Influence of intralipid on free propofol fraction assayed in human serum albumin solutions and human plasma
Introduction
The main medium distributing drugs in the human organism is blood, in which they are partly bound with proteins and blood cells and partly exist in a free form. It is generally assumed that only an unbound drug can reach the site of action by diffusing across the membranes and exerting pharmacological effects by interacting with receptors[1]. For this reason, the therapeutic effect of a drug should be related not to its total, but to its free concentration. Most frequently, however, the therapeutic effect of a drug is correlated with its total concentration, since for many drugs, their free fraction is constant in the wide range of their total concentrations. Moreover, the procedure of total drug assay is cheaper, quicker and simpler. Free drug measurement is recommended mainly for the potentially dangerous drugs of a narrow therapeutic window with significant toxic effects at higher concentrations and when the protein level is changing[2], for example, in the case of renal or hepatic failure[3,4]. It should also be remembered that changes in the free fraction of a drug are more pronounced for highly-bound drugs, where even a slight decrease in protein binding may substantially increase their free fraction and cause a dangerous toxic effect.
Propofol is an example of a high (97%–99%) protein-binding drug[5–9]. It is one of the most frequently applied intravenous anesthetics used mainly for induction and maintenance of total anesthesia. It is also used in smaller doses for sedative, antiemetic, antipruritic or anticonvulsant purposes[10]. As its effect site is the central nervous system, propofol can be highly dangerous when applied as a sedative agent in long-time infusions to high-risk patients hospitalized in intensive care units.
The decrease of total propofol concentration below 3 μg/mL leads to an increase of its free fraction[11]. At very low total propofol concentrations, its free fraction tends to reach 100%[11]. The cited results were obtained when investigating the total and free drug concentration in human plasma and human serum albumin (HSA) solutions spiked with an ethanolic solution of propofol. It is not known however if the same happens in protein samples (plasma or HSA) spiked with propofol in intralipid emulsion (eg Diprivan, Astra-Zeneca, Caponago, Italy). Some changes can be expected, taking into account the results obtained by Zamacona et al[12], who have shown the increase of propofol binding by blood proteins at elevated concentrations of triglycerides and cholesterol. The resolution of this problem is important for 2 reasons: (1) clinically, because in a typical clinical procedure propofol is administered in the form of soybean oil emulsion; and (2) analytically, to find out if it is essential to spike protein samples with propofol in intralipid solution or if the ethanolic solutions of propofol are sufficient to model the discussed phenomenon.
Materials and methods
Sample preparation and processing
Artificial plasma (HSA solution) A part of the investigations was performed using artificial plasma solutions containing different amounts of HSA. They were obtained by diluting the HSA preparation (ZLB, Bern, Switzerland) with the physiological sodium chloride solution (Polfa, Lublin, Poland) to a required concentration. The following solutions were prepared: 3, 4, 6.5 and 8 g/100 mL.
Human plasma Plasma was obtained from the blood of a healthy volunteer. Blood sampling was performed after obtaining an approval from the University Ethics Committee and consent from the subject. The plasma was isolated from heparinized blood by centrifugation at 85×g.
Propofol solutions Three types of propofol solutions were prepared:
1. Artificial plasma samples containing 4 g of HSA per 100 mL of the solution were spiked with propofol (Aldrich, Steinheim, Germany) solution in 40% ethanol (POCh, Gliwice, Poland) to reach the following total propofol concentrations: 1, 3, 5, 8, and 12 μg/mL. No intralipid was present in these samples. The final concentration of ethanol in the samples was 0.2% (different volumes of propofol solution were mixed with 40% ethanol in order to introduce the constant volume of alcohol into each sample).
2. Artificial plasma samples containing 3 or 4 or 6.5 or 8 g HSA/100 mL were spiked with Diprivan™ preparation (AstraZeneca, Caponago, Italy), that is, 1% propofol solution in intralipid to the following total propofol concentrations: 1, 3, 5, 8, and 12 μg/mL. The final samples contained the intralipid concentration of 10, 30, 50, 80, and 120 μg/mL respectively.
3. Artificial plasma samples containing 5 g of HSA per 100 mL of the solution were spiked with Diprivan. Subsequently, intralipid (100 mg/mL soybean oil/water emulsion; Baxter Healthcare, Thetford, UK) was added so that the final samples contained constant amount of intralipid (120 μg/mL) and 1, 3, 5, 8, or 12 μg/mL of propofol.
