Regulating expressions of cyclin D1, pRb, and anti-cancer effects of deguelin on human Burkitt’s lymphoma Daudi cells in vitro1
Introduction
A large number of natural products have been evaluated as potential chemopreventive agents. Deguelin (Figure 1), a natural plant-derived rotenoid, most commonly used as an insecticide in Africa and South America, has been isolated from several plant species, including Mundulea sericea (Leguminosae). Its main active composition is rotenone, and it has very strong photodissociation[1]. The recent research has indicated that deguelin usually has a very strong antitumor function, and can suppress many kinds of tumors cell at nmol levels[1]. Notably, deguelin can suppress colon-cancer cell HT-29 growth[2], inhibit the growth of precancerous and cancerous lung cells, and induce premalignant and malignant human bronchial epithelial (HBE) cells apoptosis with no toxic effects on normal cells[3]. These recent experiments verified that deguelin could lead the cell cycle to block and induce apoptosis, however, its mechanism is not yet completely clear.
Burkitt’s lymphoma (also called small noncleaved cell lymphoma) is a type of on-Hodgkin’s lymphoma, a cancer in the lymphatic system. Despite recent advances in radio-therapy, chemotherapy, and stem cell transplant, the severe morbidity from lymphoma has not been improved[4]. Much effort has been focused on the discovery and development of new chemopreventive agents, especially agents targeted at mechanisms known to be involved in the process of carcinogenesis. Therefore, we have sought to identify novel agents that can prevent lymphoma carcinogenesis effectively but with minimal toxicity.
This study was designed to explore the mechanism of deguelin-induced apoptosis in Daudi cells. We studied deguelin on human Burkitt’s lymphoma Daudi cells in vitro and compared the cytotoxicities of deguelin on Daudi cells with human peripheral blood monocular cell (PBMC), and focused on the changes in the expression of cyclin D1 and phosphor-Rb, and analyzed its underlying mechanism.
Materials and methods
Drugs and reagents Degulin was purchased from the Sigma Chemical Company (St Louis, MO, USA) and initially dissolved in dimethylsulfoxide(Me2SO), and stoered at -20 °C, and thawed before use. MTT was purchased from Janssen Chimica Company (New Brunswick, NJ, USA). RPMI-1640 medium, Hoechst 33258, and Me2SO were purchased from Sigma Co. Anti-cyclin D1 and anti-phosphor Rb antibodies were purchased from Santa Cruz (California, USA). Chemiluminescence (ECL) reagent kits were purchased from Pierce Biotechnology, Inc (Rockford, IL, USA). Daudi cells line was obtained from China Center for Typical Culture Collection (Wuhan, China). PBMC were obtained by Ficoll-Hypaque density gradient centrifugation. All kinds of cells were grown in RPMI-1640 culture medium containing 10% fetal calf serum (FCS) and 2 mmol/L L-glutamine at 37 ºC in a 5% CO2 incubator.
MTT assay The antiproliferative effects of deguelin against different group cells were determined by the MTT dye uptake method. Briefly, the Daudi cells and PBMC (40 000 per well) were incubated in triplicate in a 96-well plate. Different concentrations of deguelin were added, and the final concentrations were 0, 5, 10, 20, 40, 80, and 160 nmol/L. The plates were in the presence or absence of the indicated test samples for 0, 24, 36, 48, 60, and 72 h. The group in maximal Me2SO dissolution concentration acted as the control group. Thereafter, 20 µL MTT solution (5 mg/mL in phosphate-buffered saline [PBS]) was added to each well. After 4 h at 37 °C, the supernatant was removed and 150 µL Me2SO was added. When the blue crystal was dissolved, the optical density (OD) was detected at 570 nm wavelength using a 96-well multiscanner autoreader (Biotech Instruments µQuant, New York, USA). The following formula was used: Cell proliferation(%)=[1–(OD of the experimental samples/OD of the control)]×100%.
