Effects of complete Freund's adjuvant on immunohistochemical distribution of IL-1β and IL-1R I in neurons and glia cells of dorsal root ganglion1
Introduction
Interleukin-1 beta (IL-1β) is a bioactive polypeptide cytokine that is produced by a large variety of cells, including macrophages, fibroblasts, keratinocytes, synovicytes, mast cells, glial cells, and neurons; and has various immune roles responding to antigens, malignant cells, inflammatory stimuli, and tissue injury[1]. IL-1β also has proinflammatory cytokine activity and induces hyperalgesia after subcutaneous injection into the rat paw[2] or after intrathecal administration in rats and mice[3,4]. This means administration of exogenous IL-1β can produce hyperalgesia. However, inflammation can also induce the release of endogenous IL-1β which was responsible for hyperalgesia as well. A previous study showed that intraplantar injection of complete Freund’s adjuvant (CFA) in adult rats resulted in a significant elevation in IL-1β and nerve growth factor (NGF) levels in the inflamed tissue[5–6]. IL-1β exerts its effects through high-affinity receptors on target cells, of which two types have been identified: IL-1RI and IL-1RII[7]. IL-1 receptor antagonist (IL-1Ra), has been shown conclusively to block the effects of IL-1β[8]. Targeted deletion of the IL-1 receptor type I or the IL-1 receptor accessory protein, and transgenic over-expression of IL-1Rα within the brain and spinal cord significantly lowered thermal and mechanical pain sensitivity in mice[9].
The major cellular source of IL-1β production in the central nervous system are from glial cells, predominantly microglia. However, several studies have provided evidence for the neuronal expression of IL-1β in the peripheral nervous system: sensory neurons in the dorsal root ganglia (DRG) as well as sympathetic neurons[10]. Moreover, cytokines released in the periphery by sensory and by sympathetic neurons have an established role in regulating immune cell activation, connective tissue and vascular responses, and nociception in peripheral inflammatory processes. Among the cytokines produced and released by both sensory and sympathetic neurons are transforming growth factor-β (TGF-β), tumor necrosis factor-alpha (TNF-α), and interferon-gama (IFN-γ)[11,12]. Sympathetic neurons have also been demonstrated to express IL-1β[13,14]. IL-1β appears to be an important mediator in nocicepsis and has been shown to induce the release of substance P from nociceptive sensory neurons[15]. The expression of IL-1β as well as the type I IL-1 receptor in sensory neurons in rat DRG has been investigated using nonradioactive in situ hybridization and immunohistochemistry[16].. However, there is no morphological evidence for the coexistence of IL-1β and IL-1RI of neurons and glia cells in DRG and the effects of articular injection of CFA.
In the present study, we investigated the effects of CFA on inflammatory hyperalgesia and the morphological changes of IL-1β and IL-1RI coexistence in neurons and glia cells of rat DRG.
Materials and methods
Animals Experiments were carried out on male adult Sprague-Dawley rats (180–200 g), purchased from the Experimental Animals Center of Tongji Medical College of Hua-zhong University of Science and Technology (Grade II, Certificate N
Preclinical tests The following parameters were measured: (1) weight; (2) mobility score[19] (0=the rat only lies down; 1=the rat only crawls; 2=the rat walks with difficulty; 3=the rat walks and runs with difficulty; 4=the rat walks and runs normally); (3) diameter of the tibiotarsal joint, detected with vernier caliper.
Pain behavioral parameters The following pain parameters were measured using teo tests in the following sequence[20]: (1) flexion scores: mean scores of rats was evaluated by flexion of one hind paw. Under these conditions a squeak or leg-withdrawal could be elicited. Five such stimuli were repeated at 5-s intervals, and a rating of 1 or 0 was respectively given depending on whether the animal emitted a squeak or leg-withdrawal. Thus for each animal the total scores of squeak and leg-withdrawal ranged from 0 to 10; (2) extension scores: mean scores of rats as evaluated by extension of one hind paw (identical rating used as for flexion score).
Each group animals were tested before injection to obtain baseline value and continued to be detected in 5 h, 1 d, and 2 d post-injection.
