Taurine inhibits ischemia/reperfusion-induced compartment syndrome in rabbits1
Introduction
Compartment syndrome is characterized by increased tissue hydrostatic pressure within a closed fascial space and by secondary compromise of skeletal-muscle capillary perfusion, with resultant ischemia, tissue necrosis and potential systemic toxicity[1]. The exact mechanisms of compartment syndrome are poorly understood; thus, effective treatments are deficient. The surgical management of compartment syndrome has changed little in the 70 years since fasciotomy was first described. Although effective, fascio-tomy increases morbidity, length of hospitalization and patient suffering, and may require subsequent skin grafting. Prevention of compartment syndrome by pharmacological methods, therefore, is an attractive prospect.
Ischemia/reperfusion (I/R) injury is one of the main factors contributing to increased anterior compartment pressure (ACP) and tissue necrosis after trauma. I/R disturbs oxygen-derived free radical metabolism and induces lipid peroxidation injury, which is considered to be the most important mechanism of I/R injury pathogenesis[2]. Recently, more attention has been paid to the application of free radical scavengers and cytoprotective agents[3,4].
Taurine (2-aminoethane sulfonic acid), a sulfur-containing amino acid derived from the metabolism of methionine, is the most abundant intracellular amino acid in humans and is implicated in numerous biological and physiological functions. Taurine is not used in protein synthesis but rather is found free or in simple peptides. The diet of healthy individuals is the major source of taurine, although in the presence of vitamin B6, taurine is also synthesized from methionine and cysteine. Taurine has been considered to be a strong endogenous cytoprotective agent. Its bioactions include inhibition of lipid peroxidation, detoxification, membrane stabilization, osmoregulation, modulation of cellular calcium levels, and it possibly acts physiologically as a trap for hypochlorous acid (HOCl). Exogenous administration of taurine has a preventive and therapeutic effect on tissue I/R injuries to the heart[5], liver[6], kidney[7] and hind limb muscles[8]. To explore the possibility of taurine therapy for compartment syndrome, we observed the alterations in muscular taurine content in the hind limbs of rabbits with I/R-induced compartment syndrome and the therapeutic effects of supplemental taurine on anterior compartment pressure and tissue damage.
Materials and methods
Reagents Taurine, triphenyltetrazolium chloride (TTC), sulphosalicylic acid, 3,3',5,5'-tetramethylbenzidine (TMB), thiobarbituric acid and 1,1,3,3-treaethyloxypropane were purchased from Sigma Company (St Louis, MO, USA). Other chemicals and reagents were of analytical purity.
Animals White rabbits weighing 1.5 kg to 2.0 kg were purchased from the Animal Center, Health Science Center, Peking University (Beijing, China). All animal experiments in this study were carried out with the approval of the Animal Care Committee of the First Hospital, Peking University, in accordance with the Chinese Council on Animal Care Guidelines. The rabbits were divided randomly into a taurine-treated group and a control group.
Preparation of ischemia-induced compartment syndrome model[9]. Briefly, white rabbits were anesthetized with intramuscular ketamine HCl (40 mg/kg) and acepromazine maleate (1 mg/kg) supplemented with 0.5% lidocaine HCl. A PE-50 tube filled with 0.9% NaCl and 10 kIU/L heparin was inserted into the right common carotid artery through a neck midline incision. The mean arterial blood pressure (MABP) and heart rate (HR) were recorded on a microcomputer-controlled physiological polygraph (PowerLab, 4s, Castle Hilllo-cation, Australia). A venous catheter was inserted into the right external jugular vein for collecting blood. A lower midline incision was made and extended across the groin. The dissection was carried caudally to the popliteal arteries. All arterial branches of the aorta, including the internal iliac arteries, were isolated, ligated with 4-0 silk sutures, and divided. The right hind limb was used for tibialis anterior muscle sampling for determination of TTC levels. The left hind limb was preserved for monitoring ACP. Ten minutes before ligation of both femoral arteries, animals given systemic infusions of heparin (100 IU/kg) to prevent aortic thrombosis. Just before femoral artery ligation, a full-thickness tibialis anterior muscle sample of the right hind limb was obtained to determine content of TTC, malondialdehyde and conjugated diene (CD), and venous blood was collected for assay of plasma malondialdehyde and CD content. After arterial ligation, the wounds were closed in a single layer with 3-0 silk sutures. The animals were allowed to recover and placed individually in a padded heated pen.
