Bradykinin potentiates 5-HT3 receptor-mediated current in rat trigeminal ganglion neurons
Introduction
Serotonin (5-HT), a major component of the inflammatory chemical milieu, is released from platelets, mast cells, or basophils that infiltrate an area of tissue damage[1]. Once released, 5-HT is free to interact with a number of molecularly distinct receptor subtypes, including the 5-HT3 receptor expressed in primary afferent nociceptors[2,3], and is capable of evoking pain and hyperalgesia[4]. To date, receptors for 5-HT can be classified into seven types and can be further distinguished into at least 13 subtypes. Unlike all other known 5-HT receptor subtypes, which are G-protein coupled, the 5-HT3 receptor is a member of the excitatory ligand-gated ion channel (LGIC) superfamily[5,6]. Researchers believe that 5-HT3 receptors located on sensory nerve terminals are mainly responsible for 5-HT-induced pain and hyperalgesia, although other subtypes of 5-HT receptors are also involved[4,7–10]. Recently we have demonstrated the potentiation of 5-HT3 receptor function by substance P and α-methyl-5-HT, an agonist of 5-HT2 receptor, through a protein kinase C (PKC)-dependent pathway in primary sensory neurons[11,12].
Bradykinin (BK) is an inflammatory mediator that plays a pivotal role in pain and hyperalgesia by exciting nociceptors and sensitizing them through activation of PKC[13]. BK responses are mediated by BK receptors. There are two main types of BK receptors, B1 and B2. B2 BK receptors are constitutively and abundantly expressed in primary sensory neurons[14]. The B2 BK receptor has been implicated in BK-induced nociceptor activities and nociceptive behaviors[15–17], and animals deficient in B2 BK receptors show hypoalgesia and reduced inflammatory responses[18,19]. The cDNA of the B2 BK receptor has already been cloned[20] and evidence for the contribution of the PKC pathway to the B2 BK receptor-mediated algesic action of BK has accumulated[17,21]. Thus, it is highly possible that 5-HT3 receptor function is also enhanced by BK through a PKC-dependent pathway. The present study aimed to explore whether the modulation of BK in 5-HT3 receptor-mediated current could occur in trigeminal ganglion (TG) neurons.
Materials and methods
Isolation of TG neurons Spraque-Dawley rats, 2–3 weeks old, were anesthetized with ether and decapitated. The TG were removed and transferred immediately into Dulbecco’s modified Eagle’s medium (DMEM, Sigma, St Louis, MO, USA) at pH 7.4. After removal of the surrounding connective tissues the TG were minced with fine spring scissors and the ganglion fragments were placed in a flask containing 5 mL DMEM in which trypsin (type II-S, Sigma) 0.5 g/L, collagenase (type I-A, Sigma) 1.0 g/L, and DNase (type IV, Sigma) 0.1 g/L had been dissolved, and incubated at 35 °C in a shaking water bath for 30–35 min. Soybean trypsin inhibitor (type II-S, Sigma) 1.25 g/L was added to stop trypsin digestion. Dissociated neurons were placed into a 35 mm Petri dish and kept for at least another 30 min before electrophysiological recording. The neurons selected for the patch-clamp experiment measured 20–45 μm in diameter.
