Visual recognition memory is related to basic expression level of NMDA receptor NR1/NR2B subtype in hippocampus and striatum of rats1
Introduction
N-Methyl-D-aspartate (NMDA) receptors, the major ionotropic glutamate receptors in the central nervous system, play key roles in excitatory synaptic transmission and plasticity, and are involved in many physiological and pathological processes[1,2]. NMDA receptors are mainly composed of NR1 subunit and NR2A, NR2B, NR2C, or NR2D subunit, respectively, and the different subunit combinations form different NMDA receptor subtypes with distinct functional properties[3,4].
It has been demonstrated that NMDA receptors are involved in long-term synaptic plasticity and certain forms of learning and memory[5–8]. For example, numerous data indicate that NMDA receptor activity and NMDA receptor-dependent synaptic plasticity in the hippocampus are crucial for spatial recognition memory[7,8]. However, there have been a few reports about the role of NMDA receptors in visual recognition memory[9–12], and there are some discrepant or even contradictory results in the visual recognition memory with regard to different doses of NMDA receptors antagonist, and animal species. Furthermore, most of those findings were mainly obtained from animals with specific brain region injuries, pharmacological manipulated animals or transgenic mice with one of NMDA receptor subunits abnormally expressed. Little is known whether the basic expression levels of NMDA receptors is related to different visual recognition memory in normal adult rats.
Therefore, the objective of our studies was to examine the relationship between the basic expression levels of NR1 and NR2B subunits in six specific regions (cortex, hippocampus, striatum, amygdala, diencephalons, and olfactory bulb) and visual recognition memory in rats.
Materials and methods
Animals All experiments were carried out in accordance with the National Institutes of Health Guide for the Care and Use of Laboratory Animal. The animals used in this study were 6-week old male Sprague-Dawley (SD) rats, weighing 175–190 g (Grade II, Certificate N
Novel object recognition task Rat was individually habituated to an open-field box (50 cm×50 cm×30 cm high) for 3 d. During training sessions, two novel objects were placed into the open field and the animal was allowed to explore for 5 min. The time spent for exploring each object was recorded. During retention tests, the animal was placed back into the same box after a 24-h delay, in which one of the objects used during training was replaced by a novel object, and allowed to explore freely for 5 min. Behaviour was considered exploratory when an animal was touching or directed towards the object at a distance ≤2 cm with its nose; while behaviour was not considered exploratory when an animal was turning around or sitting on the object. To avoid interference effect of the olfactory cue due to scent traces left, after each experiment, both the objects and the arena were scraped by 10% ethanol. The preference index, a ratio of the amount of time spent exploring any one of the two objects (training session) or the novel one (retention session) over the total time spent exploring both objects, was used to measure recognition memory. All 40 male SD rats were ranked by the preference index. From these, eight superior and eight inferior rats were used as high visual recognition memory group and low visual recognition memory group, respectively, in the present study.
Preparation of tissue The rats were killed by decapitation and the brains were quickly removed. The brains were dissected on ice into six specific regions (cortex,hippo-campus, striatum, amygdala, diencephalon, and olfactory bulb), and homogenized twice in 100 volumes (100×wet weight) of ice-cold 10 mmol/L Tris-HCl (pH 7.4) containing 320 mmol/L sucrose with a Tissuemizer (Heidolth DIA×900) at speed 5 for 10 s with a 20 s interval between bursts. The tissue homogenate was centrifuged at 700×g for 10 min at 4 ºC. The supernatant was collected and centrifuged at 37 000×g for 40 min at 4 ºC. This high-speed pellet was resuspended in 10 mmol/L Tris-HCl (pH 7.4). Protein concentrations were determined using Folin phenol reagent with bovine serum albumin as a standard.
