Rat adipose-derived stromal cells expressing BMP4 induce ectopic bone formation in vitro and in vivo1
Introduction
The treatment of fracture nonunion and segmental bone defect is a commonly attempted procedure in orthopedic surgery, in which bone augmentation is usually required. Because of the limited supply and associated morbidity of autologous bone grafts and the limited osteoinductive potential of allograft bone, there is increased interest in using tissue engineering and gene therapy strategies to enhance bone repair. Bone morphogenetic proteins (BMP), which belong to the TGF-β superfamily, have been the most frequently studied osteoinductive cytokines. A variety of animal studies with BMP have yielded promising results in promoting fracture repair and bone healing[1], but because of the inability to identify a suitable delivery system, the requirement of large doses, and its half-life, short-term bioavailability greatly limited their application into clinical trials. However, gene therapy could provide an alternative method for the delivery of the BMP protein into tissues for either short term or long-term expression. Some researchers have confirmed the ability of virally (eg adenovirus, retrovirus, adeno-associated virus) mediated gene transfer of BMP (eg BMP2, 4, 6, 7) to induce bone formation in vitro and in vivo[2–6]. BMP4 is one of the structurally related BMP belonging to the TGF-β superfamily. The major practical application of BMP4 may be its capacity of stimulating bone repair[3]. Previous studies have demonstrated that BMP4 could participate in in vivo endochondral ossification and was one of the main local contributing factors in callus formation in the early phase of fracture healing[7]. BMP4 is found in primitive mesenchymal and chondrocytic cells, the cambium layer of the periosteum, the marrow cavity and the muscles adjacent to the fracture site[8]. In contrast, BMP2 is found only in the bone itself. This indicates that BMP4 may play a unique role in the early phases of fracture healing. Furthermore, the BMP4 adenovirus displayed more osteogenic potential compared with the BMP2 and BMP7 adenovirus[4].
Recently, the use of cell therapy based gene delivery of osteogenic factors to facilitate bone healing has attracted particular attention[7,9–11]. To date, several cell populations have been used for such studies, including primary bone marrow stromal cells (BMSC)[7], skeletal muscle-derived cells[12], chondrocytes[13] and skin fibroblasts[10]. Bone marrow has been well established as a source of mesenchymal stem cells capable of differentiation into multiple mesenchymal tissue lineages (eg bone, cartilage, muscle and tendon) when appropriately stimulated. Due to their natural osteoprogenitor characteristics, primary BMSC are likely candidates for the ideal delivery vector in gene therapy for bone healing[6,13-15]. Autologous BMSC are taken from bone marrow through biopsy, which is painful and yields only a limited number of stem cells. Thus, culture expansion of these cells is demanded, especially in the older population[16,17]. Recent studies have indicated that adipose-derived stromal cells (ADSC) are capable of differentiating along multiple mesenchymal cell lineages including osteoblasts, neural cells, chondrocytes and myoblasts[16,18–20] and fat tissue is a reliable source of autologous ADSC. Compared with cells harvested by bone marrow aspiration, ADSC are easily and abundantly available and carry a relatively lower donor site morbidity[17]. Attaining large numbers of stem cells at harvest could reduce the required amount of harvested fat tissue, which is most beneficial in non-obese individuals, and would reduce or even eliminate the need for costly and lengthy tissue culture expansion that would subject the patient to a staged second procedure[17]. The ideal bone engineering technique should involve procurement of clinically useful numbers of progenitor cells at initial harvest, the addition of an osteogenic signaling protein or gene, and implantation into the patient during the same procedure. Hence, these cells are a potential substrate for the clinical application of bone tissue engineering. However, whether ADSC could be effectively used in an ex vivo system (requiring harvesting, manipulation, and re-implantation) for osteoinductive regional gene therapy requires further investigation.
In the present study, we used an adenovirus vector encoding BMP4 to transduce ADSC to observe whether the overexpression of BMP4 can enhance the osteogenic potential of ADSC in terms of osteogenic differentiation and mineralization in vitro and induce new bone formation in vivo.