Tissue preparation On d 3 post-injection, all animals were deeply anesthetized with 20% urethane (1 g/kg) by abdominal injection, cleared with 200 mL of saline solution and perfused with 300 mL of 4% phosphate-buffered paraformaldehyde (pH 7.4) through left ventricle. After perfusion, the L4-L5 ipsilateral DRG was removed and post-fixed for 8-10 h. They were then soaked in 20% sucrose phosphate-buffered solution at 4 ºC overnight prior to freezing and subsequent cryosectioning. Sections (10 µm) were cut on a cryostat.
The sections were put in 0.01 mol/L PBS buffered solution. Sections were selected randomly to do experiments with double immunofluorescence staining of IL-1RI/IL-1β, or IL-1RI immunohistochemistry single staining procedure.
Double immunofluorescence staining procedure We used the following steps: (1) sections were incubated with 0.25% Triton X-100 for 30 min at 37 ºC; (2) after being rinsed by 0.01 mol/L PBS, sections were incubated with 15% normal goat serum for 30 min at 37 ºC; (3) sections were then incubated for 48 h at 4 ºC in a mixture of the two primary antibodies (rabbit anti-rat IL-1RI, 1:100, Santa Cruz; mouse anti-rat IL-1β, 1:100, Serotec); (4) after being rinsed in PBS, sections were incubated for 3 h at room temperature in a mixture of the two secondary antibodies, Cy3-conjugated anti-rabbit IgG (Sigma, 1:200) and FITC-conjugated anti-mouse IgG (Sigma, 1:200); (5) the sections were picked and mounted on slides with glycerin-PBS buffered solution (1:9).
Omission of the primary antibody and incubation in IL-1β or IL-1RI pre-absorbed antibody served as negative controls. Laser scanning confocal microscope (Lesia, German) was used to take photos and fluorescent microscope (Olympus, Japan) was used to capture images and count positive cells.
IL-1RI immunohistochemistry procedure We used following steps: steps (1) and (2) were the same as above double immunofluorescence stain procedure; (3) sections were then incubated for 48 h at 4 ºC in the primary antibodies (rabbit anti-rat IL-1RI, Santa Cruz,1:200). (4) after being rinsed in PBS, sections were incubated for 30 min at room temperature of the secondary antibodies, biotinylated goat anti-rabbit IgG (Santa Cruz, 1:200); (5) after being thoroughly washed in PBS, the sections were incubated in a streptavidine-peroxidase conjugate (Santa Cruz, 1:200) for 30 min at room temperature; (6) after being rinsed with 0.05 mol/L Tris-buffered saline, the immunoreaction product was revealed by a freshly prepared solution containing 0.04% 3,3-diamino-benzidine and 0.01% H2O2. The sections were picked up and mounted on slides with glutin. They were dehydrated with graded alcohol and transparentized with xylene, then covered with neutral gum. Each experiment had set up blank antitheses as described before.
Statistic analysis Seven rats per group were used for analysis. Digital images were captured in four sections per animal and 10 squares (38.4 mm2 each) per section of immunofluorescence or immunohistochemistry respectively, using an Olympus microscope (objective×20; eyepiece×10) and a 3CC immunohistochemistry-D color video camera (Sony). The number of immunofluorescence-stained IL-1RI/IL-1β positive neurons or immunohistochemistry-stained IL-1RI positive glia cells in each image were counted by a blinded experimenter with a computer-assisted image analyzer (Image Pro-plus Kodak, USA). The number of positive DRG large-, medium-, and small-sized neurons were calculated. As reported previously, the primary sensory neurons in the DRG have been classified into large type A neurons (diameter over 30 µm), medium diameter neurons type B, and small type C neurons (diameter under 20 µm)[21]. For large neurons were regarded as medium sized ones when they were not cut through their nucleus. In our study we calculated the total of medium and large neurons of immunofluorescence-stained IL-1RI/IL-1β positive neurons. The SPSS software (SPSS 11.0) was used for data analysis. The data was expressed as mean±SD, and analysis of variance (ANOVA) was used to compare the difference among different groups.
Results
Preclinical and behavioral studies After 5 h, 1 d, and 2 d of intra-articular injection of 50 µL CFA, the weight of the rats was unchanged (P>0.05, data not shown). The rats of the AA group developed inflammation which was confined to the inoculated articular characterized by edema of ipsilateral tibiotarsal joint (Table 1). There was a dramatic decrease observed in the mobility scores of rats of the AA group compared with the NS group (P<0.05, P<0.01, Table 2). In other words, the inoculated rats are impaired in their ambulatory activities.
