Hepatocyte-like cells from directed differentiation of mouse bone marrow cells in vitro1
Introduction
Liver transplantation is one of the most effective treatments for patients with acute and chronic liver failure[1]. However, since donor livers are scare, only a small number of patients can receive a replacement liver in time. Thus, it is necessary to seek alternative therapies to replace liver transplantation.
Bioartificial liver or hepatocyte transplantation, serving as temporary liver support, can provide necessary liver functions[2-3]. The functional hepatocyte is the core of the temporary liver support. Since donor hepatocytes are limited, it is imperative to explore ways to gain functional hepatocytes. Stem cells are defined as cells that have clonogenic and self-renewing capabilities, and can differentiate into multiple different cell types that make up the organ under the right condition or given the right signals[4]. Thus, stem cell can be an ideal resource of functional hepatocytes.
Hepatocytes originating from bone marrow stem cells were first observed in rats after combined bone marrow transplantation and liver damage. In rats, a combination of hepatotoxin, which induces widespread liver damage, and 2-acetylaminofluorine, which prevents endogenous liver repair, were used. Then, a combination of Y chromosome fluorescence in situ hybridization (FISH) and transgene expression were used to confirm that bone marrow stem cells were the source of resultant hepatocytes[5]. The same results were demonstrated in mice[6] and humans[7,8]. In a later study, Lagasse et al demonstrated that transplantation of xc-kithighThy11ow Lin-Sca-1+(KTLS) bone marrow cells to irradiated hosts could treat an inborn error of hepatic meta-bolism[9]. Multipotent adult progenitor cells (MAPCs), a subpopulation of mesenchymal stem cells in the bone marrow, were found to differentiate into many kinds of cells including hepatocytes[10]. Recently, it has been reported that bone marrow stromal cells can develop into hepatocyte-like cells[11]. Thus, we can confirm from these findings that stem cells in bone marrow exist and can differentiate into hepatocytes in vivo and in vitro.
The differentiation processes of stem cells are likely to be complex, but must exist in the microenvironment of the cells, the signals which originate from the extracellular matrix through adhesion-related events[12], and the cocktail of soluble ligands now known to control cell growth, differen-tiation, and morphogenesis[13]. In the course of hepatic differentiation, some cytokines, including fibroblast growth factor-4 (FGF-4)[14], oncostatin M (OSM)[15], hepatocyte growth factor (HGF)[16] and epithermal growth factor (EGF)[17], control the hepatic differentiation and maturation. In the present study, we used the cell directed differentiation medium including FGF-4 and OSM, to investigate hepatocyte-like cells from the directed differentiation of mouse bone marrow cells in vitro.
Materials and methods
Materials C57BL/6 mice (3–4 weeks old) were purchased from the Model Animal Research Center of Nanjing University (Grade: SPF, N
Dulbecco’s modified essential media-low glucose (DMEM-LG), Iscove’s modified Dulbecco’s medium (IMDM), L-glutamine, and fetal calf serum (FCS) were obtained from Hyclone Laboratories (South Logan, UT84321, USA). MCDB-201, insulin-transferrin-selenium (ITS), dexametha-sone, ascorbic acid 2-phosphate, benzylpenicillin, strepto-mycin, and fibronectin were obtained from Sigma-Aldrich (Saint Louis, Missouri 63103, USA). FGF-4 and OSM were obtained from R&D Systems (Minneapolis, MN 55413, USA).
The antibody against mouse albumin was obtained from Dako Systems (DK-2600, Glostrup, Denmark). The antibody against mouse cytokeratin18 was obtained from Chemicon (Temecula, Ca 92590, USA). Fluorescein (FITC)-conjugated secondary antibody, phycoerythrin (PE)-conjugated secondary antibody, and rhodamine (TRITC)-conjugated secondary antibody were obtained from Sigma-Aldrich (Saint Louis, Missouri 63103, USA). Trizol reagent was also purchased from Sigma-Aldrich. A TITANIUMTM one-step RT-PCR kit was purchased from Clontech (Palo Alto, CA 94303-4230, USA). Periodic acid-Shiff (PAS) staining solution was purchased from Shanghai Bioengineer Company (590 Zhaojiabang Road, Shanghai, China). A Colorimetric assay kit was purchased from Randox Laboratories (Randox Laboratories, Antrim, UK).