Materials and methods
Isolation and culture of rat ADSC All animal experimental protocols were approved by the Animal Care and Use Committee of Peking University, Beijing, China and were in compliance with the “Guide for the Care and Use of Laboratory Animals” published by the National Academy Press (NIH Publication N
Construction of adenovirus BMP4 vector The first generation (E1–, E3–) recombinant adenoviral vector was used to construct rat BMP4 recombinant using the AdEasy-1 system (gift from Dr T HE, Howard Hughes Medical Institute, Baltimore, MD)[21]. Briefly, rat BMP4 (gift from Dr Yan CHAN, Hospital for Sick Children, University of Toronto, Ontario, Canada) was inserted into the Xho I and EcoR V restriction sites of the shuttle vector pAdTrack-CMV(cytomegalovirus). The resultant DNA was linearized by digestion with Pme I, and subsequently cotransformed into E coli BJ5183 with an adenoviral backbone plasmid (pAdEasy-1). Recombinants were selected for kanamycin resistance and confirmed by digestion with Pac I. Finally, the linearized recombinant plasmid was transfected into 293A cells using the lipofectamine (Invitrogen, Carlsbad, CA, USA) method for adenovirus packaging. The cells were harvested until full cytopathic effect was evident, and then underwent 3 cycles of freezing and thawing. The cell lysates were then collected after centrifugation. The primary recombinant adenovirus with rat BMP4 gene (named Ad-BMP4) was propagated by re-infecting 293A cells and purified by CsCl gradient ultracen-trifugation. Purified viruses were stored in 10% glycerol/phosphate buffered saline (PBS) at -80 °C at a concentration of 2×1010plaque-forming units (pfu)/mL. The control recombinant adenovirus carrying the enhanced green fluorescence protein gene, Ad-EGFP (enhanced green fluorescence protein), was constructed with a similarl method (1×1010 pfu/mL).
In vitro transduction of Ad-BMP4 into ADSC To demonstrate the transduction efficiency by adenovirus, ADSC were seeded at 3×105 onto a 6-well plate in growth medium and allowed to reach 80% confluence. The cells were transduced with Ad-BMP4 at an multiplicity of infection (MOI; pfu/cell) of 200, 300 and 0 (mock) for 24 h. The cells were then observed under fluorescence microscope.
RT-PCR analysis Total RNA were prepared from cultured cells (1×106) with Trizol reagent following a standard protocol provided by the manufacturer (Life Technologies, Gaithersburg, MD, USA). Isolated RNA was reverse transcribed and then amplified with a commercial kit (Access RT-PCR system, Promega, Madison, WI, USA) according to the manufacturer’s protocol. Oligonucleotide primers (Table 1) were designed using Oligo 6 primer analysis software. Total RNA (2 µg) were reverse transcribed for 45 min at 54 °C, and PCR amplification was then performed. PCR products were separated on 1.5% agarose gels and visualized by ethidium bromide staining.