Effects of CFA on the pain behavioral parameters of rats Five hours, 1d, and 2 d after intra-articular injection of 50 µL CFA, tactile hyperalgesia induced by CFA was observed in the foot flexion and extension scores of ipsilateral hindpaw of AA group (Table 3, 4), while the pain scores of the contralateral hindpaw of both groups were close to 0 and had no significant difference (P>0.05, data not shown).
Effects of CFA on double immunofluorescence staining of IL-1β and IL-1RI of ipsilateral DRG Three days after injection, IL-1β and IL-1RI positive products were detected in the same neurons as well as in some glial-like cell types. IL-1β/IL-1RI immunoreactivity positive products were mainly located in the cytoplasma and nucleus of the positive neurons of NS group (Figure 1), while immunoreactivity positive products were distributed in the membrane and nucleus of the positive neurons of the AA group (Figure 2). There were still a few neurons presented no IL-1β or IL-1RI immunoreactivity in the DRG of the NS group, but most neurons in the DRG of AA group presented IL-1β or IL-1RI immunoreactivity (Figure 1C, 2C).
With statistic analysis, it was found that the number of IL-1RI single stained neurons, IL-1β/IL-1RI double-stained neurons, and the percentages of the number of double-stained neurons to the number of IL-1β positive neurons of small neurons in DRG of AA group were higher than that of NS group (P<0.05 or P<0.01, Table 5). The number of IL-1RI single stained neurons, IL-1β/IL-1RI double-stained neurons of large and medium neurons in DRG of AA group were higher than that of NS group (P<0.05, Table 6). The percentage of double-stained neurons to IL-1β or IL-1RI positive neurons of large and medium neurons in DRG presented no significant difference between the two groups (P>0.05, Table 6).