Hoechst 33258 staining Deguelin-induced apoptosis was monitored by the extent of nuclear fragmentation. Nuclear fragmentation was visualized by Hoechst 33258 staining of apoptotic nuclei. The apoptotic cells were collected by centrifugation, washed with PBS and fixed in 4% paraformaldehyde for 20 min at room temperature. Sub-sequently, the cells were washed and resuspended in 20 µL PBS before deposition on poly lysine-coated coverslips and were left to adhere on cover slips for 30 min at room temperature and then the coverslips were washed twice with PBS. The adhered cells were then incubated with 0.1% Triton X-100 for 5 min at room temperature and rinsed with PBS three times. The coverslips were treated with Hoechst 33258 for 30 min at 37 °C, rinsed with PBS and mounted on slides with glycerol- PBS. The cells were viewed with an Olympus BH-2 fluorescence microscope (Tokyo, Japan).
Annexin V-PI assay The cells were collected and washed with PBS, followed by being resuspended in binding buffer (HEPES-NaOH 10 mmol/L, pH 7.4, NaCl 140 mmol/L, CaCl2 2.5 mmol/L). The samples were incubated with 5 µL Annexin-V in dark for 15 min, washed with binding buffer and resuspended in 1% formaldehyde in the binding buffer at 4 ºC for 30 min. After being washed with binding buffer again, the cells were stained with 500 µL PI (1 µg/ml, Sigma) for 15 min then measured on flow cytometry (Stanford, Becton Dickin-son, USA).
DNA content and cell cycle analysis Untreated and treated cells were collected, after cultured in the presence or absence of degulin for the indicated time, rinsed with PBS, and suspended in 75% ethanol at –20 ºC overnight. Fixed cells were centrifuged at 1200×g and washed with PBS twice. To detect DNA content, cells were contained in the dark with PI 50 mg/L and 0.1% RNAse A in 400 µL PBS at 25 ºC for 30 min. Stained cells were analyzed on FACSort (Becton Dickinson). The percentage of apoptotic cells was determined using the CellQuest software program.
Western blot analysis Lysates were prepared from 1×107 cells by dissolving cell pellets in 100 µL of lysis buffer Na2HPO4 pH 7.4 20 mmol/L, 150 mmol/L NaCl, 1% Triton X-100, 1% aprotinin, 1 mmol/L phenylmethylsulfonyl fluoride, 10 g/L leupeptin, 100 mmol/L NaF, and 2 mmol/L Na3VO4). Lysates were centrifuged at 18 000×g for 15 min and the supernatant was collected. Protein content was determined using a Bio-Rad protein assay (Bio-Rad Laboratories, Hercules, CA, USA). Sodium dodecylsulfate-polyacrylamide gel electrophoresis (SDS-PAGE) sample buffer (10 mmol/L Tris-HCl, pH 6.8, 2% SDS, 10% glycerol, 0.2 mol/L DTT) was added to the lysates. Lysates were heated to 100 ºC for 5 min, and 80 µg of protein was loaded into each well of a 10% SDS-PAGE gel. Resolved proteins were electrophoretically transferred to nitrocellulose and blocked with 5% non-fat milk, and the primary antibodies cyclin D1 and phosphor Rb (dilution 1:200), respectively. After overnight incubation at 4 °C the blots were washed, exposed to HRP-conjugated corresponding secondary antibodies for 1 h, and finally detected by ECL. Quantification of the bands was carried out using densitometric analysis software, Quantity One (Bio-Rad), and processed as described previously.
Statistical analysis All data were expressed as mean±SD using SPSS 10.0 for windows 98. Using linear t-tests for statistics analyses, P values of less than 0.01 or 0.05 were considered to be statistically significant.
Results
Effects of deguelin on proliferation of Daudi cells and PBMC Daudi cells and PBMC treated with deguelin for 0 h, 24 h, 36 h, 48 h, 60 h, and 72 h, respectively, resulted in the inhibition of cell proliferation in a dose- and time-dependent manner. The OD value of the deguelin-treated group decreased significantly compared with the untreated group and PBMC group. There are significant differences between the deguelin-treated and untreated group (Figure 2). It has also shown great difference between deguelin-treated and PBMC group (Figure 3). The IC50 value of 24 h on Daudi cell was 51.55 nmol/L, whereas the IC50 value of 24 h on PBMC was 1.63 µmol/L. The IC50 value of 24 h on PBMC was higher than Daudi cell group with great significance (P<0.01).