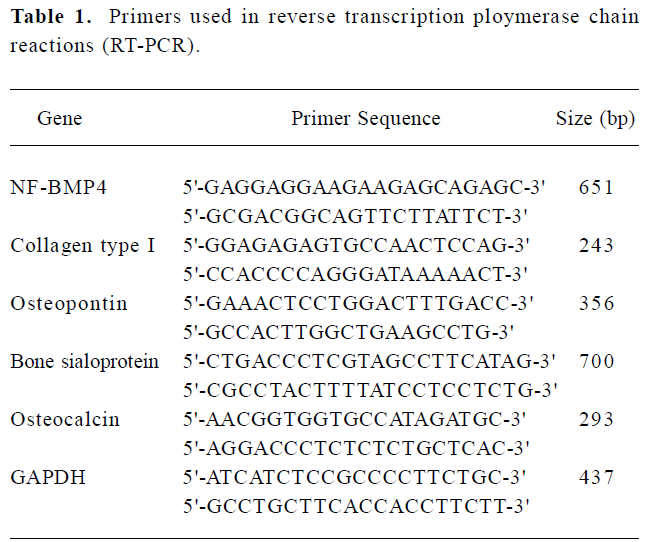
Full table
Immunofluorescence analysis for BMP4 expression in ADSC ADSC were seeded at 1×105 onto a 6-well plate and cultured for 24 h in growth medium. The cells were then transduced with Ad-BMP4 (MOI 200) or Ad-EGFP for 24 h. Then, the ADSC were washed with PBS, fixed with 4% formaldehyde in PBS for 10 min, permeabilized with 0.1% Triton X-100 in PBS for 10 min, treated with normal rabbit serum (Zhongshan Biochemical, Beijing, China) to block non-specific antibody binding, and then incubated with a goat polyclonal antibody against rat BMP4 (Santa Cruz Bio-technology, Santa Cruz, CA, USA) overnight at 4 °C. Cells were then thoroughly washed with PBS and stained with TRITC-conjugated rabbit anti-goat IgG (Zhongshan Bio-chemical, Beijing, China) at a dilution of 1:200 and subsequently analyzed under fluorescence microscope.
Western blotting analysis for BMP4 expression in ADSC ADSC were treated as earlier described and the harvested medium was collected at 48 h post transduction. Proteins were run on SDS-PAGE gels and electrotransferred to nitrocellulose membrane at 4 °C for 2 h. The blots were probed with anti-BMP4 (Santa Cruz, USA) at 1:500 dilutions overnight at 4 °C. The proteins were detected by chemiluminescence according to manufacture’s recommendations (ECL, Amersham Arlington Heights, IL, USA). GAPDH was used as an internal control.
Alkaline phosphatase activity assay ADSC were seeded on 6-well plates and were transduced with Ad-BMP4 (MOI 200) or Ad-EGFP or 0 (mock). Each group consisted of 3 wells. Alkaline phosphatase (ALP) activity assay was carried out as previously described[22]. On d 1, 3, 5, and 7, cell lysates were harvested and ALP activity was measured using an ALP assay kit (Zhongsheng Biochemical, China). The enzyme activity was normalized against the protein concentration and expressed as U·g-1·L-1. Protein concentration was measured by BAC protein assay kit (Pierce Biotechnology Inc., Rockford, IL, USA) using bovine serum albumin as the standard.
Matrix mineralization In order to determinate bone nodule formation and extracellular matrix mineralization, on d 14 the cells were fixed for von Kossa silver staining. Briefly, fixed cells were stained with 5% silver nitrate for 20 min under sunlight, fixed with sodium thiosulfate for 5 min, rinsed with distilled water and mounted for inspection.
Osteogenic activity in athymic nude mice Nine male athymic nude mice (age between 4 and 6 weeks) were used in this study. Athymic nude mice were randomly assigned to 2 groups. Group I contained 6 animals, each of them injected with 2×106 Ad-BMP4-transduced cells and group II contained 3 animals injected with 2×106 Ad-EGFP-transduced cells. The mice were anesthetized with a mixture of ketamine (90 mg/kg, ip) and xylazine (10 mg/kg, ip) and a 5 mm incision was made on the left hind limb under sterile conditions. Then Ad-BMP4-transduced cells or Ad-EGFP-transduced cells were injected directly into the gastrocnemius muscle. The incision was routinely closed with an interrupted 4-0 silk suture.
At 1, 3, and 4 weeks post-operation, all animals were examined by X-ray film for evidence of new bone formation and then were killed by an administration of a fatally high dose of anesthetics. The newly formed bone at the operation site was evaluated by radiographic and histological analyses. The harvested ossified tissues were fixed in 10% formalin neutral buffer solution at pH 7.4 for 2 d and then decalcified with decalcifying solution composed of 10% HCl and 0.1% ethylenediaminetetraacetic acid for another 2 d. The specimens were then dehydrated through a series of graded ethanol, followed by infiltration and embedding into paraffin wax. The tissues were cut into 6 µm sections and stained with hematoxylin eosin (HE) and alcian blue.