Electrophysiological recordings Whole-cell patch-clamp recordings were carried out at room temperature (22–24 ºC) using a whole-cell patch-clamp amplifier (CEZ-2400, Nihon Kohden, Tokyo, Japan). Pipettes were filled with internal solution containing (in mmol/L): KCl 140, CaCl2 1, MgCl2 2.5, HEPES 10, egtazic acid 11, and ATP 5; the pH was adjusted to 7.2 with KOH and the osmolarity was adjusted to 310 mOsm/L with sucrose. Cells were bathed in an external solution containing (in mmol/L): NaCl 150, KCl 5, CaCl2 2.5, MgCl2 2, HEPES 10, and D-glucose 10; the osmolarity was adjusted to 340 mOsm/L with sucrose and the pH was adjusted to 7.4 with NaOH. The resistance of the recording pipette was in the range of 2–5 MΩ. A small patch of membrane underneath the tip of the pipette was aspirated to form a gigaseal and then more negative pressure was applied to rupture it, thus establishing a whole-cell configuration. The adjustment of capacitance compensation and series resistance compensation was done before recording the membrane currents. The holding potential was set at -60 mV, unless otherwise indicated. Membrane currents were filtered at 10 kHz (-3 dB), and the data were stored and analyzed in a computer with data acquisition software and hardware systems (Huazhong University of Science and Technology, Wuhan, China) and recorded using a pen recorder (Nihon Kohden).
Drug application Drugs used in the experiments included: serotonin hydrochloride (5-HT, Sigma), bradykinin (BK, Sigma), 2-methyl-5-hydroxytryptamine maleate (Research Biochemicals Incorporated, Natick, MA, USA), ICS -205,930 (Research Biochemicals Incorporated), Hoe 140 (Sigma), and GF 109203X (Research Biochemicals Incorporated). All drugs except GF 109203X were dissolved in the external solution just prior to use and held in a linear array of fused silica tubes (od/id=500/200 µm) connected to a series of independent reservoirs. The distance from the tube mouth to the cell examined was approximately 100 µm. The application of each drug was driven by gravity and controlled by the corresponding valve, and rapid solution exchange could be achieved by shifting the tubes horizontally with a micromanipulator. Cells were constantly bathed in normal external solution flowing from one tube connected to a larger reservoir between drug applications. In a number of the experiments GF 109203X needed to be applied intracellularly and was dissolved in the internal solution.
Data analysis Data were statistically analyzed using Student’s t-test or analysis of variance (ANOVA). Statistical analysis of the concentration-response data was carried out using the non-linear, curve-fitting program ALLFIT. Current values were expressed as mean±SEM.
Results
Current mediated by the 5-HT3 receptor in rat TG neurons In our experiments neurons freshly isolated from rat TG were round or elliptic in shape under light microscopy. The majority of the cells examined responded to 5-HT applied externally with a concentration (1×10-3–1×10-6 mol/L)-dependent inward current (76.3%, 74/97). This 5-HT-activated current (I5-HT, 1×10-4 mol/L) could be mimicked by 2-methyl-5-HT (1×10-4 mol/L), a specific 5-HT3 receptor agonist, and could be blocked by ICS-205,930 (1×10-6 mol/L), a selective antagonist of 5-HT3 receptor, indicating that this current was mediated by the 5-HT3 receptor (Figure 1A).
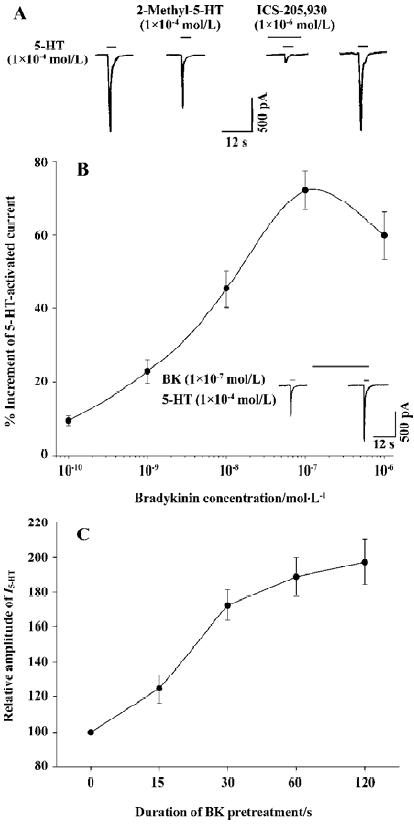
When 5-HT was applied regularly for 3-s durations with 3-min intervals, the I5-HT was repeated stably within at least 90 min, and the change in amplitude was within 8% (data not shown). Thus, we used this pattern of 5-HT applications in the following experiments.