At the completion of the 7 h ischemic period, the animals were re-anesthetized and the lower midline incision and leg wounds were opened. Circulation between the proximal and the distal femoral arteries was restored by interposing a heparin-flushed polyethylene (PE 200) shunt. Five minutes before shunt placement, the animals received intravenous injections of heparin (100 IU/kg). Just before re-establishment of arterial flow, samples of tibialis anterior muscle were again obtained from the right hind limb. The pressure within the anterior muscle compartment of the left hind limb was measured with the use of the slit catheter technique, the catheter being connected to a saline-filled central venous pressure manometer[10]. Compartment pressure was monitored continuously and recorded at 1 h and 2 h of reperfusion. Beginning 10 min before shunt placement, experimental animals received 20 min intravenous infusions of taurine (1 g/kg in 8 mL normal saline) and the control group received 8 mL/kg normal saline only. Lactated Ringer’s solution (10 mL·kg-1·h-1) was infused via an ear vein throughout each experiment. At the completion of each experiment, animals were humanely killed via lethal urethane injection.
Measurement of taurine content in plasma and gastrocnemius muscle Gastrocnemius muscle samples (0.2 g) were homogenized in 5 volumes of normal saline. The homogenate was mixed with 5 volumes of 10% sulphosalicylic acid and centrifuged at 1000×g for 10 min at 4 oC. Plasma was obtained from collected blood by centrifugation at 2500×g for 10 min at 4 ºC. Taurine content in the tissue supernatant and plasma was determined by use of a high-performance amino acid analyzer (Hitachi Model 835, Hitachi, Japan).
Assay for TTC content and dry:wet ratios of tibialis anterior muscle The details of reduced TTC content as an estimate of oxidative metabolism have been reported by Belkin et al[11]. A spectrophotometric standard curve was constructed before each experiment. The TTC assay was repeated in duplicate at each of the experimental phases. In addition, at these intervals, minced samples of tibialis anterior muscle were weighed and allowed to dry at 90 ºC for 24 h. Samples were then re-weighed, and the dry weight: wet weight ratio value was calculated. These determinations were also carried out in duplicate.
Measurement of gastrocnemius muscle myeloperoxidase activity[12] Tissue myeloperoxidase activity was assessed by measuring the H2O2-dependent oxidation of TMB. The reactive mixture contained 2.1 mL of buffer-based (0.1 mol/L potassium phosphate buffer, pH 5.4) solution of H2O2 (0.1 mmol/L), 0.6 mL of the same buffer and 0.1 mL of TMB (1.6 mmol/L). The reaction was started by adding 200 µL of extract of tissue (at a protein concentration of 0.20 g/L), and the absorbance was measured every minute for 4 min. One unit of enzyme activity was defined as the amount of myeloperoxidase present that caused a change in absorbance of 1.0/min at 655 nm.
Assay of plasma lactate dehydrogenase (LDH) activity and content of malondialdehyde and CD in muscle and plasma Plasma LDH activity was measured by use of an automatic biochemistry analyzer. Malondialdehyde content was measured by use of the thiobarbituric acid reaction. Standard malondialdehyde was prepared by acid hydrolysis of 1,1,3,3-treaethyloxypropane[13]. CD content was determined according to the method reported by Waller et al[14].
Incubation of muscle slices with oxidative stress in vitro The gastrocnemius were taken from another 6 normal anesthetized rabbits, and tissue slices (3 µm) were prepared. The 50 mg of prepared muscle slices was incubated in 1 mL of Krebs-Henseleit buffer at 37 oC, equilibrated with 95% O2 and 5% CO2. The muscular oxidative stress was induced in vitro by adding three exogenous free radical-generating systems (FRGS): 400 µmol/L HOCL, 1.0 mmol/L hydrogen peroxide (H2O2), or an enzymatic system, composed of 20 IU/L of xanthine oxidase (XO) and its substrate xanthine (Xn, 0.5 mol/L). In each group, taurine (0, 5, 10, and 20 mmol/L)[15] was added. At the end of the incubation (10 min and 20 min, respectively), tissue slices were washed 3 times with ice-cold Krebs-Henseleit buffer, and homogenized for malondialdehyde determination.