Effects of CFA on single immunohistochemistry staining of IL-1RI of ipsilateral DRG Three days after injection, the IL-1RI in DRG was expressed in glial-like cell types as well as in sensory neurons using a microscope under normal light. Some of the glial-like cells were satellite cells ensheathing the large sensory cell bodies, some sparsely distributed in the connective tissues between neurons of DRG. The IL-1RI immunoreactivity positive products were stained as brown and mainly located in the membrane and cytoplasma of glia cells. The stained color of glia cells in DRG of AA group was deeper than that of the NS group (Figure 3, 4). The number of glia cells of DRG of AA group was 13.45±2.35/38.4 mm2, which was higher than that of NS group (5.31±1.58/38.4 mm2) (P<0.01).
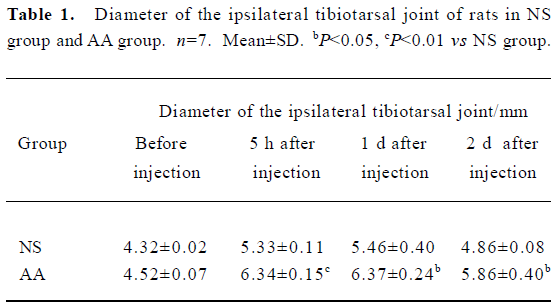
Full table
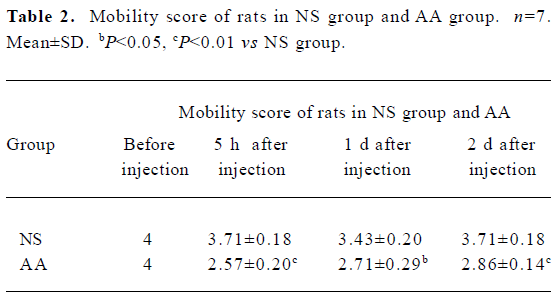
Full table
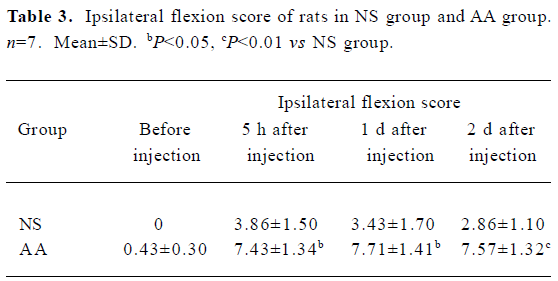
Full table
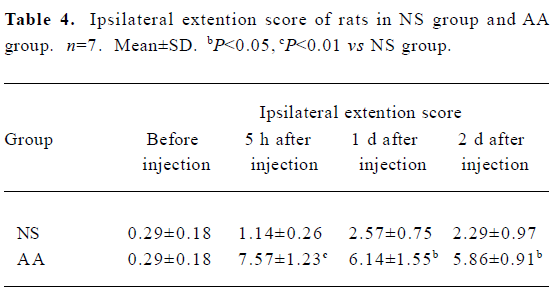
Full table
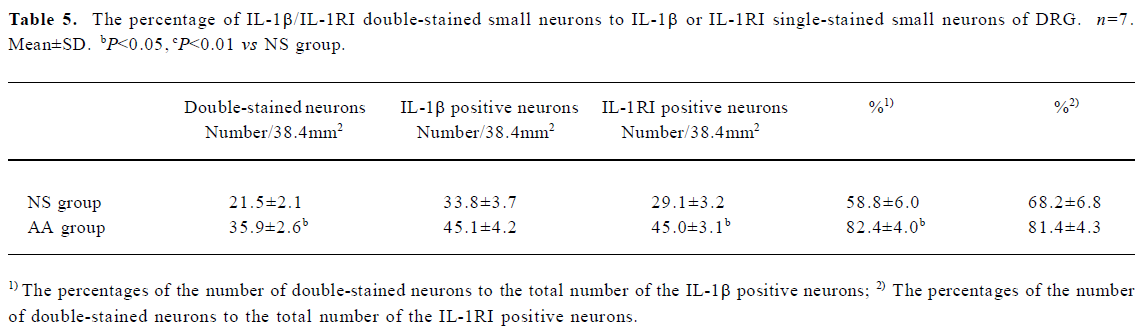
Full table
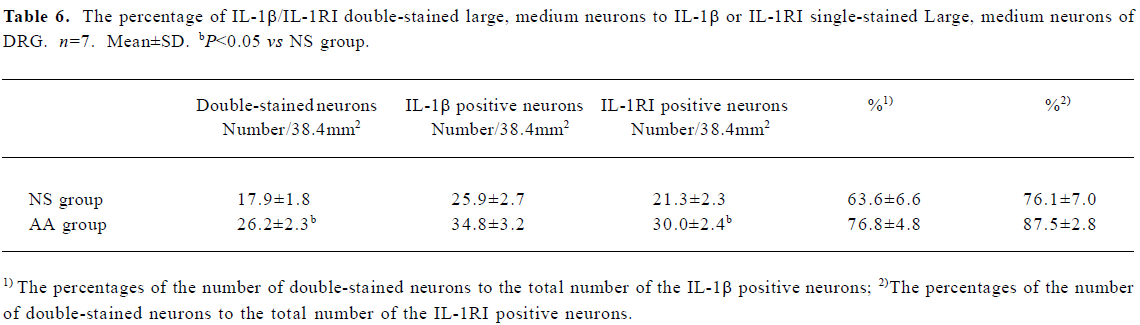
Full table
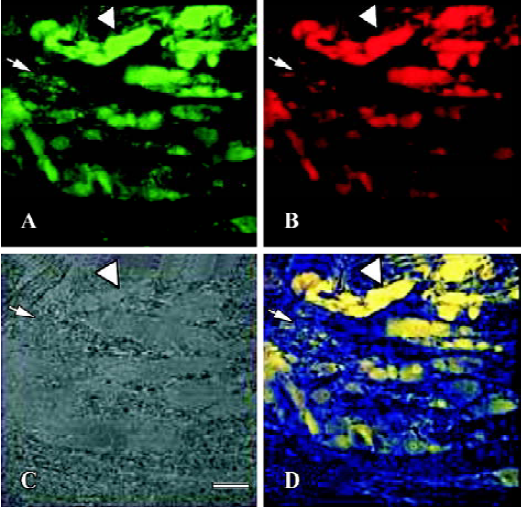
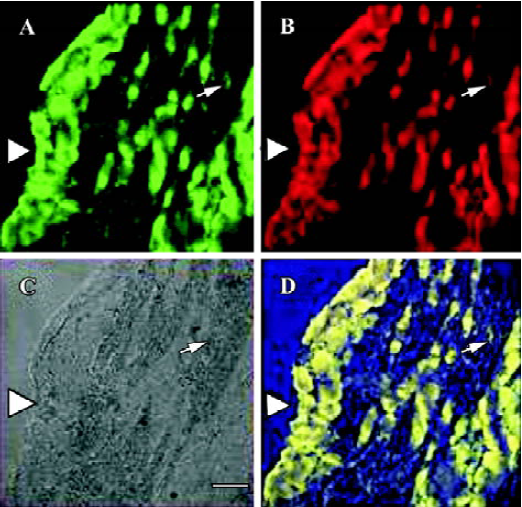
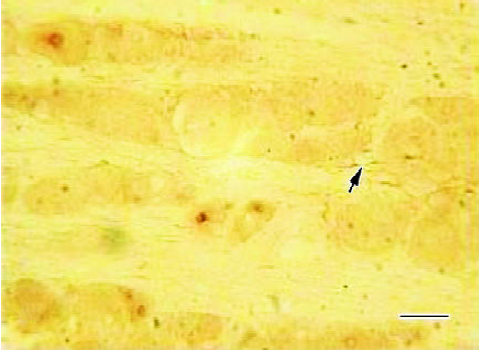
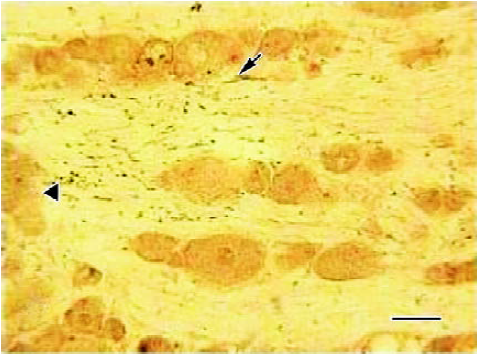
Discussion
IL-1β is one of the cytokines synthesized and released from immunocytes and considered to be an important mediator during inflammation and hyperalgesia. Indeed, the injection of IL-1β into animals has been shown to produce hyperalgesia in response to mechanical