Semiquantitative Western blot Sodium dodecyl sulfate-polyacrylamide gel electrophoresis (SDS-PAGE) and transfer of proteins to nitrocellulose membranes were performed according to conventional methods. The denatured membrane proteins were separated on 7.5% polyacrylamide gels and transferred to nitrocellulose membranes in transfer buffer (Tris 25 mmol/L, glycine 192 mmol/L, 20% methanol and 0.05% SDS, pH 8.3). The membranes were incubated with a blocking buffer of 5% non-fat dry milk in TBST (Tris-HCl 20 mmol/L, NaCl 140 mmol/L, 0.1% Tween-20, pH 7.4) at room temperature for 1 h. The membranes were then incubated with anti-NR1, and anti-NR2B antibodies (1 g/L) in blocking buffer overnight at 4 ºC. After several washes with TBST, the membranes were incubated with horseradish peroxidase-conjugated secondary antibody in blocking buffer for 1 h at room temperature. Following washes for 20 min with four intermediate changes with TBST, proteins were visualized with enhanced chemiluminescence. The resulting film was scanned and analyzed using a laser densitometer and GelScan XL software (Pharmacia LKB). In order to make different gel samples evaluable, every gel was run with three lanes of the same cortex proteins as a standard[13,14].
Statistical analysis All results were expressed as mean± SD. Differences were analyzed by group comparisons t-test with Mann-Whitney Test by SPSS 11.0 software. P<0.05 was considered statistically significant.
Results
Novel object recognition task The preference indexes for the high visual recognition memory group and the low visual recognition memory group were summarized in Figure 1. During the training sessions, no significant difference was found in the exploratory preference between the two groups (0.43±0.12 vs 0.49±0.11, P>0.05). During the retention sessions, the exploratory preference of the high visual recognition memory group was significantly higher than that of the low one (P<0.01).
Relationship between the basic expression level of NR1 subunit and visual recognition memory in rats The protein level of NR1 subunit in the hippocampus in the high visual recognition memory group was 35.9% higher than that in the low group (1.89±0.18 vs 1.39±0.39, P<0.01). However, no significant difference was found in the protein levels of NR1 subunit in the cortex, striatum, amygdala, diencephalon and olfactory bulb of rats between the two groups (Figure 2).
Relationship between basic expression level of NR2B subunit and visual recognition memory in rats As shown in Figure 3, the protein level of NR2B subunit in the hippocampus in the high visual recognition memory group was 53.3% higher than that in the low group (2.04±0.22 vs 1.33±0.57, P<0.05). In addition, the NR2B level in the high one was 25.0% higher than that in the low group in the striatum (P<0.05). While, no significant difference was found in the protein levels of NR2B subunit in other brain regions between the two groups (P>0.05).
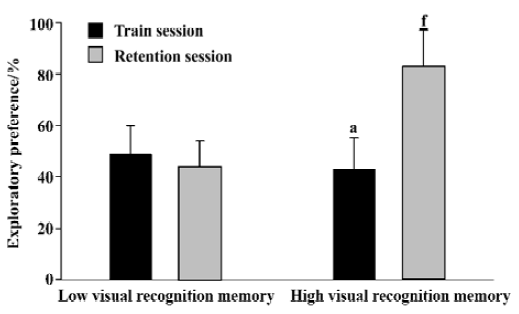
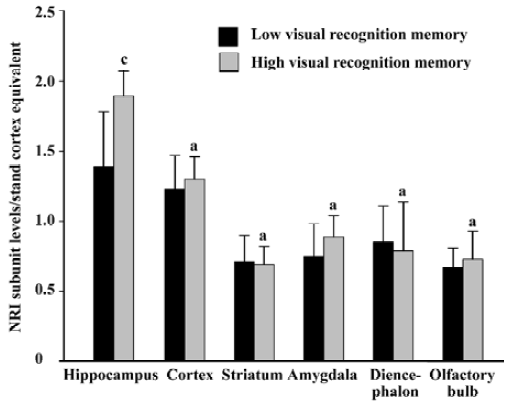
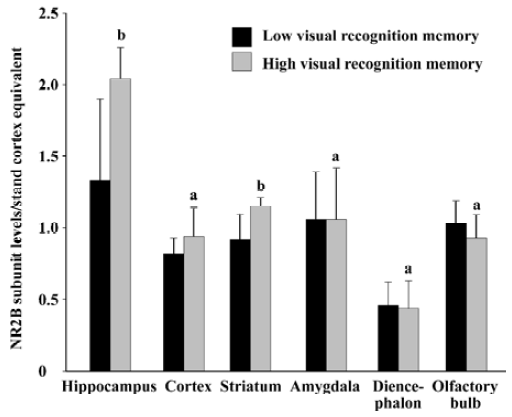
Discussion
In the present study, we observed that both NR1 and NR2B subunits in the hippocampus in the high visual recognition memory group were remarkably higher than those in the low group. So the NMDA receptor NR1/NR2B subtype is higher in the high visual recognition memory group, although it is unclear whether there is any difference in the basic expression levels of the other subtypes of NMDA receptors. The result is consistent with the previous studies, which found that the transgenic mice overexpressing NR2B subunit in the forebrains exhibited an enhancement of novel -object-recognition memory[10], and the CA1-specific NR1 subunit knockout mice exhibited impairment in object recognition[15]. Therefore, our data provided more evidence in vivo to support the concept that NMDA receptors in the hippocampus are involved in visual recognition memory.