Statistical analysis The data are expressed as mean±SD. Statistical analyses comparing multiple variables were carried out using ANOVA with the Student-Newman-Keuls test. For comparisons between 2 variables, the unpaired Student’s t-test was used. P<0.05 was considered statistically significant.
Results
Ischemia/reperfusion-induced compartment syndrome in rabbits After 7 h of ischemia, the MABP, HR, ACP, muscular dry:wet ratio, taurine content and plasma LDH activity remained unchanged, but the TTC level was reduced by 80% (P<0.01). Compared with 7 h ischemia, 2 h reperfusion resulted in decreased MABP and HR (P<0.01); however, ACP increased 4.5-fold (P<0.01) (Figure 1) and plasma LDH activity increased 2.5-fold (P<0.01). Muscles showed edema, the dry:wet ratio decreased by 14%, and taurine content was reduced by 33% in gastrocnemius muscle, but increased by 37% in plasma. The muscular TTC level was not further altered from that after ischemia (Table 1).
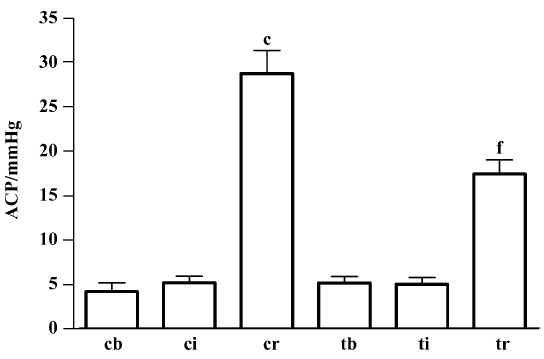
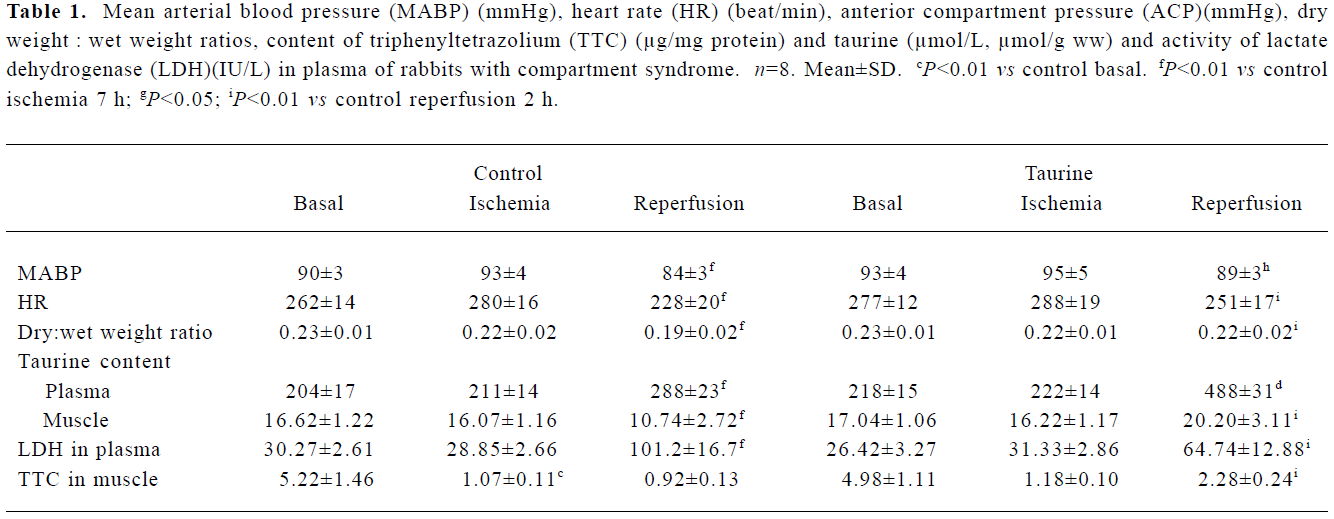
Full table
Treatment with taurine inhibited skeletal muscle I/R injury After ischemia, the values of various parameters were not statistically different between the control and taurine groups. At 2 h reperfusion, the administration of taurine before reperfusion resulted in a stable MABP and HR, the values being higher than those in the control group (P<0.01). The ACP was lowered by 39% (P<0.01). The muscular dry:wet weight ratio increased by 16%. Tissue taurine content was elevated by 88%, but plasma LDH activity was decreased by 36% (P<0.01). Muscular TTC content was 1.5-fold higher than controls (Table 1).