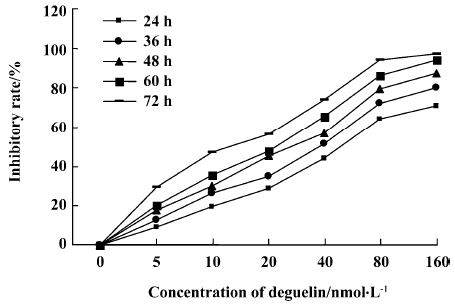
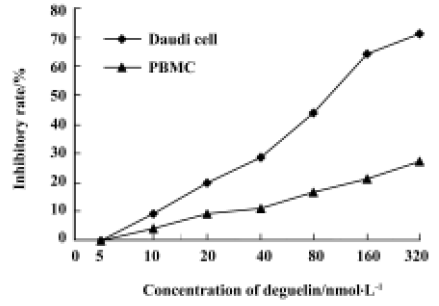
Hoechst 33258 staining We used Hoechest 33258 staining to investigate the changes in the nucleus of cells, and many apoptotic bodies containing nuclear fragments were found in deguelin-treated (40 nmol/L for 24 h) cells, but none in untreated cells. Arrows indicate apoptotic nuclear fragmentation (Figure 4). At the same time, cytoplasmic shrinkage was observed in cells cultured with deguelin.
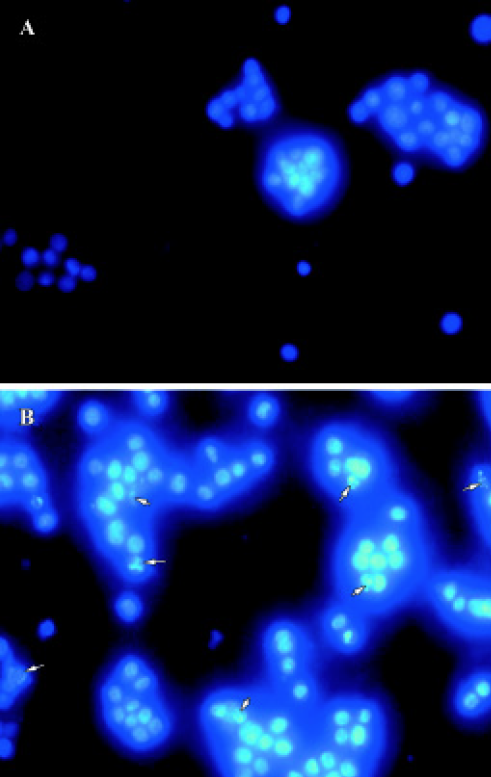
Annexin V-PI assay All untreated cells were Annexin-V negative, which meant no apoptosis occured. In contrast, when Daudi was treated with deguelin, apoptotic cells became Annexin-V positive and PI negative almost all the apoptosis occured in cells undergoing the G1-phase. With the increasing dose of deguelin, early apoptotic cells increased from (15.46%±0.62%) to (18.48%±2.98%) with 20 nmol/L and 40 nmol/L deguelin, respectively (Figure 5). It meant that deguelin could induce G1-phase specific apoptosis.
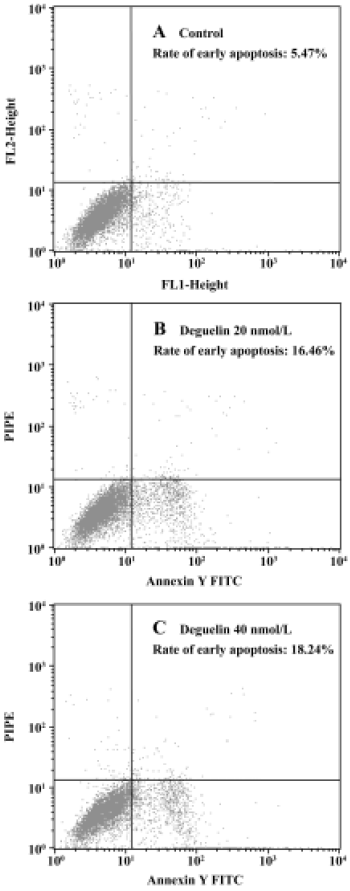
Cell cycle analysis Figure 6 illustrates changes in DNA content distribution treated with deguelin 0, 5, 10, 20, 40, and 80 nmol/L for 24 h. As the treatment dose increased, the percentage of cells in G1/G0 phase increased and S phase decreased accordingly. Treated with 0, 5, 10, 20, 40, and 80 nmol/L deguelin for 24 h, the rate of G1/G0 phase cells were increased by 37.34%, 40.91%, 44.69%, 52.45%, and 56.56% in a dose-dependent manner. Meanwhile, the rate of S phase cells were decreased by 37.72%, 35.97%, 34.91, 24.11, and 21.36% in a dose-dependent manner. It showed few changes in G2/M phase. These results illustrated that deguelin arrested the Daudi cells at G1/G0 phase in vitro.