Results
BMP4 expression in ADSC transduced with Ad-BMP4 RT-PCR clearly demonstrated the expression of BMP4 in Ad-BMP4-transduced cells. A 651 bp fragment was seen in Ad-BMP4-transduced ADSC cells at 24 h, but not in Ad-EGFP-transduced and untransduced cells (Figure 1A). The expres-sion of BMP4 in the supernatant of ADSC transduced with Ad-BMP4 was confirmed by Western blotting analysis. The expression of BMP4 protein was visualized only in the ADSC transduced with Ad-BMP4 (Figure 1B). No definitive immunoblotting band was found in the conditioned medium of untransduced or Ad-EGFP-transduced cells.
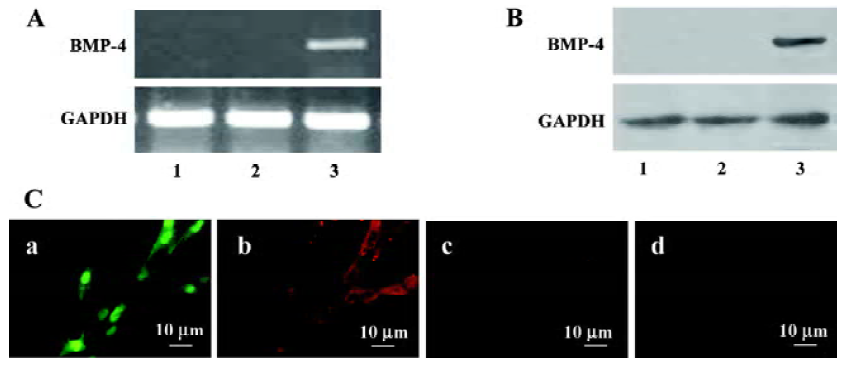
Immunofluorescence analysis revealed the expressed BMP4 protein accumulated mainly in the cytoplasm in Ad-BMP4-transduced cells. In the negative control without primary antibody and Ad-EGFP-transduced cells, no BMP4 positive cells were detected and only faint background staining was observed (Figure 1C).
Osteogenic induction activity in Ad-BMP4-transduced ADSC in vitro The expression of osteogenic genes including collagen type I, bone sialoprotein, osteopontin and osteocalcin was assessed at 3 and 7 d by RT-PCR. Upregulated mRNA expression of collagen type I, bone sialoprotein, osteopontin was observed in ADSC transduced with Ad-BMP4 (Figure 2A) at 3 and 7 d, and osteocalcin was found at 7 d. In ALP activity assay, although there was an amount of cellular intrinsic ALP activity, ADSC transduced with Ad-BMP4 showed an increase of ALP activity compared to those transduced with Ad-EGFP or untransduced cells (Figure 2B). Von Kossa staining for phosphate deposits was used to detect matrix mineralization of ADSC at 14 d post-transduction. Ad-EGFP-transduced cells and untransduced cells exhibited no mineral deposition, while Ad-BMP4-transduced cells displayed strong positive staining for mineralization (Figure 2C).
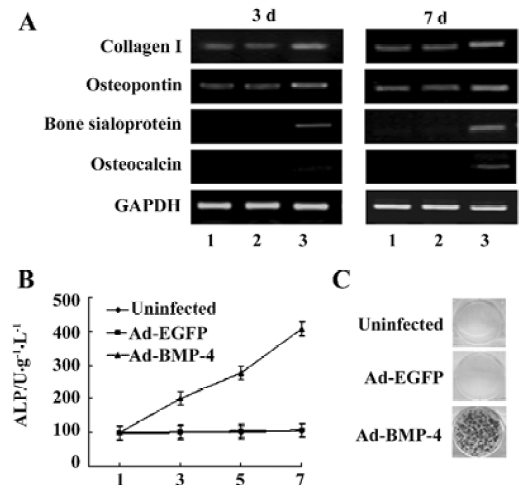
Radiographic evidence for bone formation in athymic mice Under X-ray examination, new bone was visible in all athymic nude mice injected with Ad-BMP4 transduced cells (Figure 3) as early as 3 weeks post-operation. In contrast, no radiographic evidence for bone formation could be seen in the mice implanted with Ad-EGFP-transduced cells at all time intervals.