Treatment with taurine inhibited muscular myeloperoxi-dase activity induced by I/R After 7 h of ischemia, the gastrocnemius muscle myeloperoxidase activity remained unchanged. However, 2 h reperfusion resulted in a significant 3.5-fold increase in gastrocnemius muscle myeloperoxidase activity (P<0.01). Administration of taurine before reperfusion inhibited the myeloperoxidase activity by 48% compared with the control group (P<0.01, Figure 2).
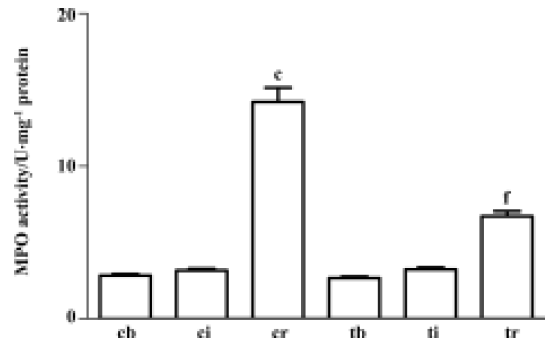
Supplementation of taurine attenuated lipid-peroxida-tion injury induced by I/R Ischemia (7 h) alone did not significantly change the levels of plasma and muscular lipid peroxidation products from those before ischemia (all P>0.05). After 2 h of reperfusion, the control group rabbits showed a significant increase in malondialdehyde and CD content in plasma and tibialis anterior muscle compared with before reperfusion (all P<0.01). The taurine group showed an inhibited formation of lipid per-oxidation products: decreased plasma and muscle malo-ndialdehyde content, by 38% and 22% (P<0.01), and decreased CD content, by 23% and 30% (P<0.01), respec-tively, compared with the control group (Table 2).
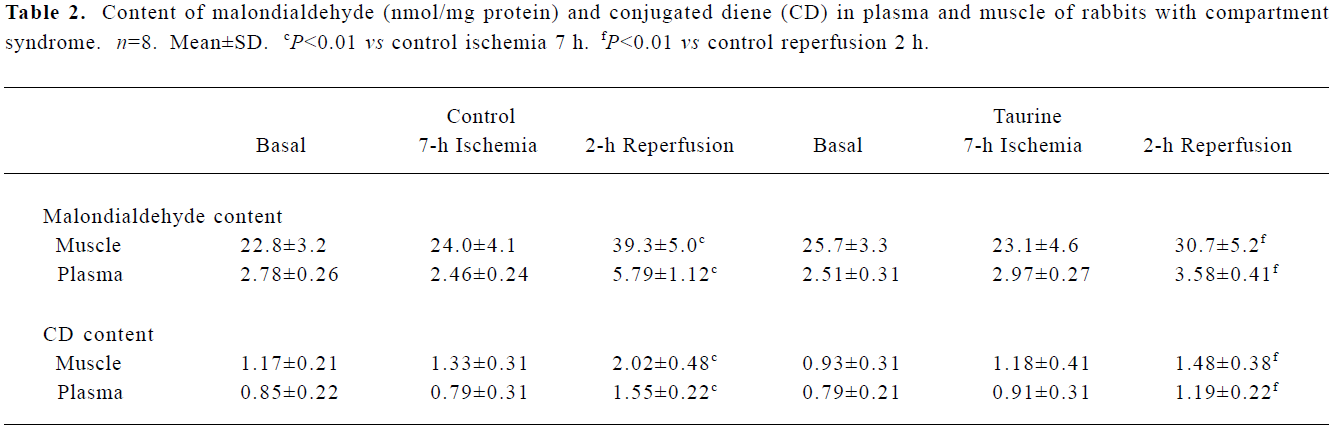
Full table
Protective effects of taurine against in vitro FRGS-induced oxidative stress In our experiment the concentrations of three FRGS all induced lipid peroxidation, and malondialdehyde content increased to a resemble level (P<0.01). Taurine decreased malondialdehyde content in a concentration-dependent manner in tissue slices incubated with H2O2 for 20 min (P<0.01) and 20 mmol/L taurine slightly decreased malondialdehyde content in tissue slices incubated with H2O2 for 10 min (P<0.05). Taurine did not change malondialdehyde content when incubated with XO and Xn (produced O2–) (P>0.05). In muscle slices incubated with HOCl, taurine decreased malondialdehyde content in a concentration- and time-dependent manner (P<0.01). The effect was stronger than that against H2O2 and O2– (Figure 3, 4).