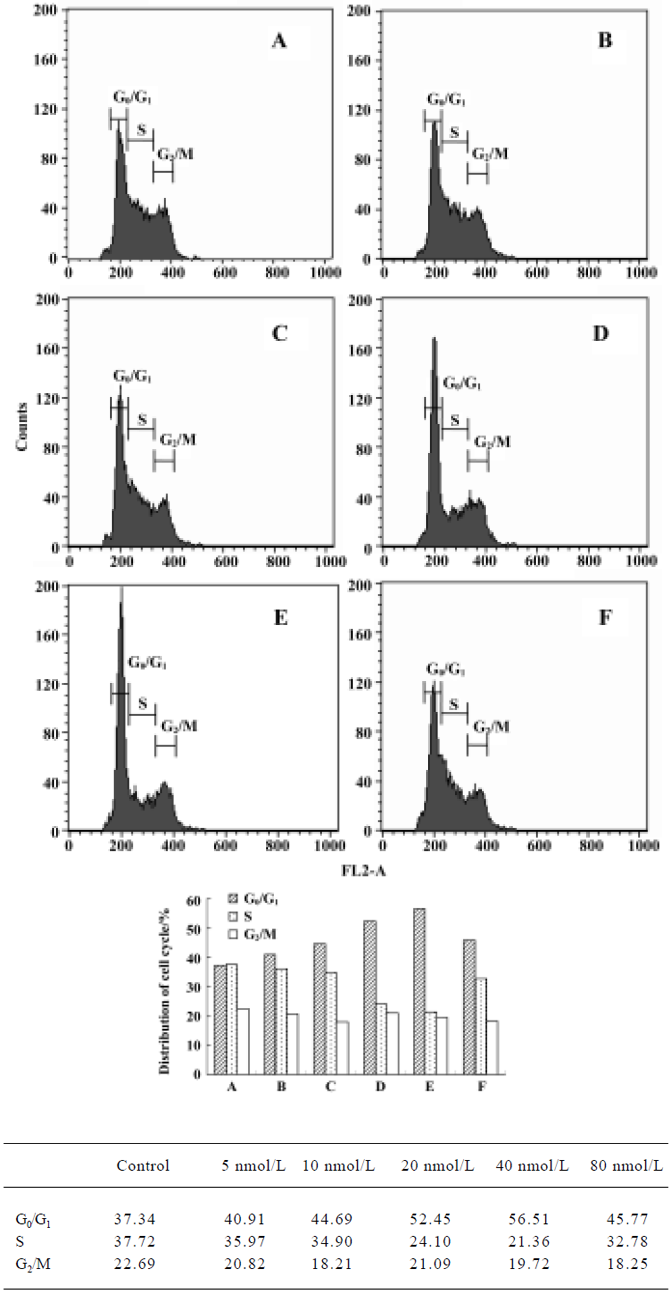
Cyclin D and pRb analysis by Western blot After treated with 0, 50, 100, 200, and 400 nmol/L deguelin for 24 h, both the expression of cyclin D1 and pRb were down-regulated dramatically in a dose-dependent manner. The OD values of cyclin D1 and pRb on Daudi cells were greatly significant compared with the control cells, and P values were less than 0.05 or 0.01, respectively (Figure 7).