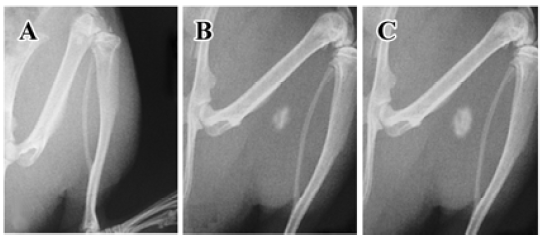
Histological evidence for endochondral ossification Athymic nude mice were sacrificed at 1, 3, and 4 weeks after the operation and had undergone histological analysis. HE and alcian blue staining revealed remodeled bone tissue only in mice receiving Ad-BMP4 (Figure 4). One week after the operation, there was a significant accumulation of chondrocytes within the injected muscle tissues, which produced a cartilaginous matrix surrounded by some cells suggestive of undifferentiated mesenchymal cells. At 3 weeks’ post-injection, woven bone was formed in the sections, with a well-defined cortical rim and trabecular structure. The irregular medullary cavity containing bone marrow-like cells and adipocytes was also visible. Four weeks later, the woven bone became more mature, characterized by a well-defined and highly-organized lamellar cortical bone matrix and an enlarged medullary cavity. These results demonstrated that ADSC transduced with Ad-BMP4 had induced bone formation in the muscle tissues via endochondral mechanism. In contrast, no bone tissue structure was found in the mice receiving Ad-EGFP injection (Figure 4). There were no detectable immunological and inflammatory responses in any mice, and all mice survived until the scheduled date of killing with no apparent complications.
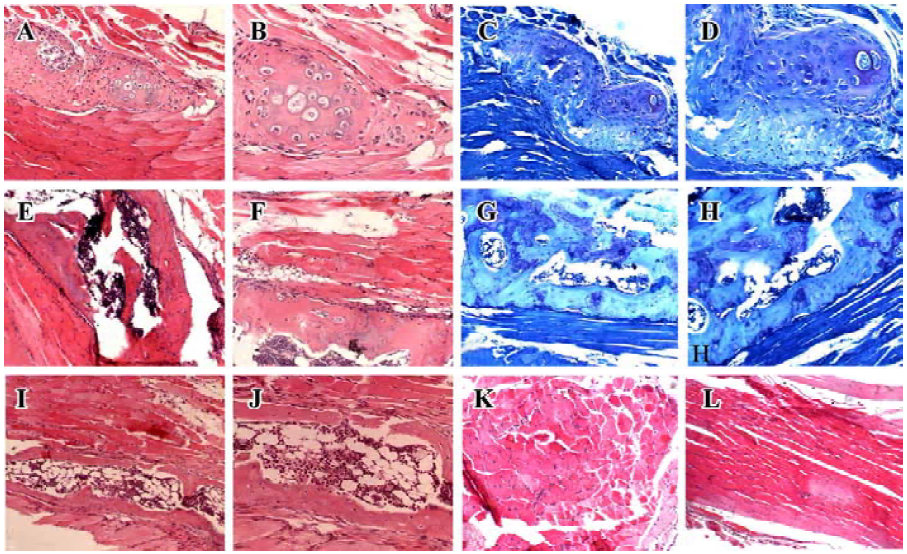
Discussion
This study demonstrates that ADSC can undergo efficient and stable transduction by an adenovirus vector encoding BMP4, and more importantly, they can induce new bone formation in athymic mice.