Potentiation of I5-HT by pre-application of BK BK applied for 30 s prior to the application of 5-HT (1×10-4 mol/L) potentiated I5-HT reversibly in the majority of the neurons examined (66/74, 89.2%). In 18 of the 74 (24.3%) cells sensitive to 5-HT there was also a response to BK with a very small inward or outward current (<150 pA), which showed slow desensitization (data not shown). The BK potentiation of I5-HT was observed irrespective of whether BK evoked an inward or outward current, or no response. The I5-HT was potentiated by the pre-application of BK (1×10-6–1×10-10 mol/L) in a concentration-dependent manner. Figure 1B shows that with an increase in BK concentration from 1×10-10 to 1×10-6 mol/L, the amplitude of I5-HT (1×10-4 mol/L) increased stepwise until it reached its maximum at a concentration of 1×10-7 mol/L BK (71.6±4.9%). Thereafter this potentiating effect did not increase further, but rather decayed with further increases in BK concentration until 10-6 mol/L (Figure 1B).
Effect of the duration of BK pre-application on I5-HT To explore the relationship between the effect of BK on I5-HT and the duration of the pre-application of BK, different BK pre-application durations ranging from 15 to 120 s were tested. Figure 1C illustrates that the amplitude of I5-HT (1×10-4 mol/L) increased with increasing BK (1×10-7 mol/L) pre-application durations. With the duration of BK pre-application at 120 s, the amplitude of I5-HT increased (1.97±0.13)-fold compared with that of the control. However, there was no enhancing effect observed when 5-HT and BK were co-applied for 3 s (n=7; data not shown).
Effect of the B2 BK receptor antagonist Hoe 140 on BK potentiation of I5-HT To verify whether the BK potentiation of I5-HT was mediated by the receptor for BK, we examined the effect of the pre-application of both BK and Hoe 140, a selective B2 BK receptor antagonist, on I5-HT. The pre-application of both BK and Hoe 140 abolished BK-induced potentiation of I5-HT significantly (Figure 2A,B, paired t-test, P<0.01, n=7).
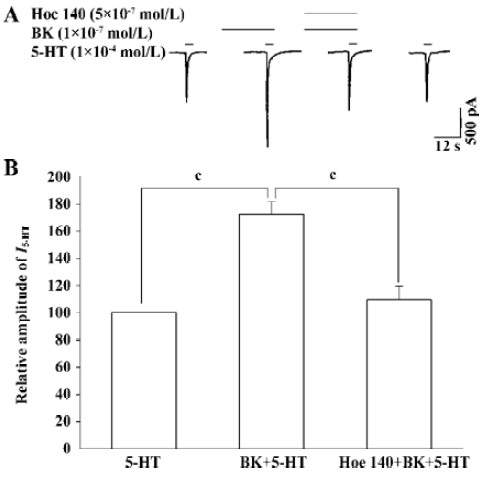
Concentration-response relationship for 5-HT with and without BK pre-application Figure 3A demonstrates the concentration-response curves for 5-HT with or without the pre-application of BK (1×10-7 mol/L). The threshold concentrations of 5-HT in the two concentration-response curves for 5-HT with or without BK pre-application were similar at approximately 3×10-6 mol/L; and the EC50 values were also very similar (19.1±3.2 µmol/L and 20.9±3.5 µmol/L; t-test; P>0.05; n=8); whereas the maximal response induced by 5-HT with BK pre-application increased by 68.9%±7.2% of that without BK pre-application. The present results reveal that the concentration-response curve for 5-HT pretreated with BK shifts upwards compared with the curve for 5-HT alone.
