubaidi MR, et al. Photo-oxidative stress down-modulates the activity of nuclear factor-kappaB via involvement of caspase-1, leading to apoptosis of photoreceptor cells. J Biol Chem 1999;274:3734-43.
- Crawford MJ, Krishnamoorthy RR, Rudick VL, Collier RJ, Kapin M, Aggarwal BB, et al. Bcl-2 overexpression protects photooxidative stress-induced apoptosis of photoreceptor cells via NF-kappa B preservation. Biochem Biophys Res Commun 2001;281:1304-12.
- Sen R, Baltimore D. Inducibility of kappa immunoglobulin enhancer-binding protein NF-κB by a posttranslational mechanism. Cell 1986;47:921-8.
- Legrand-Poels S, Schoonbroodt S, Matroule JY, Piette J. NF-kappa B: an important transcription factor in photobiology. J Photochem Photobiol B 1998;45:1-8.
- Zhou JY, Tang FD, Mao GG, Bian RL. Effect of α-pinene on nuclear translocation of NF-κB in THP-1 cells. Acta Pharmacol Sin 2004;25:480-4.
- Souabni A, Cobaleda C, Schebesta M, Busslinger M. Pax5 promotes B lymphopoiesis and blocks T cell development by repressing Notch1. Immunity 2002;17:781-93.
- Bhakar AL, Tannis LL, Zeindler C, Russo MP, Jobin C, Park DS, et al. Constitutive nuclear factor-kappa B activity is required for central neuron survival. J Neurosci 2002;22:8466-75.
- Yoshizawa K, Nambu H, Yang J, Oishi Y, Senzaki H, Shikata N, et al. Mechanisms of photoreceptor cell apoptosis induced by N-methyl-N-nitrosourea in Sprague-Dawley rats. Lab Invest 1999;79:1359-67.
- Yang JN, Lin SC, Chen JM, Zhang Y, Hu SX. Photoreceptor cell apoptosis of rat’s retina induced by N-methyl-N-nitrosourea. Chin J Ocul Fundus Dis 2004;20:33-6.
- Zhao ZX, Guo XR, Li YS. Characteristics of retinitis pigmentosa in indocyanine green angiography. Chin J Ocul Fundus Dis 2001;17:26-9.
- Li XY, Yang LZ, Kang FY, Zhang SS, Li GB, Huo YM, et al. The effect of ligustrazaine on the microcirculation of rabbit eyes with continuous elevated IOP. Chin J Microcirc 2001;5:119-20.
- Feng GQ, Liu J, Fu RF, Feng GY, Weng SI. Protective effect of alprostadil combined with ligustrazine and Astragalus mem-branaceus on myocardial ischemia reperfusion injury in rats. Chin J New Drugs Clin Remedies 2001;20:3-6.
- He JK, Gu ZL, Fan PS. Inhibitory effects of prostaglandin A1 on apoptosis of rat cardiac microvascular endothelial cells was mediated by NF-κB. Acta Pharmacol Sin 2002;23:331-5.
- Karin M, Ben-Neriah Y. Phosphorylation meets ubiquitination: the control of NF-κB activity. Annu Rev Immunol 2000;18:621-63.
- Zhang Z, Wei T, Hou J, Li G, Yu S, Xin W. Tetramethylpyrazine scavenges superoxide anion and decreases nitric oxide production in human polymorphonuclear leukocytes. Life Sci 2003;72:2465-72.
- Lu YM, Wan FS, Tang SB. Effects of tetramethylpyrazine on expression of Bcl-2 and Fas proteins in myocardial injury induced by catecholamine in rats. Chin J Clin Pharmacol Ther 2004;9:197-200.