The ideal source of adult autologous cells suitable for clinical therapy should be readily harvested, rapidly expandable in sufficient number, and have a strong potential for differentiation. At the same time, for clinical application of cell-based gene therapy, the target cells need to be efficiently transduced with a vector and able to express the desired protein. ADSC could be amplified to sufficient numbers by short-term culture for implantation in vivo and are capable of differentiating into osteoblasts, adipocytes, muscle cells and neural cells when treated with established lineage-specific factors[18–21]. In our study, about 50% of ADSC transduced at the MOI 200 were positive and the transduction efficiency increased with the increased MOI with an over 90% transduction rate at the MOI 300 (data not shown) without selection, thus indicating a high initial transduction efficiency at a MOI of 300.
Adenoviral vectors can mediate gene transfer to both replicating and non-replicating cells and be produced in high titres. One of the disadvantages of adenoviral vectors is that these vectors could elicit immune responses which could produce adverse effects in immunocompetent animal and would not allow for sustained expression of the therapeutic gene[23]. Previous studies revealed only transient gene expression due to the adenovirus’ extrachromosomal life cycle[24], which is advantageous for growth factor-based gene therapies for bone defects because long-term generation of secreted therapeutic protein is not necessary for many patients with bone defects[6,24]. In addition, the lack of integration also obviates the danger of insertional mutagenesis associated with other vectors such as retrovirus[12,24]. Therefore, we chose the adenoviruses delivery system containing BMP4 to investigate the osteogenic differentiation of ADSC in vitro and in athymic mice. After in vitro transduction, the ADSC could express the BMP4 protein in the cytoplasm and secrete it into the culture medium in our study, as was proved by immunofluorescence and Western blotting analysis.
The expression of osteoblast specific genes including collagen type I, bone sialoprotein, osteopontin and osteocalcin was strongly upregulated in ADSC transduced with Ad-BMP4. Type I collagen, one of the first products formed during bone induction, is the foundation of the extracellular matrix on which future mineralization takes place. The level of type I collagen was upregulated in ADSC expressing BMP4 at 3 d, indicating the beginning of extracellular matrix deposition. Osteopontin is another non-specific early bone marker that was found at both 3 and 7 d. Bone sialoprotein is a late bone marker only produced by mineralizing cell types which was found in ADSC transduced with Ad-BMP4 at 3 and 7 d. The presence of bone sialoprotein in 3 and 7 d cultures indicates rapid osteoinduction. Osteocalcin is another late bone marker and signals terminal osteoblast differentiation. Osteocalcin was produced by ADSC transduced with Ad-BMP4 in 7 d cultures. Furthermore, Ad-BMP4 transduced cells demonstrated a significant increase of ALP activity and matrix mineralization which indicated BMP4 might induce functional osteogenesis. The high levels of ALP expression of ADSC transduced with Ad-BMP4 also demonstrated that the transduced cells were responding to the bioactive BMP4 they secreted in an autocrine manner. The ability to respond to autostimulation may allow the ADSC to play both an osteoinductive role, by secreting BMP4 in vivo, and an osteoprogenitor role, as the carrier cells undergo osteogenic differentiation and become bone. It was found that osteogenic potentials of ADSC varied by their anatomical sites with visceral ADSC, exhibiting higher osteogenic potential than those isolated from the subcutis in rabbits. However, the mechanism is still unidentified[20]. Whether the ADSC of different anatomical sites of rats have different osteogenic potentials needs to be investigated.