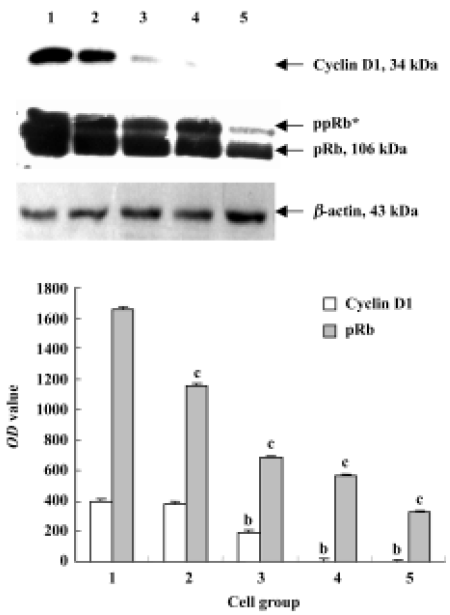
Discussion
Several natural compounds, especially plant products and dietary constituents, have been found to exhibit chemopre-ventive activities both in vitro and in vivo in model systems. Their mechanisms of action vary widely, with many suppressing cell growth or modulating cell differentiation and a few also inducing apoptosis. Rotenoids, which constitute a class of compounds from the flavonoid family, have chemopreventive activity, act by inhibiting NADH: ubiqui-none oxidoreductase activity, and by suppressing steady-state mRNA levels and enzymatic activity of 12-O-tetra-decanoylphorbol-13-acetate (TPA)-induced ornithine decarboxylase (ODC) activity[5]. One rotenoid, deguelin, has been isolated from several plant species, including Mundulea sericea (Leguminosae)[6]. Deguelin has been shown to have cancer-chemopreventive effects in models of skin, mammary, and lung tumorigenesis. Deguelin has been widely used as an insecticide in Africa, South Africa, and China[7].
This experiment also founded that deguelin could suppress Daudi cell selectively, and it had low toxicity on human peripheral blood monocular cells (PBMC). Deguelin-induced antiproliferative effects on Daudi cells, indicating that deguelin may have potential as they accumulated in the G0/G1 phase of the cell cycle and underwent apoptosis in a dose- and time-dependent manner. We found that deguelin decreased the expression of cyclin D1 and pRb in Daudi cells, suggesting that changes in the ratio of cyclin D1 and pRb might contribute to the apoptosis-promoting activity of deguelin in these cells. Cyclin D1 and pRb are important proteins for regulating cell cycle. Chun et al[4] found that deguelin could inhibit both premalignant and malignant HBE cells and these were more sensitive to deguelin than normal HBE cells. They also found deguelin increased the expression of Bax and decreased the expression of Bcl-2 in premalignant and malignant HBE cells, suggesting that changes in the ratio of Bax to Bcl-2 might contribute to the apoptosis-promoting activity of deguelin in these cells. This suggests that mechanisms of regulating cell cycle may contribute to the sensitivity of tumor cells to deguelin.
The results of Hoechest 33258 staining and Annexin V-PI assay showed that deguelin could induce Daudi cells apoptosis in a dose-dependent way. Arrows indicate apo-ptotic nuclear fragmentation. Apoptotic cells presented typical characteristics including the cell nuclear concentration, nucleus gathered by the core. With the increasing dose of deguelin, early apoptotic cells increased from 15.46%±0.62% to 18.48%±2.98% with 20 nmol/L and 40 nmol/L deguelin, respectively. The latest research materials indicate that deguelin can inhibit other tumor cell proliferation such as breast cancer, lung cancer, colon-cancer, and so on. Its underlying mechanism relates to inducing inhibition of cyclooxygenase-2 and extracellular signal-regulated kinase expression, participating in the phosphatidylinositol 3-kinase/Akt pathway[7–9].