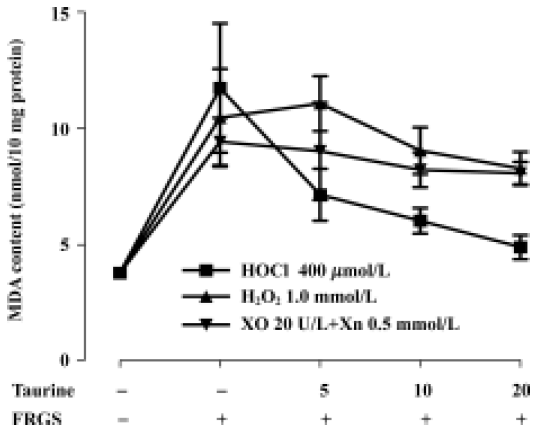
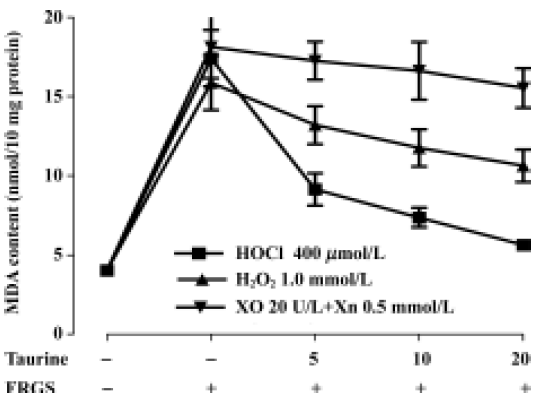
Discussion
A realistic, working definition of compartment syndrome is skeletal muscle injury that leads to partial or total destruction of the integrity of cell walls, with partial or total release of intracellular contents into the interstitial fluid space. Once in the interstitial fluid, these contents change the osmotic gradient of the tissues, with a subsequent shift of fluid from the circulation to the injured tissues, increasing the pressure within muscles encased in fascial sheaths. In compartment syndrome, swelling caused by the osmotic gradient increases the local pressure above the systolic pressure and hence suppresses blood flow within the muscular anatomic unit. This process leads to prolonged ischemia and further cell death with the additional release of intracellular contents.
The exact mechanism of compartment syndrome is not fully understood. Perler et al[16] reported that free radical-mediated reperfusion injury was the most important factor in compartment syndrome. Ablating free radicals by scavenging superoxide radicals at reperfusion with superoxide dismutase or by blocking secondary hydroxyl radical formation with deferoxamine significantly ameliorated the increased compartment pressure and improved muscle I/R injury. Oxidative stress can lead to compartment syndrome, as ischemia and hypoxia, reperfusion-induced alteration of intracellular osmostasis, induced cellular swelling, Ca2+ overload, decreased stability of cell membranes and leaking cytosolic enzymes.
Taurine is a strong endogenous cytoprotective agent and has multiple functions such as in membrane stabilization, detoxification, antioxidation, osmoregulation and calcium modulation[17]. Because taurine has protective effects in I/R injury in various organs[5–7], we investigated its possible effect in protecting skeletal muscle against I/R-induced compartment syndrome.
We chose the rabbit hind-limb model of compartment syndrome because the fascial compartments of rabbits are anatomically similar to those of humans. The elevation in ACP after 2 h of reperfusion was dramatic. The tissue showed edema, and the intracellular contents leaked into the extracellular space. TTC-level reduction, an indirect measure of oxidative metabolism, was decreased during ischemia and reperfusion and indicated that muscle viability was reduced. These observations have been reported in the literature[9]. During reperfusion, the taurine content of the gastrocnemius muscle was significantly reduced but that in plasma increased, which suggests increased release of taurine content out of the tissue. Administration of taurine before reestablishment of arterial flow increased tissue taurine content and resolved features of the compartment syndrome: MABP and HR were restored, ACP declined, and muscular edema and necrosis were relieved. Zhang et al[8] reported that taurine improved rat hind-limb I/R injury induced by a tourniquet through inhibiting lipid peroxidation. In this study, we found that malondialdehyde and CD levels, the final products of lipid peroxidation, were elevated during reperfusion, but pretreatment with taurine effectively inhibited the formation of lipid-peroxidation products, which suggests that taurine could be effective in ameliorating compartment syndrome. To illustrate the pathway by which taurine inhibited lipid peroxidation, we observed myelo-peroxidase activity in gastrocnemius muscle from rabbits with I/R injury. Muscular myeloperoxidase activity increased significantly showed that neutrophil infiltrated and HOCl induced oxidative stress injury during I/R. The results from these in vitro studies further confirmed that taurine could reduce the level of oxidative injury caused by HOCl specifically.