Previous investigators have demonstrated the potential of BMP4 gene therapy for bone regeneration. Fang and colleagues[24] were the first to document the osteoinductivity of plasmid mediated BMP4 gene transfer for segmental bone defect healing in rats. By means of directly injection, Ad-BMP4 could also be transferred in vivo and induce new bones in mice[3]. However, its clinical application is severely limited by the lack of an appropriate delivery system to achieve a sustained and localized effect in vivo. Recently, a retroviral vector carrying BMP4 cDNA was also developed to produce secretion of high levels of BMP4 protein. Rat muscle-derived stem cells transduced with this retroviral vector could induce in vivo ectopic bone formation and promote critical-sized skull defect healing in mice[12]. Adipose tissue is plentiful, easily obtainable and contains pluripotent cells that can be harvested in large numbers, eliminating the need for culture expansion. Our results demonstrate that a genetically engineered cell-based protein delivery system using ADSC can generate BMP4 protein in vitro and that this sustained BMP4 delivery platform allowed ectopic bone formation in vivo while ADSC transduced with Ad-EGFP could not induce bone formation. Lee et al[25] used Y-chromosome-specific FISH (fluorescence in situ hybridization) to follow the fate of transplanted cells in vivo, and demonstrated that only 5% of genetically engineered original muscle-derived cells differentiated into osteogenic cells, while a large number of implanted cells enhanced bone healing primarily by delivering BMP2. Whether ADSC underwent the same fate in our study remains unknown. We have no direct evidence to demonstrate whether the implanted cells underwent bone formation or just exerted their effect as a vehicle delivering BMP4, inducing new bone formation in primitive pluripotent mesenchymal cells attracted to the sites of transplantation. Further investigation is needed to determine the early cellular events after transplantation.
In summary, we have shown that BMP4 induced osteoblastic differentiation and mineralization in adipose tissue-derived stromal cells in vitro and in vivo. ADSC appear to be an ideal source of mesenchyme-lineage stem cells for gene therapy and engineering strategies for tissue regeneration. If ADSC transduced with Ad-BMP4 can heal bony defects in vivo, these cells could be an attractive alternative to BMSC, which are more difficult to obtain in adequate numbers. Although there are many issues that need to be addressed before ADSC Ad-BMP4 can be further applied in humans, our result is still promising for bone augmentation in future clinical settings, including the treatment of fracture nonunion, segmental bone defect, spinal fusion, or other orthopedic disorders.
Acknowledgement
We thank Dr Yan CHEN (Hospital for Sick Children, University of Toronto, Ontario, Canada) for kindly providing us BMP4 cloning plasmid containing full-length rat BMP4 cDNA, Dr TC HE (Howard Hughes Medical Institute, Baltimore, MD) for providing the AdEasy System.
References
- Franceschi RT, Yang S, Rutherford RB, Krebsbach PH, Zhao M, Wang D. Gene therapy approaches for bone regeneration. Cells Tissues Organs 2004;176:95-108.
- Engstrand T, Daluiski A, Bahamonde ME, Melhus H, Lyons KM. Transient production of bone morphogenetic protein 2 by allogeneic transplanted transduced cells induces bone formation. Hum Gene Ther 2000;11:205-11.
- Chen Y, Cheung KMC, Kung HF, Leong JCY, Lu WW, Luk KDK. In vivo new bone formation by direct transfer of adenoviral mediated bone morphogenetic protein-4 gene. Biochem Biophys Res Commun 2002;298:121-7.
- Yang M, Ma QJ, Dang GT, Ma KT, Cheng P, Zhou CY. Adeno-associated virus-mediated bone morphogenetic protein-7 gene transfer induces C2C12 cell differentiation into osteoblast lineage cells. Acta Pharmacol Sin 2005;26:963-8.
- Li JZ, Li H, Sasaki T, Holman D, Beres B, Dumont RJ, et al. Osteogenic potential of five different recombinant human bone morphogenetic protein adenoviral vectors in the rat. Gene Ther 2003;10:1735-43.
- Lieberman JR, Daluiski A, Stevenson S, Jolia L, Wu L, McAllister P, et al. The effect of regional gene therapy with bone morphogenetic protein-2-producing bone marrow cells on the repair of segmental femoral defects in rats. J Bone Joint Surg 1999;81A:905-17.
- Nakase T, Nomura S, Yoshikawa H, Hashimoto J, Hirota S, Kitamura Y, et al. Transient and localized expression of bone morphogenetic protein 4 messenger RNA during fracture healing. J Bone Miner Res 1994;9:651-9.
- Yaoita H, Orimo H, Shirai Y, Shimada T. Expression of bone morphogenetic proteins and rat distal-less homolog genes following rat femoral fracture. J Bone Miner Metab 2000;18:63-70.