Preparation of bone marrow cells Bone marrow cells were prepared as previously described[18]. Fresh bone marrow aspirate extracted from the tibias and the femora of the C57BL/6 mice was suspended in DMEM-LG media and was centrifuged to pellet the cells, and the fat was removed. The cell pellet was resuspended in DMEM-LG media and fractionated on a density gradient generated by centrifugation of 1.077 g/L percoll solution at 1150×g for 30 min at the room temperature. The cells in the percoll interface were then collected and rinsed twice. Cell viability was determined by the trypan blue exclusion test. Only suspensions with cell viability of 95% were used.
Directed differentiation of bone marrow cells Bone marrow cells were inoculated in bone marrow cells’ directed differentiation media at 5×105 cells/cm2 in 10 mg/L fibronectin-coated culture flasks. Bone marrow cells’ directed differentiation media consisted of the following: 54% DMEM-LG, 36% MCDB-201, 10% FCS with 1×ITS, 1×10-8 mol/L dexa-methasone, 1×10-4 mol/L ascorbic acid 2-phosphate, 100 U/L benzylpenicillin, 100 mg/L streptomycin, 30 μg/L FGF-4, 30 μg/L OSM. As a negative control, bone marrow cells’ culture medium was similar to the directed differentiation medium but without FGF-4 and OSM. Cells were cultured in a humidified atmosphere of 5% CO2 and 95% air at 37 °C. After 72 h, non-adherent cells and debris were removed, and the adherent cells were cultured continuously. Cultures were maintained by media exchange every 3 d.
Cell morphology was observed under Olympus phase contrast microscope (CX40RF200, Olympus optical Co LTD, Japan). On d 0, 3, 6, 9, 12, 15, 18, 21, some cells were detached with 0.25% trypsin-EDTA solution and collected for RNA extraction. Cells on d 21 were detached with the same method and used for experiments. Cell supernatant on d 0, 3, 6, 9, 12, 15, 18, 21 were collected for albumin ELISA.
Hepatocyte isolation and culture Hepatocytes were isolated from 3–4 weeks old C57BL/6 mice by a conventional two-step collagenase liver perfusion[19] and cultured on fibronectin-coated flasks 10 mg/L in IMDM containing 10% FCS, L-glutamine 2 mmol/L, dexamethasone 1 μmol/L, insulin 1 μmol/L, benzyl penicillin 100 U/L, and streptomycin 100 mg/L. Cultures were maintained by media exchange every 3 d.
RNA extraction and RT-PCR analysis Total RNA was extracted by using Trizol reagent from C57BL/6 mouse hepatocytes, fresh bone marrow cells, cultural bone marrow cells, and directed differentiated bone marrow cells. In total, 1 μg RNA was used for cDNA synthesis and amplification by one-step RT-PCR kit. For hepatocyte nuclear factor-3β (HNF-3β), albumin (ALB), cytokeratin18 (CK18) and transthyretin (TTR), the following reaction conditions were used: reverse transcription at 50 °C for 1 h, denaturation of RNA/DNA hybrid and inactivation of reverse transcriptase at 94 °C for 5 min. Polymerase chain reaction (PCR) was used for 40 cycles, denaturation at 94 °C for 30 s, annealing at 60 °C for 1 min, extension at 72 °C for 1 min, final extension at 72 °C for 5 min. For glucose-6-phosphatase (G-6-Pase) and tyrosine aminotransferase (TAT), the following reaction conditions were used: reverse transcription at 50 °C for 1 h, denaturation of RNA/DNA hybrid and inactivation of reverse transcriptase at 94 °C for 5 min. PCR was used for 30 cycles, denaturation at 94 °C for 1 min, annealing at 55 °C for 1 min, extension at 72 °C for 1 min, final extension at 72 °C for 5 min.