and thermal stimuli [2–4,22]. There are several lines of evidence indicating that IL-1β alters primary afferent neural activity or sensitivity in mice and rats[10,23–25]. Nociceptive primary afferent neurons contain neuropeptides such as SP, neurokinin A, and calcitonin gene-related peptide that are released on stimulation of these afferent fibers. A previous study of cellular culture experiment demonstrated that IL-1β can induce SP to release from cultured DRG by activating the COX-2 system. IL-1R antagonist can block this access, which suggests an IL-1β exerted effect by combine with IL-1R on DRG[15]. Using RT-PCR with in situ hybridization, it was found that type I IL-1R, not type II, expressed on the DRG of normal rats[26]. However, there is no study on the coexistence of IL-1RI and IL-1β in neurons and glia cells of DRG and the influence of adjuvant-induced arthritic on it.
In the present study, we demonstrated that both IL-1RI and IL-1β coexsisted in the sensory neurons and glia cells of normal saline injected rats. The combined expression of IL-1β and the type I IL-1 receptor suggests autocrine or paracrine IL-1β signaling in sensory neurons.
Meanwhile a significant increase in the IL-1RI and IL-1β double stained neurons in ipsilateral DRG were observed in the AA group than in the NS group, and IL-1RI mainly distributed in the membrane of the AA group than in the cytoplasma of NS group. This morphological change also paralleled to the pain behaviour scores. It indicated that the inflammation resulted in the up-regulation of the expression of IL-1RI on the membrane of neurons in ipsilateral DRG, and the increase of IL-1β sythesis as well.