Taurine is a sulfur-containing amino acid, the most abundant free amino acid in excitable tissues and cells, including those in nerves and muscles. Experimental data reveals that many functions of taurine, such as membrane stabilization, osmoregulation and calcium modulation, could be considered to be beneficial for compartment syndrome. Transport of taurine across cell membranes is one of the major steps in its physiological roles. For example, the biophysical and biochemical properties of taurine make it an excellent candidate for osmoregulation. During hyper-osmolar conditions, the increase in cell taurine content appears to be achieved primarily by active transport of taurine into the cell, while under hypo-osmolar conditions, a decrease in intracellular taurine content is a consequence of a marked stimulation in taurine efflux, which protects cells from dehydration with edema[18]. Taurine regulates the metabolism of phospholipid, the main content of the cellular membrane, and directly affects the stability of the membrane. In addition, taurine maintains the homeostasis of calcium by regulating the interaction between Ca2+ and the membrane[19].
In addition, taurine is an antioxidative agent and prevents injury from lipid peroxidation. However, the mechanism by which it does this is unclear. In the present study, muscular myeloperoxidase activity was increased significantly in I/R-induced compartment syndrome, which suggested that HOCl-induced oxidative stress might play an important role in I/R-induced compartment syndrome. Aneja et al[20] reported that extensive I/R-induced tissue injury was associated with neutrophil infiltration and myeloperoxidase activity enhancement. Myeloperoxidase is a major neutrophil protein and is also present in monocytes. In neutrophils, it is stored in azurophilic granules and released during phagocytosis. It is a heme enzyme that uses the superoxide and hydrogen peroxide generated by the neutrophil oxidative burst to produce hypochlorous acid and other reactive oxidants. The role of HOCl is well established in tissue damage associated with reperfusion injury. HOCl is a major oxidant produced by neutrophils and monocytes, via the myeloperoxidase-catalyzed oxidation of chloride by hydrogen peroxide. HOCl is a potent oxidant capable of damaging host tissue during inflammation[21]. The strong oxidizing species HOCl plays a highly significant role in the bactericidal function of the neutrophil. However, inappropriate and/or excessive activation of neutrophils leads to oxidative stress and collateral damage to surrounding tissues.
As an antioxidant, taurine could effectively antagonize the toxic effect of HOCl. More recent information has revealed that taurine could interact with peroxide anions to form stable products such as taurine chloramine (TauCl). TauCl is formed through the sequestration of taurine with HOCl and has been found to be an exceptionally stable and long-lived compound with cytoprotective properties due to its ability to preserve cellular function in response to physiological stress[19]. In in vitro studies, taurine greatly inhibited lipid peroxidation induced by OCl–, indicating the important protective role of taurine against OCl– attack[22]. It is reasonable that taurine ameliorates ACP in compartment syndrome induced by I/R at least in part through scavenging HOCl and attenuating lipid peroxidation.
At present, the only approach to relieving compartment syndrome is surgical fasciotomy to allow decompression of the elevated intracompartmental pressure, thereby facilitating microvascular perfusion. Although highly effective, fasciotomy is an imperfect treatment, so effective pharmacological approaches to treat compartment syndrome are required. Taurine is an endogenous substance in the body; it is therefore not associated with toxicity or drug dependence, and is well tolerated when used clinically[23]. It is reasonable to suggest that the clinical application of taurine could be a new strategy for the prevention and treatment of compartment syndrome.
References
- Mubarek SJ, Hargens AR. Acute compartment syndromes. Surg Clin North Am 1983;63:539-65.