Primers used for amplification are listed in Table 1. All primers were synthesized by Shanghai Sangon Biological Engineering Technology & Service Co Ltd, (Shanghai, China). mRNA levels were normalized using β-actin as a housekeeping gene. The amplified products were subjected to electrophoresis in 1% agarose gels and stained with ethidium bromide.
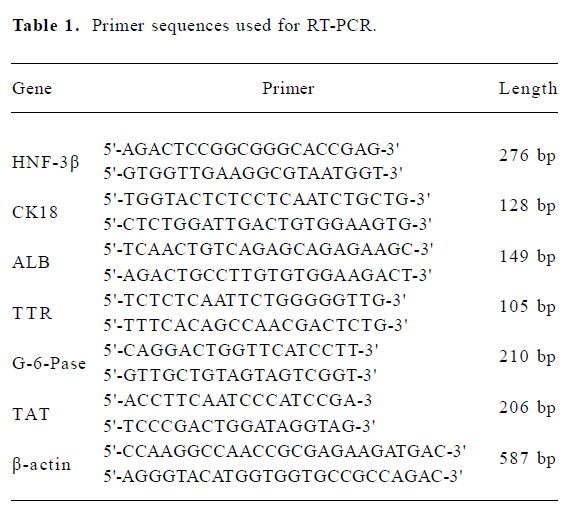
Full table
Western blot analysis Cells, including C57BL/6 mouse hepatocytes, fresh bone marrow cells, cultural bone marrow cells, and directed differentiated bone marrow cells, were washed with 0.01 mol/L PBS and lysed with lysis buffer. About 50 ug of proteins in each supernatant were boiled for 5 min in a sodium dodecyl sulfate (SDS) sample buffer and subjected to electrophoresis on 10% SDS-PAGE gels. The proteins were transferred to Polyvinylidene Fluoride (PVDF) membrane through semidry transfer at 210 mA for 0.5 h. The membrane was blocked for 30 min at room temperature in 3% nonfat milk in Tris-buffered saline (TBS) with 0.05% Tween20, and incubated with primary antibodies against mouse ALB (1:1000), mouse HNF-3β (1:500), and mouse CK18 (1:500) at room temperature for 1 h. The membrane was rinsed and washed 3 times in TBS-Tween20 for 10 min each, and then incubated at room temperature for 1 h with a Horseradish peroxidase (HRP)-conjugated second antibody (1:1000). After adequate washes with TBS, the membrane was stained with diaminiobenzidene (DAB) stain at room temperature for 2–3 min, and then washed with PBS.
Albumin ELISA Cell culture media at various time points were collected as samples for ALB ELISA. One microlitre of a monoclonal antibody against mouse ALB was diluted with 100 μL coating buffer for each well and incubated for 60 min. After incubation, the capture antibody solution was aspirated and washed with wash solution. Total 200 μL of blocking solutions were added to each well and incubated for 30 min. After incubation, the blocking solution was removed and each well was washed. One hundred microlitre standards or samples were transferred to assigned wells and incubated for 60 min. After incubation, standards or samples were removed, and each well was washed. 100 uL HRP conjugate (1:10000) were transferred to each well and incubated for 60 min. After incubation, the HRP conjugate were removed and each well was washed. Tetramethylbenzidine (TMB) 100 μL were transferred to each well and incubated for 30 min. To stop the TMB reaction, 100 μL of H2SO4 2 mmol/L were applied to each well. The plate was read at the wavelength 450 nm for TMB through the microtitration plate reader (TECAN A-S002, Austria).
Periodic acid-Shiff (PAS) staining Cells were fixed with 20% formaldehyde and intercellular glycogen was stained with PAS staining solution according to the standard protocol.