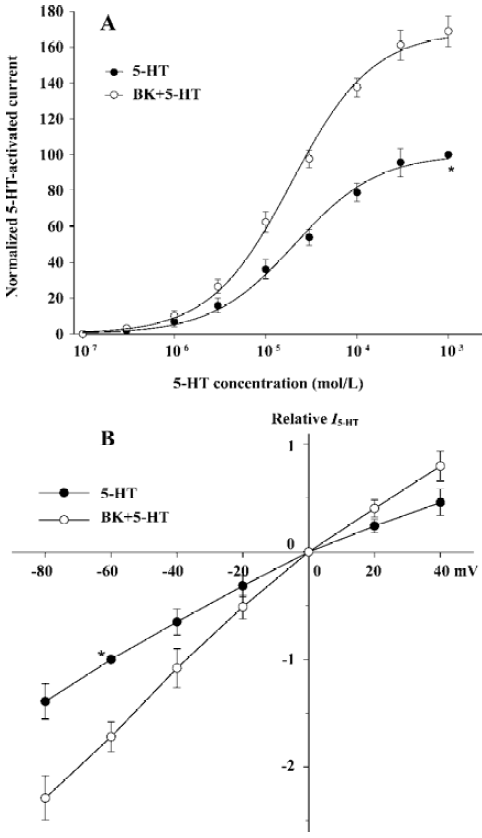
Current-voltage (I-V) relationship for I5-HT with or without BK pre-application I5-HT (1×10-4 mol/L) with or without the pre-application of BK (1×10-7 mol/L) was recorded at different holding potentials. All current values from the same cell were normalized to the current response induced by 5-HT alone at a holding potential of -60 mV when I-V curves were drawn (Figure 3B). The reverse potential values for the two curves were essentially the same at 0 mV. The amplitude of I5-HT with BK pre-application was greater than that without BK pre-application at all holding potentials from -80 to +40 mV, and the BK-induced alteration of I5-HT did not correlate with the change in holding potential (ANOVA; P>0.05, n=8), which suggests that the potentiation of I5-HT by BK occurs in a voltage-independent manner and the reverse potential of I5-HT is unchanged by BK.
Intracellular signal transduction mechanism underlying BK potentiation of I5-HT To explore whether this enhancing effect is mediated through BK-receptor-induced intracellular signal transduction, for example, activating PKC, GF-109203X, a selective PKC inhibitor[22], was included in the recording pipette for intracellular dialysis using the re-patch technique. In the control experiment with the pipette filled with normal internal solution, the BK-induced potentiation of I5-HT was 72.2%±5.2%. In contrast, when using a pipette filled with GF-109203X (2 µmol/L) containing internal solution the BK-induced potentiation of I5-HT was 13.5%±4.3%. It is evident that GF-109203X applied intracellularly removes the enhancing effect of BK on I5-HT (Figure 4).