It has been found that intraplantar injection of CFA increased the concentration of IL-1β and NGF accompanied by elevation of the level of substance P and calcitonin gene-related peptide (CGRP) in the L4 dorsal root ganglion 48 h post CFA injection[5]. The author demonstrated that IL-1β could alter neuropeptide expression in primary sensory neurons secondary to an increase of NGF levels as NGF has well known actions on the regulation of both SP and CGRP in adult rat DRG neurons[6]. It has been recently proved that IL-1β increases SP synthesis by enhancing the expression of preprotachykinin mRNA encoding for SP and other tachykinins in the DRG[27]. Our results showed that IL-1β could be synthesized in neurons of DRG, as wells as in the inflamed tissue from previous study, it indicated that more IL-1β was able to release by neurons of DRG and excite more IL-1RI, which presumably brought about more SP synthesis and release in the central and peripheral terminals of neurons in the DRG, which in turn led to hyperalgesia.
We also found that the number of glia cells in ipsilateral DRG, which express IL-1RI, increased after CFA injection. The expression of type I IL-1 receptor in non-neuronal cells in the DRG might mediate another possible function of IL-1β synthesized by the sensory neurons. It has been reported that IL-1β enhances neurite outgrowth in DRG[28], which would contribute to tactile hypersensitivity of articular arthritis. This effect of IL-1β seems to be indirect and presumably involves an IL-1β-induced increased in release of neurotrophins from nonneuronal cells[29]. It could be that, under inflammatory condition sensory neurons regulate their own maintenance by releasing more IL-1β and thus stimulating their environment to release neurotrophins to induce hyperalgesia.
Our study showed that IL-1β/IL-1RI doubled stained immunoreactivity positive products were located in the nucleus as well as the cytoplasma or membrane of positive neurons in ipsilateral DRG of both groups. This is consistent with the results of immunoelectron microscopy, which found that GSSP-intensified IL-1β immunoreaction products were found dispersed in small aggregates throughout the cytoplasm and a few small immuno-aggregates of IL-1β were also found in the nucleus of neurons in DRG. The functional significance of intranuclear IL-1β/IL-1RI is as yet unclear, but might suggest involvement in transcriptional regulation[16].
In conclusion, we found coexistence of IL-1β and IL-1RI in sensory neurons and glia cells of DRG. The presence of the type I IL-1 receptor in neurons and non-neuronal cells suggests an as yet unknown autocrine and/or paracrine function of IL-1β in the DRG. The function was enhanced in the articular arthritis induced by CFA and might play an important role in hyperalgisia under the inflammatory condition.
Footnote
Project supported by the grant of Major Research Plan of National Natural Science Foundation of China (N
References
- Cunha FQ, Ferreira SH. Peripheral hyperalgesic cytokines. Adv Exp Med Biol 2003;521:22-39.
- Sachs D, Cunha FQ, Poole S, Ferreira SH. Tumour necrosis factor-alpha, interleukin-1beta and interleukin-8 induce persistent mechanical nociceptor hypersensitivity. Pain 2002;96:89-97.
- Sung CS, Wen ZH, Chang WK, Ho ST, Tsai SK, Chang YC, et al. Intrathecal interleukin-1beta administration induces thermal hyperalgesia by activating inducible nitric oxide synthase expression in the rat spinal cord. Brain Res 2004;1015:145-53.
- Tadano T, Namioka M, Nakagawasai O, Tan-No K, Matsushima K, Endo Y, et al. Induction of nociceptive responses by intrathecal injection of interleukin-1 in mice. Life Sci 1999;65:255-61.
- Safieh-Garabedian B, Poole S, Allchorne A, Winter J, Woolf CJ. Contribution of interleukin-1 beta to the inflammation-induced increase in nerve growth factor levels and inflammatory hyper-algesia. Br J Pharmacol 1995;115:1265-75.
- Woolf CJ, Safieh-Garabedian B, Ma QP, Crilly P, Winter J. Nerve growth factor contributes to the generation of inflammatory sensory hypersensitivity. Neuroscience 1994;62:327-31.
- Cunningham ET, De Souza EB. Interleukin-1 receptors in the brain and endocrine tissues. Immunol Today 1993;14:171-6.
- Cutolo M. IL-1Rα: its role in rheumatoid arthritis. Reumatismo 2004;56:41-5.
- Wolf G, Yirmiya R, Goshen I, Iverfeldt K, Holmlund L, Takeda K, et al. Impairment of interleukin-1 (IL-1) signaling reduces basal pain sensitivity in mice: genetic, pharmacological and developmental aspects. Pain 2003;104:471-80.