Urea assay Urea concentrations were measured through a colorimetric assay kit. The mouse bone marrow cells were plated at 5×105 cells/cm2 on 1 mg/L fibronectin (FN)-coated 6-well plates in bone marrow cells’ directed differentiated medium or cultural medium. The cells (on d 3, 6, 9, 12, 15, 18, and 21) were incubated in 2 mL medium containing 5 mmol/L NH4CL for 24 h in 5% CO2 at 37 °C. After incubation, the urea concentrations in the supernatant were measured. Mouse hepatocytes grown in the monolayer with the same density was used as positive control and culture medium used as nega tive control. No urea was detected in culture medium alone.
Results
Changes of cell amount and morphology The changes in cell amount and morphology could be seen in the course of the cell-directed differentiation. Bone marrow cells were inoculated at the density of 5×105 cells/cm2. However, when the nonadherent cells were removed 3 d later, we found that the adherent cells’ density was suitable for the adherent cells to grow well. On d 12, we could see some epithelial-like cells or polygonal cells in the directed differentiation medium, and the number and sizes of colonies of epithelial-like cells or polygonal cells increased in the course of the cell directed differentiation. On d 21, cells were detached and counted. The yield of the cells was approximately 8×103/cm2. In the negative group, we could see many fibroblast-like cells or fusiform cells, and only slight polygonal cells (Figure 1).
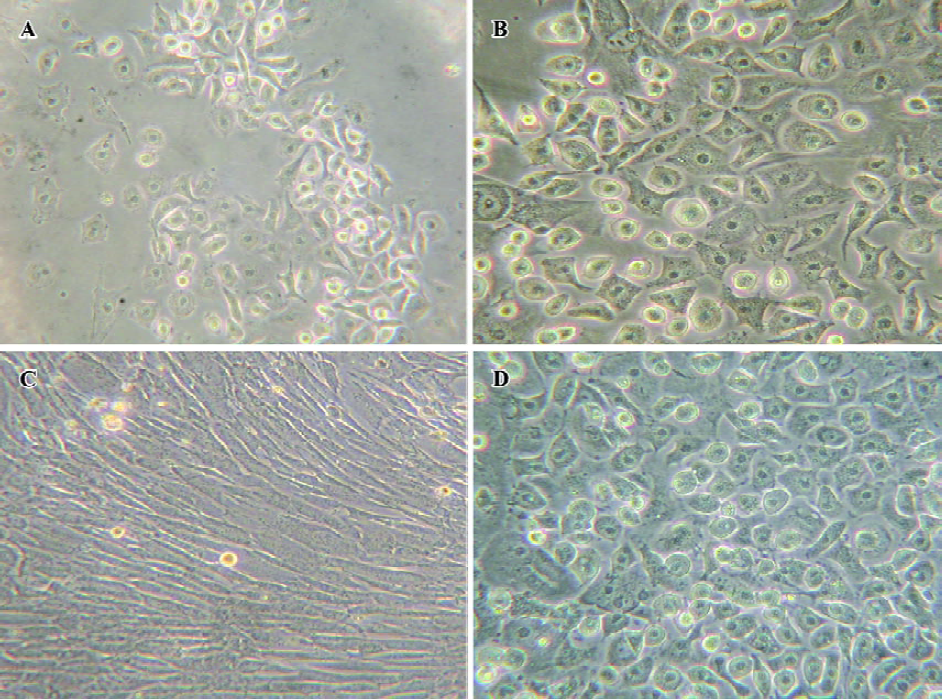
Gene expressions of liver specific markers To assess the directed differentiation of bone marrow cells into hepatic lineages, we first examined mRNA expressions of endodermal and liver specific genes including HNF-3β, ALB, CK18, TTR, G-6-Pase, and TAT, which could not be detected in fresh bone marrow cells and cultural bone marrow cells (Figure 2). In the bone marrow cells’ directed differentiation culture group, HNF-3β, ALB, and CK18 mRNA expressions first appeared within 6 d, and lasted throughout the later directed differentiation. TTR mRNA was expressed within 9 d, and its expression lasted throughout the later directed differentiation. From our research, we found that G-6-Pase and TAT mRNA expressions could be detected within 12 d, and their expressions lasted in the course of the later directed differentiation (Figure 2).