Previous studies showed that lesions to the perirhinal, postrhinal cortex and associated structures induced deficits in the ability to recognize single objects[16,17], and that NMDA receptor blockade in the perirhinal cortex impaired object discrimination in rats[18]. It seems that NMDA receptors in the cortex are related to visual recognition memory. However, in our study, no change in the basic expression levels of NR1 and NR2B subunits in the cortex was found between the groups with different visual recognition memory. Quinlan et al also reported that the NR2B subunit level in the visual cortex remained unchanged by visual experience[19]. The explanation for these converse results might be that the cortical coding of object recognition decays quickly and is not sufficient for maintaining information about objects across longer retention intervals (eg 24 h)[20]. It is also possible that the NMDA receptor NR2B subunit in some sub-regions of the cortex is related to visual memory. So our results at least suggest that the basic expression levels of NR1 and NR2B subunits in the cortex correlated less with visual recognition memory when a 24 h retention interval was imposed.
The striatum is a brain region involved in procedural memory and diverse forms of implicit memory[21,22]. It is well known that glutamatergic input from the cortex to the striatum is critical for striatal learning[23] and NR2B subunit in the striatum is correlated with water maze performance in the inferior group of the aged rats[24]. Our results here provided more evidences that the basic expression level of NR2B subunit in the striatum was also correlated with visual recognition memory.
The novel-object-recognition task was used in this study, which is based on the spontaneous preference that animals display for novel objects. This task is considered to need only “familiarity judgment” as to whether the objects presented in the choice phase are novel or familiar to the animals[25]. The advantage of this method is that performance does not depend on the retention of a rule, and it is not influenced by changes in response to reward, which can provide a relatively pure measure of “working memory”[26].
In conclusion, the main finding of the present study is that the basic expression level of the NMDA receptor NR1/NR2B subtype in the hippocampus and striatum is related to visual recognition memory.
Footnote
Project supported by Key National Basic Research Program of China (N
References
- Constantine-Paton M, Cline HT, Debski E. Patterned activity, synaptic convergence, and the NMDA receptor in developing visual pathways. Annu Rev Neurosci 1990;13:129-54.
- Mori H, Mishina M. Structure and function of the NMDA receptor channel. Neuropharmacology 1995;34:1219-37.
- Nakanishi S. Molecular diversity of glutamate receptors and implications for brain function. Science 1992;258:597-603.
- Monyer H, Burnashev N, Laurie DJ, Sakmann B, Seeburg PH. Developmental and regional expression in the rat brain and functional properties of four NMDA receptors. Neuron 1994;12:529-40.
- Bliss TV, Collingridge GL. A synaptic model of memory: long-term potentiation in the hippocampus. Nature 1993;361:31-9.
- Bear MF, Malenka RC. Synaptic plasticity: LTP and LTD. Curr Opin Neurobiol 1994;4:389-99.
- Tsien JZ, Huerta PT, Tonegawa S. The essential role of hippocampal CA1 NMDA receptor-dependent synaptic plasticity in spatial memory. Cell 1996;87:1327-38.
- Nakazawa K, McHugh TJ, Wilson MA, Tonegawa S. NMDA receptors, place cells and hippocampal spatial memory. Nat Rev Neurosci 2004;5:361-72.