- Watkins LR, Maier SF, Goehler LE. Immune activation: the role of pro-inflammatory cytokines in inflammation, illness responses and pathological pain states. Pain 1995;63:289-302.
- Kiefer R, Kreutzberg GW. Gamma interferon-like immunoreactivity in the rat nervous system. Neuroscience 1990;37:725-34.
- Xian CJ, Zhou XF. Neuronal-glial differential expression of TGF-alpha and its receptor in the dorsal root ganglia in response to sciatic nerve lesion. Exp Neurol 1999;157:317-26.
- Freidin M, Bennett MV, Kessler JA. Cultured sympathetic neurons synthesize and release the cytokine interleukin 1 beta. Proc Natl Acad Sci USA 1992;89:10440-3.
- Kreicbergs A, Ahmed M, Ehrnberg A, Schultzberg M, Svenson SB, Bjurholm A. Interleukin-1 immunoreactive nerves in heterotopic bone induced by DBM. Bone 1995;17:341-5.
- Morioka N, Takeda K, Kumagai K, Hanada T, Ikoma K, Hide I, et al. Interleukin-1beta-induced substance P release from rat cultured primary afferent neurons driven by two phospholipase A2 enzymes: secretory type IIA and cytosolic type IV. J Neurochem 2002;80:989-97.
- Copray JCVM, Mantingh I, Brouwer N, Biber K, Kust BM, Liem RSB, et al. Expression of interleukin-1 beta in rat dorsal root ganglia. J Neuroimmunology 2001;118:203-11.
- Butler SH, Godefroy F, Besson JM, Weil-Fugazza J. A limited arthritic model for chronic pain studies in the rat. Pain 1992;48:73-81.
- Liu HX, Xu W, Luo F, Wang JS, Han JS. A limited arthritic model for the chronic pain in rats. Chin J Pain Med 1996;2:223-8.
- Butler SH, Weil-Fugazza J, Godefroy F, Besson JM. Reduction of arthritis and pain behaviour following chronic administration of amitryptiline or impipramine in rats with adjuvant-induced arthritis. Pain 1985;23:159-75.
- Liu HX, Xiong L, Luo F, Han JS. Improved scoring method for arthritic flexion pain test in Freund’s adjuvant monoarthiritic model in the rat. Chin J Pain Med 1998;4:173-8.
- Kai-Kai MA. Cytochemistry of the trigeminal and dorsal root ganglia and spinal cord of the rat. Comp Biochem Physiol 1989;93A:183-93.
- Oka T, Oka K, Hosoi M, Hori T. Inhibition of peripheral interleukin-1β-induced hyperalgesia by the intracerebroventricular administration of diclofenac and α-melanocyte-stimulating hormone. Brain Res 1996;736:237-42.
- Perretti M, Ahluwalia A, Flower RJ, Manzini S. Endogenous tachykinins play a role in IL-1-induced neutrophil accumulation: involvement of NK-1 receptors. Immunology 1993;80:73-7.
- Fukuoka H, Kawatani M, Hisamitsu T, Takeshige C. Cutaneous hyperalgesia induced by peripheral injection of interleukin-1β in the rat. Brain Res 1994;657:133-40.
- Malcangio M, Bowery NG, Flower RJ, Perretti M. Effect of interleukin-1β on the release of substance P from rat isolated spinal cord. Eur J Pharmacol 1996;299:113-8.
- Obreja O, Rathee PK, Lips KS, Distler C, Kress M. IL-1β potentiates heat-activated currents in rat sensory neurons: involvement of IL-1RI, tyrosine kinase, and protein kinase C. FASEB J 2002;16:1497-503.
- Igwe OJ. c-Src kinase activation regulates preprotachykinin gene expression and substance P secretion in rat sensory ganglia. Eur J Neurosci 2003;18:1719-30.
- Horie H, Sakai I, Akahori Y, Kadoya T. IL-1 beta enhances neurite regeneration from transected-nerve terminals of adult rat DRG. Neuroreport 1997;8:1955-9.
- Zhou XF, Deng YS, Chie E, Xue Q, Zhong JH, McLachlan EM, et al. Satellite-cell-derived nerve growth factor and neurotrophin-3 are involved in noradrenergic sprouting in the dorsal root ganglia following peripheral nerve injury in the rat. Eur J Neurosci 1999;11:1711-22.