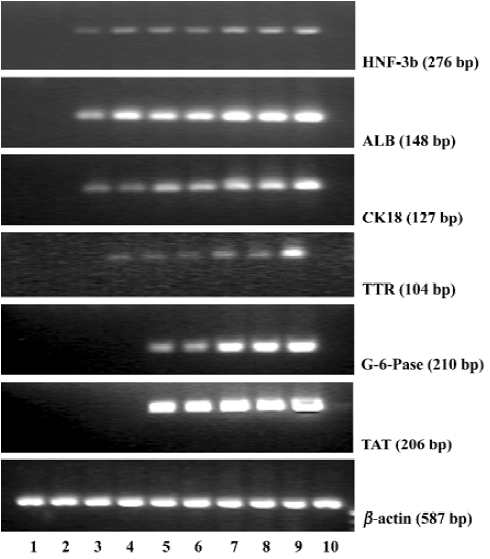
Protein expressions of liver specific markers We found that fresh bone marrow cells and cultural bone marrow cells on d 21 did not express any HNF-3β, ALB or CK18, but directed differentiated bone marrow cells on d 21 expressed HNF-3β, ALB, and CK18 (Figure 3).
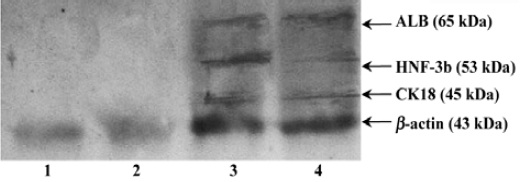
Hepatocyte functional activity ALB secretion was measured at various times throughout the cell differentiation. Undifferentiated bone marrow cells and cultural bone marrow cells did not secret any ALB. Following treatment with FGF-4 and OSM, directed differentiated bone marrow cells produced ALB in a time-dependent manner. On d 15, the amount of secreted albumin reached the maxium amount (Figure 4).
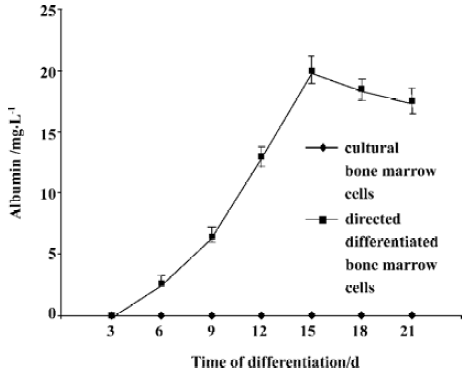
Intracellular glycogen accumulation, one feature of adult liver, was analyzed by staining the cells with the PAS reagent. A slight accumulation of glycogen was detected in the course of directed differentiation, while no accumulation of glycogen was found in the culture media without FGF-4 and OSM (Figure 5).
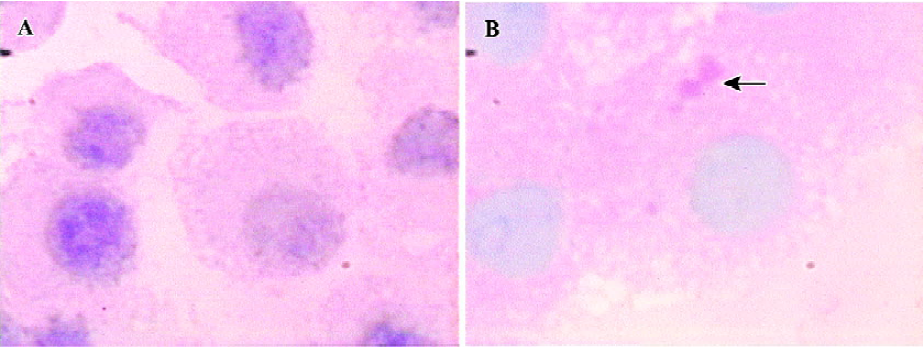
We then assessed urea production at various time point throughout the differentiation. Undifferentiated bone marrow cells and cultural bone marrow cells did not produce urea. In the directed differentiated group, directed differentiated bone marrow cells produced urea 3 d later, and in a time-dependent manner. On d 15, the amount of urea produced by directed differentiated bone marrow cells reached the maxium amount (Figure 6).