Apart from the artificial systems, samples of human plasma spiked with intralipid propofol solution to the total propofol concentrations of 1, 3, 5, 8, and 12 μg/mL were studied. The obtained samples contained the intralipid concentrations of 10, 30, 50, 80, and 120 μg/mL, respectively.
Unbound propofol isolation Unbound propofol was isolated by ultrafiltration on Amicon Micropartition System (MPS) units (Millipore, Bedford, MA, USA), utilising the YM-10 membranes (product no. 40424, Millipore, USA) of a 10 kDa molecular mass cut-off. The ultrafiltration units were centrifuged in a constant rotor angle centrifuge MPW-341 (Mechanika Precyzyjna, Warsaw, Poland). One mL of each solution was put into the sample compartment of the ultrafiltration unit. After the attachment of the ultrafiltrate collection container, the unit was centrifuged at 800×g until 400 mL of ultrafiltrate was obtained.
Propofol assay For propofol assay, to each ultrafiltrate of plasma sample (artificial or native, 400 μL), thymol (internal standard), dihydrogen sodium phosphate (1 mL of 0.1 mol/L NaH2PO4), and cyclohexane (3 mL) were added. The mixtures were vigorously shaken for 10 min at 200 r/min. After centrifugation 1100×g for 5 min, to separate the phases, an aliquot of the cyclohexane layer (2 mL) was transferred to a clean tube with tetramethylammonium hydroxide (TMAH) solution (10 µL). The solvent was evaporated until dry in a stream of nitrogen. The residue was redissolved in the mobile phase and injected into the chromatographic column. The limit of propofol quantitation in plasma ultrafiltrate was 3.7 ng/mL with a coefficient of variation (n=3) of 11.1% at 5 ng/mL, 12.1% at 20 ng/mL and 9.8% at 40 ng/mL. The relative error of determination (n=3) was 32.0% at 5 ng/mL, 9.3% at 20 ng/mL and -6.8% at 40 ng/mL. The linearity range of the whole analytical procedure was from 3 to 650 ng/mL. Other details of sample preparation and the measurement procedure are found in other studies[7–9].
Chromatographic equipment The concentrations of propofol were measured by high performance liquid chromatography (HPLC) in plasma as well as in cerebrospinal fluid (CSF). A Gilson liquid chromatograph (Middleton, WI, USA) consisting of a dual high-pressure pump (Model 122) integrated with a manometric module and a dynamic mixer was employed for HPLC analysis. Propofol in the plasma ultrafiltrate was detected with a fluorescence detector (Jasco FP-920, Tokyo, Japan) set at an excitation wavelength of 276 nm and at an emission wavelength of 310 nm. Chromatographic separations were carried out using a 150×4.6 mm id C18 silica gel column (Kromasil C18, 5 µm, HiChrom, Novato, CA, USA) equipped with a 0.5 µm prefilter (Supelco, Bellefonte, PA, USA) and a guard column ODS C18 (Alltech, Deerfield, IL, USA). The samples were injected into the column by a Model 7125 injection valve from Rheodyne (Cotati, CA, USA).
Reagents and solutions All chemicals, except those separately mentioned, were obtained from the Polish Factory of Chemical Reagents-POCh (Gliwice, Poland) and were of analytical grade. A mixture composed of 75% methanol and 25% deionized Milli-Q water (v/v) was used as the mobile phase. Stock solutions of thymol and propofol in methanol (1 mg/mL) were each prepared and stored at 4 oC. TMAH (25% solution in methanol; Aldrich, Germany) was diluted with isopropanol (3:37 v/v).
Results and Discussion
As earlier outlined, the objective of the present study was to verify if the free propofol fraction increase at a low total drug concentration observed in plasma and HSA solutions spiked with propofol in ethanolic solution[11] occurs when propofol is introduced into protein samples in intralipid emulsion. The run of the relationships between the free propofol fraction and total drug concentration shown in Figure 1 proves that the free propofol fraction increases irrespective of the type of propofol solvent used. Moreover, a significant increase of the free propofol fraction below the total propofol concentration of 3 μg/mL was observed. Thus, the increase of the free propofol fraction resulting from the decrease of total drug concentration occurs both in the presence of ethanol and intralipid.
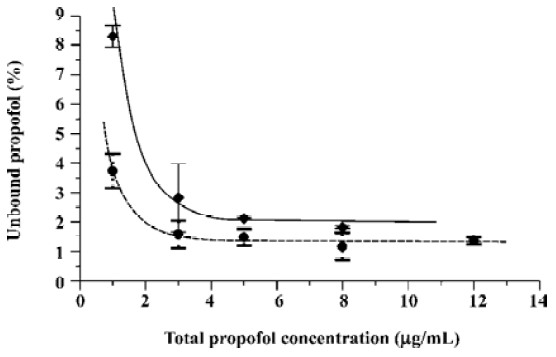
In the present research, the precise range of the free propofol fraction is not as important as the occurrence of its increase at a low total drug concentration. Hence, it was decided to apply the typical procedure of propofol determination by a simpler and more reliable HPLC with fluorescence detection[13] rather than the GC procedure requiring propofol derivatization[11].