The cell cycle is an ordered set of events, culminating in cell growth and division into two daughter cells. Non-dividing cells are not considered to be in the cell cycle. Severe defects in chromosomes block progression through the cell cycle, and can lead to cell suicide or apoptosis. In addition, cells have a finite lifespan, and at some point are no longer able to divide. Deguelin usually acts on different stages of the cell cycle of tumor cells and plays an antitumor role, and this may be one of its pharmacological mechanisms. In this report, Daudi cells were arrested in the G0/G1 phase mainly by deguelin, meanwhile the proportion of S phase was gradually reduced. After a different dose of deguelin for 24 h, cells in G0/G1 phase were most early influenced and increased gradually in a dose-dependent way with 40 nmol/L, and reached peak value. At the same time, cells in S phase were decreased gradually with 40 nmol/L resulting in the lowest value. The above data shows that deguelin usually regulates the G1/S checkpoint. Deguelin regulating G1/S check point was verified in other tumor cells. Chun et al[4] found that after treatment with 1×10-9mol/L deguelin on premalignant and malignant HBE cell, the rate of G0/G1 cells increased from 76.1% to 91.2%. At the same time, deguelin arrested premalignant and malignant HBE cells in G2/M phase that increased from 9.6% to 40.2%.
Rapidly developing technology in the field of molecular biology enables estimation of control mechanisms of cancer cells at the molecular level. Much interest has focused on the proteins participating in cell cycle control. The sequential transcriptional activation of cyclins, the regulatory subunits of cell-cycle-specific kinases, are thought to regulate progress through the cell cycle[10]. The orderly progression of dividing mammalian cells through the G1, S, G2, and M phases of the cell cycle is governed by a series of proteins called cyclins, which exert their effects by binding to and activating a series of specific cyclin-dependent kinases (CDK). Cyclins are therefore potential oncogenes. Cyclin D1 overexpression and/or amplification at its genomic locus, 11q13, are common features of several human cancers. The cyclin D1 gene, which acts at the mid-portion of the G1-S transition, is often overexpressed in human breast, colon, and squamous carcinomas, and several other types of cancer, and the cyclin E gene, which acts in late G1 is also over-expressed and dysregulated in a variety of human cancers[11]. In mechanistic studies we demonstrated that overexpression of cyclin D1 played a critical role in Burkitt lymphorma carcinogenesis. Therefore, cyclin D1 may be a useful biomar-ker in molecular epidemiology studies, and inhibitors of its function may be useful in both cancer chemo-prevention and therapy.
Retinoblastoma (Rb) is a rare tumor of the retina, associated with mutations of chromosome 13q14. The protein encoded by the Rb gene, pRb, normally plays a key role as a negative regulator of the G1/S transition in the cell cycle by binding the transcription factor E2F and preventing it from activating the transcription of genes required for the S phase[12]. pRB gene product nuclear phosphoprotein undergoes differential phosphorylation during the cell cycle[13]. A number of cellular proteins interact with hypophosphorylated pRb including: E2F transcription factor, several cyclins, RBP-1, RBP-2, c-myc, N-myc, and p46. During G1-phase, pRb is predominantly in a hypophosphorylated state and it becomes increasingly phosphorylated through the cell cycle until the end of mitosis when substantial dephosphorylation[14–16]. To further investigate the role of cyclin genes in mammary tumorigenesis, we analyzed the expression of cyclins D1 and pRb in Daudi cells by Western blot. In this study, deguelin was shown to down-regulate the expression of cyclin D1 and pRb on Daudi cells in a dose-dependent way. This incident was closely related to arrest in G0/G1. This shows that deguelin can regulate the function of molecule cyclin D1 and control cell cycle, reduce the expression of pRb, and realize its regulation and control function of G1/S checkpoints.
B-NHL plays an important role in blood system tumor. Though traditional chemotherapy and radiotherapy can achieve progress, the long-term result of treatment is not obvious [17]. We chose Burkkit’s lymphoma cell line Daudi cells as a research target. We found that deguelin could arrest Daudi cells in G0/G1 phase and induce apoptosis, and did not have any obvious poisonous function on the normal cell. As the source of deguelin is abundant and cheap, hopefully it will become a new-type of powerful antineoplastic medicine in treatment with B cells lymphoma.
Acknowledgements
Thanks to the Tumor Biology Laboratory, Center of Gynaecology, Tongji Hospital, Huazhong University of Science and Technology, China for offering relevant experimental facilities and technical support. We wish to particularly thank Prof Jian-feng ZHOU and Yun-ping LU for their guidance and help with the experiment.