- Bosch P, Musgrave DS, Lee JY, Cummins J, Ghivizzani S, Evans C, et al. Osteoprogenitor cells within skeletal muscle. J Orthop Res 2000;18:933-44.
- Krebsbach PH, Gu K, Franceschi RT, Rutherford RB. Gene therapy directed osteogenesis: BMP-7-transduced human fibroblasts form bone in vivo. Hum Gene Ther 2000;11:1201-10.
- Starr AJ. Recombinant human bone morphogenetic protein-2 for treatment of open tibial fractures. J Bone Joint Surg 2003;85A:2049-50.
- Wright V, Peng H, Usas A, Gearhart B, Cummins J, Huard J. BMP4-expressing muscle-derived stem cells differentiate into osteogenic lineage and improve bone healing in immunocompetent animals. Mol Ther 2002;6:169-78.
- Musgrave DS, Bosch P, Lee J, Pelinkovic D, Ghivizzani S, Whalen J, et al. Ex vivo gene therapy to produce bone using different cell types. Clin Orthop 2000.290-305.
- Werntz JR, Lane JM, Burstein AH, Justin R, Klein R, Tomin E. Qualitative and quantitative analysis of orthotopic bone regeneration by marrow. J Orthop Res 1996;14:85-93.
- Zuk PA, Zhu M, Ashjian P, De Ugarte DA, Huang JI, Mizuno H, et al. Human adipose tissue is a source of multipotent stem cells. Mol Biol Cell 2002;13:4279-95.
- Dragoo JL, Choi JY, Lieberman JR, Huang J, Zuk PA, Zhang J, et al. Bone induction by BMP-2 transduced stem cells derived from human fat. J Orthop Res 2003;21:622-9.
- Kang SK, Lee DH, Bae YC, Kim HK, Baik SY, Jung JS. Improvement of neurological deficits by intracerebral transplantation of human adipose tissue-derived stromal cells after cerebral ischemia in rats. Exp Neurol 2003;183:355-66.
- Estes BT, Wu AW, Guilak F. Potent induction of chondrocytic differentiation of human adipose-derived adult stem cells by bone morphogenetic protein 6. Arthritis Rheum 2006;54:1222-32.
- Peptan IA, Hong L, Mao JJ. Comparison of osteogenic potentials of visceral and subcutaneous adipose-derived cells of rabbits. Plast Reconstr Surg 2006;117:1462-70.
- Lee JA, Parrett BM, Conejero JA, Laser J, Chen J, Kogon AJ, et al. Biological alchemy: engineering bone and fat from fat-derived stem cells. Ann Plast Surg 2003;50:610-7.
- Yang M, Ma QJ, Dang GT, Ma K, Chen P, Zhou CY. In vitro and in vivo induction of bone formation based on ex vivo gene therapy using rat adipose-derived adult stem cells expressing BMP-7. Cytotherapy 2005;7:273-81.
- Song C, Guo Z, Ma Q, Guo Z, Ma Q. Simvastatin induces osteoblastic differentiation and inhibits adipocytic differentiation in mouse bone marrow stromal cells. Biochem Biophys Res Commun 2003;308:458-62.
- Gugala Z, Olmsted-Davis EA, Gannon FH, Lindsey RW, Davis AR. Osteoinduction by ex vivo adenovirus-mediated BMP2 delivery is independent of cell type. Gene Ther 2003;10:1289-96.
- Fang J, Zhu YY, Smiley E, Bonadio J, Rouleau JP. Stimulation of new bone formation by direct transfer of osteogenic plasmid genes. Proc Natl Acad Sci USA 1996;93:5753-8.
- Lee JY, Musgrave D, Pelinkovic D, Fukushima K, Cummins J, Usas A, et al. Effect of bone morphogenetic protein-2-expressing muscle-derived cells on healing of critical-sized bone defects in mice. J Bone Joint Surg 2001;83A:1032-9.