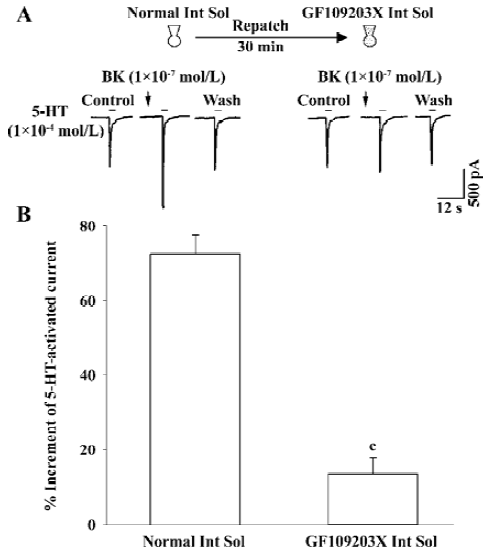
Discussion
The 5-HT-activated current we recorded from TG neurons was mediated by the 5-HT3 receptor, the sole ligand-gated ion channel (LGIC) in the family of 5-HT receptors, because it was blocked by ICS-205,930, a selective antagonist of the 5-HT3 receptor (Figure 1A). There was evidence to indicate that 5-HT3 receptors were present in rat TG neurons[23]. Similarly, B2 BK receptors were also expressed in TG neurons[14]. In the present study we recorded both B2 BK receptors and 5-HT3 receptors in TG neurons, and in the majority of these neurons (89.2%, 66/74) the pre-application of BK (1×10-7 mol/L) potentiated I5-HT (1×10-3–1×10-6 mol/L). This potentiation was mediated by B2 BK receptors because the selective B2 BK receptor antagonist Hoe 140 blocked this potentiating effect, obviously and reversibly (Figure 2). However, a previous study has reported that the inflammatory mediators BK, 5-HT, and prostaglandin E2 do not cooperate to elevate intracellular calcium concentration when applied simultaneously for 10 s in cultured dorsal root ganglion neurons[24]. The distinction possibly results from different observation indices and specimens, or may be caused by the different treatment of BK. In the present experiment, there was also no enhancing effect observed when 5-HT and BK were co-applied for 3 s, whereas I5-HT was potentiated by the pre-application of BK for more than 15 s (Figure 1C).
It is evident from Figure 1B that the enhancement of amplitude of I5-HT increased gradually with incremental increases in the concentration of BK from 10-10 to 10-7 mol/L. However, when the concentration of BK increased to 10-6 mol/L the modulatory effect of BK on I5-HT did not increase further. The decrease in potentiation of I5-HT by BK (10-6 mol/L) might be a non-specific action of the agonist that emerges at high concentrations because very high concentrations of drug or ligand may block the channel and/or shelter the binding site of the receptor[25].
From the comparison between the concentration-response curves for 5-HT with and without the pre-application of BK (Figure 3A) it is clear that: (i) pre-application of BK shifted the curve upwards; (ii) the maximal response induced by 5-HT with BK pre-application increased by 68.9%, whereas the threshold concentrations of 5-HT in both cases were similar; and (iii) the EC50 values of the two curves were very close (19.1±3.2 µmol/L vs 20.9±3.5 µmol/L). This implies that the intrinsic efficacy of the 5-HT3 receptor increases after pretreatment with BK; however, its affinity does not change.
From the I-V curves for I5-HT with and without BK pre-treatment, it can be seen that the reverse potentials were the same (0 mV), indicating that there was no change in the ionic components mediating this current. This enhancement was not caused by the release of the channel blocker, as is the case in the voltage-dependent Mg2+ block of NMDA-gated ion channel, because the BK-induced alteration of I5-HT was not correlated with the change in holding potential (Figure 3B). This implies that the potentiation of I5-HT by BK occurs in a voltage-independent manner.
The potentiation of I5-HT by BK may involve intracellular signal transduction because there was no enhancing effect observed when 5-HT and BK were co-applied; nevertheless, BK applied prior to 5-HT application induced the enhancement of I5-HT and this effect was positively related to the duration of BK pretreatment, implying that this enhancement is a time-consuming process. Furthermore, this potentiation was blocked by Hoe 140, a selective B2 BK receptor antagonist. B2 BK receptors belong to the superfamily of G-protein-coupled receptors (GPCR)[20]. When activated by BK, the B2 BK receptor is coupled to PLCβ1 via Gq/11 protein; which in turn catalyzes PIP2 into secondary messengers, IP3 and DAG. The latter activates PKC. Reports have shown that 5-HT3 receptor function is enhanced by the activation of PKC[26,27]. In the present experiment, the enhancing effect of BK on I5-HT was evidently blocked by intracellular dialysis of GF-109203X, a selective PKC inhibitor[22], indicating that potentiation occurs via a PKC-dependent pathway. How does PKC affect the function of 5-HT3 receptors? Recently, a novel mechanism for 5-HT3 receptor modulation by the activation of PKC was demonstrated[28]; that is, the PKC-induced potentiation of 5-HT3 receptor mediated current in Xenopus oocytes and mouse NIE-115 neuroblastoma cells resulted from the enhancement of F-actin-dependent trafficking of 5-HT3 receptors instead of direct phosphorylation of the 5-HT3A receptor protein.