References
- Udeani GO, Gerhauser C, Thomas CF, Moon RC, Kosmeder JW, Kinghorn AD, et al. Cancer chemopreventive activity mediated by deguelin, a naturally occurring rotenoid. Cancer Res 1997;57:3424-8.
- Lee HY, Suh YA, Kosmeder JW, Pezzuto JM, Hong WK, Kurie JM. Deguelin-induced inhibition of cyclooxygenase-2 expression in human bronchial epithelial cells. Clin Cancer Res 2004;10:1074-9.
- Chun KH, Kosmeder JW 2nd, Sun S, Pezzuto JM, Lotan R, Hong WK, et al. Effects of deguelin on the phosphatidylinositol 3-kinase/Akt pathway and apoptosis in premalignant human bronchial epithelial cells. J Natl Cancer Inst 2003;95:291-302.
- Weber AL, Rahemtullah A, Ferry JA. Hodgkin and non-Hodgkin lymphoma of the head and neck: clinical, pathologic, and imaging evaluation. Neuroimaging Clin N Am 2003;13:371-92.
- Murillo G, Salti GI, Kosmeder JW 2nd, Pezzuto JM, Mehta RG. Deguelin inhibits the growth of colon cancer cells through the induction of apoptosis and cell cycle arrest. Eur J Cancer 2002;38:2446-54.
- Evan GI, Vousden KH. Proliferation, cell cycle and apoptosis in cancer. Nature 2001;411:342-8.
- Ormerod MG. Investigating the relationship between the cell cycle and apoptosis using flow cytometry. J Immunol Methods 2002;265:73-80.
- Izevbigie EB, Bryant JL, Walker A. A novel natural inhibitor of extracellular signal-regulated kinases and human breast cancer cell growth. Exp Biol Med 2004;229:163-9.
- Sun SY, Hail N Jr, Lotan R. Apoptosis as a novel target for cancer chemoprevention. J Natl Cancer Inst 2004;96:662-72.
- Mitselou A, Ioachim E, Zagorianakou N, Kitsiou E, Vougiouklakis T, Agnantis NJ. Expression of the cell-cycle regulatory proteins (cyclins D1 and E) in endometrial carcinomas: correlations with hormone receptor status, proliferating indices, tumor suppressor gene products (p53, pRb), and clinicopathological parameters. Eur J Gynaecol Oncol 2004;25:719-24.
- Feakins RM, Nickols CD, Bidd H, Walton SJ. Abnormal expression of pRb, p16, and cyclin D1 in gastric adenocarcinoma and its lymph node metastases: relationship with pathological features and survival. Hum Pathol 2003;34:1276-82.
- Weinstein IB. Disorders in cell circuitry during multistage carcinogenesis: the role of homeostasis. Carcinogenesis 2000;21:857-64.
- Solomon DA, Wang Y, Fox SR, Lambeck TC, Giesting S, Lan Z, et al. Cyclin D1 splice variants. Differential effects on localiza-tion, RB phosphorylation, and cellular transformation. J Biol Chem 2003;278:30339-47.
- Shim MJ, Kim HJ, Yang SJ, Lee IS, Choi HI, Kim TU. Arsenic trioxide induces apoptosis in chronic myelogenous Leukemia K562 cells: possible involvement of p38 MAP kinase. J Biochem Mol Biol 2002;35:377-83.
- Chen XC, Chen LM, Zhu YG, Fang F, Zhou YC, Zhao CH. Involvement of CDK4, pRB, and E2F1 in ginsenoside Rg1 protecting rat cortical neurons from β-amyloid-induced apoptosis. Acta Pharmacol Sin 2003;24:1259-64.
- Pietenpol JA, Stewart ZA. Cell cycle checkpoint signaling: cell cycle arrest versus apoptosis. Toxicology 2002;181–182:475-81.
- Liu HL, Chen Y, Cui GH, Zhou JF. Curcumin, a potent anti-tumor reagent, is a novel histone deacetylase inhibitor regulating B-NHL cell line Raji proliferation effect. Acta Pharmacol Sin 2005;26:603-9.