As seen in Figure 1, the main difference between the investigated systems is the level of unbound propofol percentage. Spiked HSA with propofol in intralipid solution lowers the free drug fraction in comparison with the samples spiked with ethanolic solution. The observed decrease of the free drug percentage can be attributed to the lipoprotein modification of HSA by soybean lipids resulting in higher affinity of the formed lipoproteins towards propofol.
Other experiments performed with different HSA concentrations in artificial plasma spiked with intralipid solution of propofol (see Figure 2) not only confirm the increase of the free propofol fraction at a low total drug concentration for a wide range of protein concentrations (3–8 g/100 mL), but also allow one to formulate the relationship between the free drug percentage and the total protein concentration (see Figure 3). The observed decrease of the unbound propofol percentage with the increase of protein content in the sample is logical considering the binding properties of HSA towards the drug.
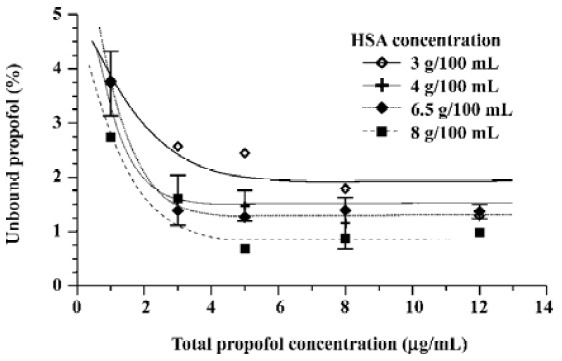
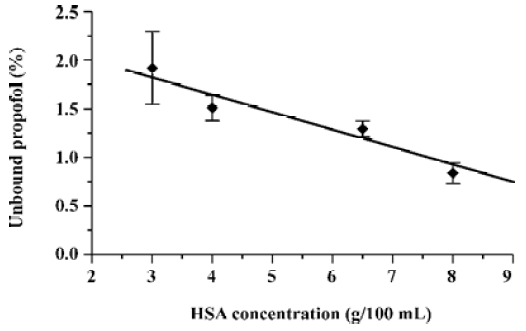
The phenomenon of the free propofol fraction increase at low total drug concentrations in the samples spiked with propofol in the intralipid emulsion is typical, not only for artificial plasma, but also for the samples of natural plasma (see Figure 4). As discussed, the increase of the free propofol fraction is observed below 3 µg/mL of the total propofol concentration.
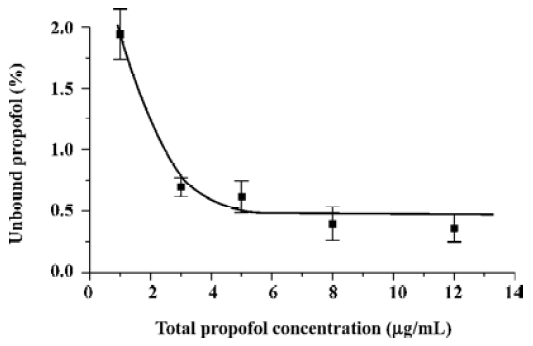
The samples prepared for the experiments illustrated in Figures 1–4 contained not only different amounts of propofol, but also proportionally different amounts of intralipid. Such variations are typical for patients receiving intralipid only in the form of propofol intralipid emulsions, that is, the greater the propofol infusion dose, the greater the amount of infused intralipid. It can be said that the observed increase of free propofol fraction resulting from the decrease of the total drug concentration is connected with the lowering intralipid concentration in the samples. In order to exclude the influence of intralipid concentration changes on the observed phenomenon, it was decided that the experiment be repeating and keeping the intralipid concentration at a constant level of 120 µg/mL, which corresponds to the highest investigated concentration of propofol. The results of this experiment are presented in Figure 5. The data in Figure 5 may be treated as more representative for the samples taken from patients receiving not only propofol infusions, but also drug-free intralipid as a parenteral nutrient. Figure 5 shows that the decrease of the total propofol concentration below 3 µg/mL leads to an increase of its free fraction despite the intralipid concentration. The smaller values of the free drug percentage in the samples containing 120 µg/mL of intralipid can also be attributed to the increased affinity of propofol to the lipid-modified HSA molecule. The whole collected data set (see Figures 1–5) suggests also that the unbound propofol fraction changes tend to be far less pronounced at total drug concentrations higher than 3 µg/mL, that is, at the level which typically exists in the blood of patients when propofol total anesthesia is maintained. Thus, the discussed effect is not likely to occur in total anesthesia conditions, provided that the patients’ hemodynamic parameters remain stable. Changes of hemodynamic parameters during anesthesia can cause free propofol fraction change, as presented by Takizawa et al[14].