What is the physiological significance of this BK modulation on I5-HT or 5-HT3 receptor function? In this work we used the cell body of TG neurons as a simple and accessible model to examine the characteristics of the membrane of peripheral terminals. The nerve endings of the peripheral axon of primary sensory neurons, including TG neurons, are sensitive to many inflammatory chemical mediators, of which BK and 5-HT are two potent stimulating mediators. In the case of inflammation and/or tissue damage these two substances are released. On the one hand, they exert a stimulating effect on the nerve endings and initiate nociceptive information through their corresponding receptors located on the membranes of separate nerve endings. On the other hand, in the present study we found that in the case of coexistence of B2 BK receptors and 5-HT3 receptors in TG neurons the inward current mediated by the 5-HT3 receptor could be strengthened by pretreatment with BK, indicating that B2 BK receptors and 5-HT3 receptors may “cross-talk” in producing algesic information at nociceptors. Behavioral experiments have also demonstrated that 5-HT causes marked potentiation of BK-induced pain responses through 5-HT3 receptors[29]. The present study may provide a hint for explaining the peripheral mechanism of pain and hyperalgesia caused by, for example, tissue damage and inflammation.
References
- Dray A. Inflammatory mediators of pain. Br J Anaesth 1995;75:125-31.
- Martin GR, Eglen RM, Hamblin MW, Hoyer D, Yocca F. The structure and signaling properties of 5-HT receptors: an endless diversity? Trends Pharmacol Sci 1998;19:2-4.
- Hamon M, Bourgoin S. Serotonin and its receptors in pain control. In: Sawynok J, Cowan A, editors. Novel aspects of pain manage-ment: opioids and beyond. New York: Wiley; 1999. p 203–28.
- Dock GJ, Sawynok J. Formalin-induced nociceptive behavior and edema: involvement of multiple peripheral 5-hydroxytrypta-mine receptor subtypes. Neuroscience 1997;80:939-49.
- Maricq AV, Peterson AS, Brake AJ, Myers RM, Julius D. Primary structure and functional expression of the 5-HT3 receptor, a serotonin-gated ion channel. Science 1991;254:432-7.
- Davies PA, Pistis M, Harma MC, Peters JA, Lambert JJ, Hales TG, et al. The 5-HT3B subunit is a major determinant of serotonin–receptor function. Nature 1999;397:359-63.
- Orwin JM, Fozard JR. Blockade of the flare response to intradermal 5-hydroxytryptamine in man by MDL72222, a selective antagonist at neuronal 5-hydroxytryptamine receptors. Eur J Clin Pharmacol 1986;30:209-12.
- Eschalier A, Kayser V, Guilbaud G. Influence of a specific 5-HT3 antagonist on carrageenan-induced hyperalgesia in rats. Pain 1989;36:249-55.
- Giordano J, Rogers LV. Perpherally administered serotonin 5-HT3 receptor antagonists reduce inflammatory pain in the rats. Eur J Pharmacol 1989;170:83-6.
- Zeitz KP, Guy N, Malmberg AB, Dirajlal S, Martin WJ, Sun L, et al. The 5-HT3 subtype of serotonin receptor contributes to nociceptive processing via a novel subset of myelinated and unmyelinated nociceptors. J Neurosci 2002;22:1010-9.
- Hu WP, You XH, Guan BC, Ru LQ, Chen JG, Li ZW. Substance P potentiates 5-HT3 receptor mediated current in rat trigeminal ganglion neurons. Neurosci Lett 2004;365:147-52.