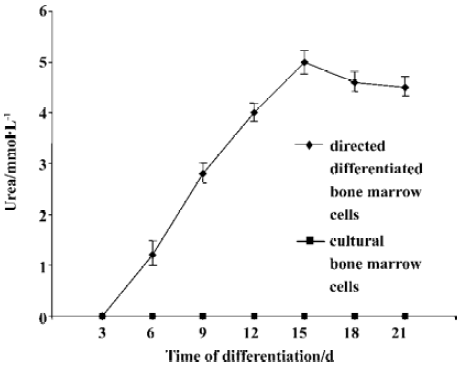
Discussion
In the present study, we selected bone marrow cells for further differentiation study because no matter hematopoietic stem cells, multipotent progenitor cells, and bone marrow stromal cells, they share common aspects. As bone marrow cells, they exist in the same bone marrow cell-cell microenvironment. They share the same character-stem cell plasticity, which means they have the ability to differentiate into cells of different tissue under certain microenviron-ments[20]. It has recently been founded by reseachers that there are ways of seperating hematopoietic stem cells and multipotent progenitor cells from bone marrow, but there is no good way to propagate hematopoietic stem cells in vitro. The multipotent progenitor cells could be propagated in vitro, but its cultural condition or requirement is very harsh[10]. In the present study, bone marrow stromal cells were shown to differentiate into hepatocyte-like phenotypes given the HGF induction, but these hepatocyte-like cells only expressed ALB and α-fetoprotein[11]. Furthermore, in the course of the organ development including the developing liver, cell-cell interactions are very important for modulating cell growth, migration, and/or differentiation, and are imperative for coordinated organ function[21]. A directed differentiated culture system, in which all bone marrow stem cells could differentiate into hepatocytes would be beneficial. From our study, we found that bone marrow cells grow easily and differentiate well in the induction of differentiation into hepatocytes.
Liver development is known to proceed through several distinct steps and many growth factors and cytokines are involved in each step. In mice, the initial event in liver development occurs at E9. The foregut endoderm becomes the liver through interaction with the cardiogenic mesoderm[22,23]. FGF-4 is involved in endoderm specification and hepatic differentiation in this step[14]. OSM, an interleukin (IL)-6 family cytokeratin, is a paracrine factor produced by hematopoietic cells and plays an important role in hepatic maturation during the mid to late fetal stages[15]. HGF has been shown to be important components of liver development and the differentiation process. However, HGF is different from OSM in inducing hepatic maturation, as it seems to be a paracrine factor that is involved in postnatal hepatic maturation[16]. Some other cytokines, including (EGF), insulin, TGF, appear to play important roles in liver development and regeneration[17]. The question is asked: in vitro, which cytokines will affect hepatocyte from the directed differentiation of stem cells? Some answers include: HGF [11,24,25], HGF and EGF[26], HGF and FGF[27]. We established a differentiation culture system including FGF-4, HGF, OSM, and EGF, where hepatocytes were in the presence of FGF-4, HGF, OSM and EGF in the cell culture system. (SHI et al, unpublished data). We then adopted a uniform design method to research the most effective density of each cytokine and get the most effective match of these cytokines. We have sifted through the most effective directed differentiation culture system including FGF-4 and OSM (SHI et al, in press). From our research, we used the most effective directed differentiation culture system and researched the differentiation of bone marrow cells into hepatocytes.