- Timoshin AA, Lakomkin VL, Ruuge EK. Effect of a series of brief cycles of ischemia and reperfusion on free radical centers of isolated rat myocardial tissue. Biofizika 1998;43:134-8.
- Van Haaften RI, Haenen GR, Evelo CT, Bast A. Effect of vitamin E on glutathione-dependent enzymes. Drug Metab Rev 2003;35:215-53.
- Dogru Abbasoglu S, Kanbagli O, Balkan J, Cevikbas U, Aykac-Toker G, Uysal M. The protective effect of taurine against thioacetamide hepatotoxicity of rats. Hum Exp Toxicol 2001;20:23-7.
- Oz E, Erbas D, Gelir E, Aricioglu A. Taurine and calcium interaction in protection of myocardium exposed to ischemic reperfusion injury. Gen-Pharmacol 1999;33:137-41.
- Wettstein M, Haussinger D. Taurine attenuates cold ischemia-reoxygenation injury in rat liver. Transplantation 2000;69:2290-6.
- Michalk DV, Hoffmann B, Minor T. Taurine reduces renal ischemia/reperfusion injury in the rat. Adv Exp Med Biol 2003;526:49-56.
- Zhang LY, Dong SY, Xu J, Yu F, Pang YZ, Tang CS, et al. Effect of taurine on rats with tourniquet shock. China J Pathphysiol 1993;9:208.
- Hakaim AG, Corsetti R, Cho S. The pentafraction of hydroxy-ethyl starch inhibits ischemia-induced compartement syndrome. J Trauma 1994;37:18-21.
- Rorabeck CH, Castle GSP, Hardie R, Logan J. Compartment pressure measurements: an experimental investigation using the slit catheter. J Trauma 1981;21:446-9.
- Belkin M, Brown RD, Wright JC. A new quantitative spectrophoto-metric assay for ischemia-reperfusion injury in skeletal muscle. Am J Surg 1988;156:83-8.
- Naito Y, Yoshikawa T, Matsuyama K, Yagi N, Arai M, Nakamura Y, et al. Neutrophils, lipid peroxidation, and nitric oxide in gastric reperfusion injury in rats. Free Radic Biol Med 1998;24:494-502.
- Skawa T, Matashushita S. Thiobarbituric acid test for detecting lipid peroxide. Lipids 1979;14:401-6.
- Waller RL, Recknagel RO. Determination of lipid conjugated dienes with tetracyanoethylene-14C: significance for study of the pathology of lipid peroxidation. Lipids 1977;12:914-21.
- Chang L, Xu JX, Zhao J, Pang YZ, Tang CS, Qi YF. Taurine antagonized oxidative stress injury induced by homocysteine in rat vascular smooth muscle cells. Acta Pharmacol Sin 2004;25:341-6.
- Perler BA, Tohmeh AG, Buckley GB. Inhibition of the compartment syndrome by ablation of free radical mediated reperfusion injury. Surgery 1990;108:40-7.
- Huxtable RJ. Physiological actions of taurine. Physiol Rev 1992;72:101-63.
- Bitoum M, Levillain O, Tappaz M. Gene expression of the taurine transporter and taurine biosynthetic enzymes in rat kidney after antidiuresis and salt loading. Pflugers Arch Eur J Physiol 2001;442:87-95.
- Lourenco R, Camilo ME. Taurine: a conditionally essential amino acid in humans? An overview in health and disease. Nutr Hosp 2002;17:262-70.
- Aneja R, Hake PW, Burroughs TJ, Denenberg AG, Wong H, Zingarelli B. Epig allocatechin, a green tea polyphenol, attenuates myocardial ischemia reperfusion injury in rats. Mol Med 2004;10:55-62.
- Winterbourn CC, Vissers MC, Kettle AJ. Myeloperoxidase. Curr Opin Hematol 2000;7:53-8.
- Li JX, Pang YZ, Tang CS, Li ZQ. Protective effect of taurine on hypochlorous acid toxicity to nuclear nucleoside triphosphatase in isolated nuclei from rat liver. World J Gastroenterol 2004;10:694-8.
- Chang L, Xu J, Yu F, Zhao J, Tang X, Tang CS. Taurine protected myocardial mitochondria injury induced by hyperhomo-cysteinemia in rats. Amino Acids 2004;27:37-48.