- Hu WP, Guan BC, Ru LQ, Chen JG, Li ZW. Potentiation of 5-HT3 receptor function by the activation of coexistent 5-HT2 receptor in trigeminal ganglion neurons of rats. Neuropharmacology 2004;47:833-40.
- Sugiura T, Tominaga M, Katsuya H, Mizumura K. Bradykinin lowers the threshold temperature for heat activation of vanilloid receptor 1. J Neurophysiol 2002;88:544-8.
- Steranka LR, Manning DC, DeHaas CJ, Ferkany JW, Borosky SA, Connor JR, et al. Bradykinin as a pain mediator: receptors are localized to sensory neurons, and antagonists have analgesic actions. Proc Natl Acad Sci USA 1988;85:3245-9.
- Ferreira J, da Silva GL, Calixto JB. Contribution of vanilloid receptor to the overt nociception induced by B2 kinin receptor activation in mice. Br J Pharmacol 2004;141:787-94.
- Dray A, Perkins M. Bradykinin and inflammatory pain. Trends Neurosci 1993;16:99-104.
- Mizumura K. Natural history of nociceptor sensitization: the search for a peripheral mechanism of hyperalgesia. Pain Rev 1998;5:59-82.
- Boyce S, Rupniak NM, Carlson EJ, Webb J, Borkowski JA, Hess JF, et al. Nociception and inflammatory hyperalgesia in B2 bradykinin receptor knockout mice. Immunopharmacology 1996;33:333-5.
- Seabrook GR, Bowery BJ, Heavens R, Brown N, Ford H, Sirinathsinghi DJ, et al. Expression of B1 and B2 bradykinin receptor mRNA and their functional roles in sympathetic ganglia and sensory dorsal root ganglia neurons from wild-type and B2 receptor knockout mice. Neuropharmacology 1997;36:1009-17.
- Ma JX, Wang DZ, Chao L, Chao J. Cloning, sequence analysis and expression of the gene encoding the mouse bradykinin B2 receptor. Gene 1994;149:283-8.
- Cesare P, Dekker LV, Sardini A, Parker PJ, McNaughton PA. Specific involvement of PKC-epsilon in sensitization of the neuronal response to painful heat. Neuron 1999;23:617-24.
- Toullec D, Pianetti P, Coste H, Bellevergue P, Grand-Perret T, Ajakane M, et al. The bisindolylmaleimide GF 109203X is a potent and selective inhibitor of protein kinase C. J Biol Chem 1991;266:15771-81.
- Zhu M, Wu SX, Wang W, Li YQ. Expression of 5-HT receptor subtype mRNAs in the rat trigeminal and spinal dorsal root ganglia: a polymerase chain reaction study. Chin J Neuroanat 2000;16:107-12.
- Linhart O, Obreja O, Kress M. The inflammatory mediators serotonin, prostaglandin E2 and bradykinin evoke calcium influx in rat sensory neurons. Neuroscience 2003;118:69-74.
- Arias HR. Binding sites for exogenous and endogenous non-competitive inhibitors of the nicotinic acetylcholine receptor. Biochim Biophys Acta 1998;1376:173-220.
- Coultrap SJ, Machu TK. Enhancement of 5-hydroxytryptamine3A receptor function by phorbol 12-myristate, 13-acetate is mediated by protein kinase C and tyrosine kinase activity. Receptor Channels 2002;8:63-70.
- Zhang L, Oz M, Weight FF. Potentiation of 5-HT3 receptor-mediated responses by protein kinase C activation. Neuroreport 1995;6:1464-8.
- Sun H, Hu XQ, Moradel EM, Weight FF, Zhang L. Modulation of 5-HT3 receptor-mediated response and trafficking by activation of protein kinase C. J Biol Chem 2003;278:34150-7.
- Richardson BP, Engel G, Donatsch P, Stadler PA. Identification of serotonin M-receptor subtypes and their specific blockade by a new class of drugs. Nature 1985;316:126-31.