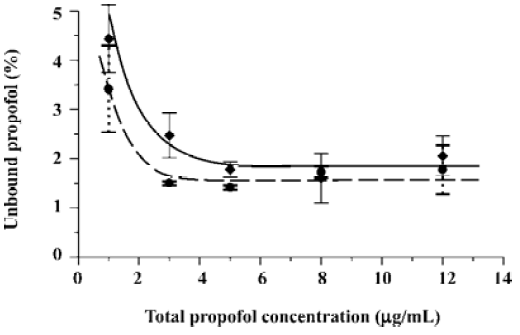
The following conclusions were reached:
1. The presence of intralipid in protein samples does not suppress the rise of the unbound propofol fraction at small total drug concentrations, however, the addition of intralipid noticeably lowers the free propofol percentage. The overall rise of the free propofol fraction at a low total drug concentration, occurring even at the presence of intralipid, must not be ignored when the drug is applied for sedative, antiemetic or other low-dosage purposes.
2. The relationship between the protein concentration and the unbound propofol fraction is almost linear.
3. The obtained results suggest that the protein samples spiked using ethanolic propofol solutions can be treated as representative for human plasma samples taken from the patients receiving propofol infusions in the intralipid form.
4. The increase of the free propofol percentage induced by the decrease of the total drug concentration in the presence of protein-binding intralipid suggests the need for a broader scope of research concerning drug-protein interactions, particularly the influence of 1 drug total concentration on the free fraction of another drug. The present results are by no means sufficient in this respect.
References
- Mehta AC. Therapeutic monitoring of free (unbound) drug levels: analytical aspects. Trends Anal Chem 1989;8:107-12.
- Paxton JW. Drug binding to plasma proteins. N Z Med J 1985;98:245-8.
- Svensson CK, Woodruff MN, Baxter JG, Lalka D. Free drug concentration monitoring in clinical practice, rationale and current status. Clin Pharm 1986;11:450-69.
- Olsen GD, Bennett WM, Porter GA. Morphine and phenytoin binding to plasma proteins in renal and hepatic failure. Clin Pharmacol Ther 1975;17:677-84.
- Gin T. Pharmacodynamics of propofol and free drug concen-trations. Anesthesiology 1993;78:604.
- Vuyk J, Engbers FH, Lemmens HJ, Burm AG, Vletter AA, Gladines MP, et al. Pharmacodynamics of propofol in female patients. Anesthesiology 1992;77:3-9.
- Dawidowicz AL, Kalitynski R. HPLC investigation of free and bound propofol in human plasma and cerebrospinal fluid. Biomed Chromatogr 2003;17:447-52.
- Dawidowicz AL, Kalitynski R, Fijalkowska A. Relationships between total and unbound propofol in plasma and CSF during continuous drug infusion. Clin Neuropharmacol 2004;27:129-32.
- Dawidowicz AL, Kalitynski R. Effects of intraoperative fluid infusions, sample storage time, and sample handling on unbound propofol assay in human blood plasma. J Pharm Biomed Anal 2005;37:1167-71.
- Borgeat A, Wilder-Smith OH, Suter PM. The nonhypnotic therapeutic applications of propofol. Anesthesiology 1994;80:642-56.
- Dawidowicz AL, Kalitynski R, Kobielski M, Pieniadz J. Influence of propofol concentration in human plasma on free fraction of the drug. Chem Biol Interact 2006;159:149-55.
- Zamacona MK, Suarez E, Garcia E, Aguirre C, Calvo R. The significance of lipoproteins in serum binding variations of propofol. Anesth Analg 1998;87:1147-51.
- Plummer GF. Improved method for the determination of propofol in blood by high-performance liquid chromatography with fluorescence detection. J Chromatogr 1987;421:171-6.
- Takizawa E, Hiraoka H, Takizawa D, Goto F. Changes in the effect of propofol in response to altered plasma protein binding during normothermic cardiopulmonary bypass. Br J Anaesth 2006;96:179-85.