In the present study, we found that these hepatocyte-like cells expressed HNF-3β, ALB, CK18, TTR, G-6-Pase, and TAT at the gene level and/or protein level. HNF-3β is a hepatocyte nuclear factor, which occurs in the cell nucleus of the premature or mature hepatocyte. Expression of ALB, the abundant protein synthesized by mature hepatocytes, starts in early fetal hepatocytes and reaches the maximal level in adult hepatocytes[28]. CK18 is a cytoskeletal protein and is expressed in mature hepatocytes. TTR represents endodermal differentiation and is expressed throughout liver maturation[29]. G-6-Pase is predominantly expressed in the liver[28]. TAT represents an excellent enzymatic marker for peri- or postnatal hepatocyte-specific differentiation. Since hormone-regulated TAT activity is strictly limited to the parenchymal cells of the adult liver, it has been used extensively for monitoring cellular differentiation in experimental models for liver development/maturation in vitro[30]. Furthermore, these hepatocyte-like cells had some hepatocellular synthesis and metabolism functions, which would be used for hepatocyte transplantation or bioartificial liver.
Bone marrow stem cell can differentiate into hepatocytes, which is referred to as stem cell plasticity. There are many published studies explaining stem cell plasticity. First, multiple stem cells can coexist in multiple tissues, even after birth, and they proliferate and differentiate in respond to local stimulation[31]. Second, multipotent stem cells, which are akin to ES cells, persist past initial lineage specification[10]. Third, cells can undergo de- and re-differentiation[32]. Cell fusion has recently been suggested as another explanation of stem cell plasticity[33]. Irrespective of the explanations of stem cell plasticity, the fact that bone marrow stem cell can differentiate into hepatocyte in vitro holds great promise for the treatment of inherited and degenerative liver diseases.
From our research, we can conclude that our differentiation media, including FGF-4 and OSM, are effective for differentiating bone marrow cells into hepatocyte-like cells, which were identified at the gene level and protein level and had some hepatocellular synthesis and metabolism functions. Thus, we will serve these functional hepatocyte-like cells as hepatocyte resources for bioartificial liver or hepatocyte transplantation.
References
- Everhart JE, Lombardero M, Detre KM, Zetterman RK, Wiesner RH, Lake JR, et al. Increased waiting time for liver transplantation results in higher mortality. Transplantation 1997;64:1300-6.
- Suh KS, Lilja H, Kamohara Y, Eguchi S, Arkadopoulos N, Neuman T, et al. Bioartifical liver treatment in rats with fulminant hepatic failure: effect on DNA-binding activity of liver-enriched and growth-associated transcription factors. J Surg Res 1999;85:243-50.
- Malhi H, Gupta S. Hepatocyte transplantation: new horizons and challenges. J Hepatobiliary Pancreat Surg 2001;8:40-50.
- Weissman IL. Stem cells: units of development, units of regeneration, and units in evolution. Cell 2000;100:157-68.
- Petersen BE, Bowen WC, Patrene KD, Mars WM, Sullivan AK, Murase N, et al. Bone marrow as a potential source of hepatic oval cells. Science 1999;284:1168-70.
- Theise ND, Badve S, Saxena R, Henegariu O, Sell S, Crawford JM, et al. Derivation of hepatocytes from bone marrow cells in mice after radiation-induced myeloablation. Hepatology 2000;31:235-40.
- Alison MR, Poulsom R, Jeffery R, Dhillon AP, Quaglia A, Jacob J, et al. Hepatocytes from non-hepatic adult stem cells. Nature 2000;406:257.
- Theise ND, Nimmakayalu M, Gardner R, Illei PB, Morgan G, Teperman L, et al. Liver from bone marrow in humans. Hepatology 2000;32:11-6.
- Lagasse E, Connors H, Al-Dhalimy M, Reitsma M, Dohse M, Osborne L, et al. Purified hematopoietic stem cells can differentiate into hepatocytes in vivo. Nat Med 2000;6:1229-34.
- Jiang Y, Jahagirdar BN, Reinhardt RL, Schwartz RE, Keene CD, Gonzalea XR, et al. Pluripotency of mesenchymal stem cells derived from adult marrow. Nature 2002;418:41-9.
- Wang PP, Wang JH, Ya ZP, Hu MY, Lau GK, Fan ST, et al. Expression of hepatocyte-like phenotypes in bone marrow stromal cells after HGF induction. Biochem Biophys Res Commun 2004;320:712-6.