- Baker KB, Kim JJ. Effects of stress and hippocampal NMDA receptor antagonism on recognition memory in rats. Learn Mem 2002;9:58-65.
- Tang YP, Shimizu E, Dube GR, Rampon C, Kerchner GA, Zhuo M, et al. Genetic enhancement of learning and memory in mice. Nature 1999;401:63-9.
- Paule MG, Fogle CM, Allen R, Hammond TG, Popke EJ. Chronic exposure to NMDA receptor and sodium channel blockers during development in monkeys and rats: long-term effects on cognitive function. Ann N Y Acad Sci 2003;993:116-22.
- Puma C, Bizot JC. Intraseptal infusions of a low dose of AP5, a NMDA receptor antagonist, improves memory in an object recognition task in rats. Neurosci Lett 1998;248:183-6.
- Zhu LJ, Chen Z, Zhang LS, Xu SJ, Xu AJ, Luo JH. Spatiotemporal changes of the N-methyl-D-aspartate receptor subunit levels in rats with pentylenetetrazole-induced seizures. Neurosci Lett 2004;356:53-6.
- Luo J, Wang Y, Yasuda RP, Dunah AW, Wolfe BB. The majority of N-methyl-D-aspartatereceptor complexes in adult rat cerebral cortex contain at least three different subunits (NR1/NR2A/NR2B). Mol Pharmacol 1997;51:79-86.
- Rampon C, Tang YP, Goodhouse J, Shimizu E, Kyin M, Tsien JZ. Enrichment induces structural changes and recovery from nonspatial memory deficits in CA1 NMDAR1 knockout mice. Nat Neurosci 2000;3:238-44.
- Bussey TJ, Muir JL, Aggleton JP. Functionally dissociating aspects of event memory: the effects of combined perirhinal and postrhinal cortex lesions on object and place memory in the rat. J Neurosci 1999;19:495-502.
- Murray EA, Mishkin M. Object recognition and location memory in monkeys with excitotoxic lesions of the amygdala and hippocampus. J Neurosci 1998;18:6568-82.
- Abe H, Iwasaki T. NMDA and muscarinic blockade in the perirhinal cortex impairs object discrimination in rats. Neuroreport 2001;12:3375-9.
- Quinlan EM, Philpot BD, Huganir RL, Bear MF. Rapid, experience-dependent expression of synaptic NMDA receptors in visual cortex in vivo. Nat Neurosci 1999;2:352-7.
- Hammond RS, Tull LE, Stackman RW. On the delay-dependent involvement of the hippocampus in object recognition memory. Neurobiol Learn Mem 2004;82:26-34.
- Kelley AE, Andrzejewski ME, Baldwin AE, Hernandez PJ, Pratt WE. Glutamate-mediated plasticity in corticostriatal networks: role in adaptive motor learning. Ann N Y Acad Sci 2003;1003:159-68.
- Sgambato V, Minassian R, Nairn AC, Hyman SE. Regulation of ania-6 splice variants by distinct signaling pathways in striatal neurons. J Neurochem 2003;86:153-64.
- Da Cunha C, Angelucci ME, Canteras NS, Wonnacott S, Takahashi RN. The lesion of the rat substantia nigra pars compacta dopaminergic neurons as a model for Parkinson’s disease memory disabilities. Cell Mol Neurobiol 2002;22:227-37.
- Schulz D, Sergeeva OA, Ianovskii E, Luhmann HJ, Haas HL, Huston JP. Behavioural parameters in aged rats are related to LTP and gene expression of ChAT and NMDA-NR2 subunits in the striatum. Eur J Neurosci 2004;19:1373-83.
- Ennaceur A, Delacour J. A new one-trial test for neurobiological studies of memory in rats. 1: behavioral data. Behav Brain Res 1988;31:47-59.
- Ennaceur A, Neave N, Aggleton JP. Spontaneous object recognition and object location memory in rats: the effects of lesions in the cingulate cortices, the medial prefrontal cortex, the cingulum bundle and the fornix. Exp Brain Res 1997;113:509-19.