- Hemler ME, Rutishauser U. Cell-to-cell contact and extracelluar matrix. Curr Opin Cell Biol 2000;12:539-41.
- Birchmeier C, Brohmann H. Genes that control the development of migrating muscle precursor cells. Curr Opin Cell Biol 2000;12:725-30.
- Wells J.M., Melton DA. Vetebrate endoderm development. Annu Rev Cell Dev Biol 1999;15:393-410.
- Taga T, Kishimoto T. Gp130 and the interleukin-6 family of cytokines. Annu Rev Immunol 1997;15:797-819.
- Kamiya A, Kinoshita T, Miyajima A. Oncostatin M and hepatocute growth factor induce hepatic maturation via distinct signaling pathways. FEBS Lett 2001;492:90-4.
- Michalopeulos GK, DeFrances MC. Liver regeneration. Science 1997;276:60-6.
- Levite M, Meshorer A, Reisner Y. A rapid method for obtaining murine bone marrow cells in high yield. Bone Marrow Transpl 1991;8:225-7.
- Seglen PO. Preparation of isolated rat liver cells. Methods Cell Biol 1976;13:23-28.
- Verfaillie CM, Pera MF, Lansdorp PM. Stem cells: hype and reality. Hematology 2002.369-91.
- Bhatia SN, Balis UJ, Yarmush ML, Toner M. Effect of cell-cell interactions in preservation of cellular phenotype: cocultivation of hepatocytes and nonparenchymal cells. FASEB J 1999;13:1883-900.
- Zaret KS. Liver specification and early morphogenesis. Mech Dev 2000;92:83-8.
- Zaret KS. Hepatocyte differentiation: from the endoderm and beyond. Curr Opin Genet Dev 2001;11:568-74.
- She HO, Masahiro M, Hirosuke K, Yusuke I, Masakiyo S, Toshiya T, et al. Hepatocyte growth factor induces differentiation of adult rat bone marrow cells into a hepatocyte lineage in vitro. Biochem Biophys Res Commun 2000;279:500-4.
- Henning CF, Michael VL, Lourdes CD, Claudia L, Dietrich K, Boris F, et al. Liver-specific gene expression in cultured human hematopoietic stem cells. Stem Cells 2003;21:98-104.
- Masahiro M, Ichiro A, Masakiyo S, Emiko N, Mayumi O, Ken K, et al. Improved conditions to induce hepatocytes from rat bone marrow cells in culture. Biochem Biophys Res Commun 2002;298:24-30.
- Robert ES, Morayma R, Lisa K, Yuehua J, Mark B, Troy L, et al. Multipotent adult progenitor cells from bone marrow differentiate into functional hepatocyte-like cells. J Clin Invest 2002;109:1291-302.
- Pan CJ, Lei KJ, Chen H, Ward JM, Chou JY. Ontogeny of the murine glucose-6-phosphatase system. Arch Biochem Biophys 1998;358:17-24.
- Makover A, Soprano DR, Wyatt ML, Goodman DS. An in situ-hybridization study of the localization of retinol-binding protein and transthyretin messenger RNAs during fetal development in the rat. Differentiation 1989;40:17-25.
- Shelly LL, Tynan W, Schmid W, Schutz G, Yeoh GC. Hepatocyte differentiation in vitro: initiation of Tyrosine amino transferase expression in cultured fetal rat hepatocytes. J Cell Biol 1989;109:403-10.
- Kawada H, Ogawa M. Bone marrow origin of hematopoietic progenitors and stem cells in murine muscle. Blood 2001;98:2008-13.
- Wilmut I, Schnieke AE, Mcwhir J, Kind AJ, Campbell KH. Viable offspring derived from fetal and adult mammalian cells. Nature 1997;385:810-3.
- Ying QY, Nichols J, Evans EP, Smith AG. Changing potency by spontaneous fusion. Nature 2002;416:545-8.