Inhibiting wear particles-induced osteolysis with doxycycline
Introduction
Total joint replacement is a common operation performed to treat severe arthritis affecting loading bearing joints for its effectiveness in reducing pain and improving joint function. However, wear particles resulting from the degradation of joint arthroplasty components have significant adverse influence on the survival of joint arthroplasty[1]. Wear particles induced by osteolysis was considered one of the principal contributors to implant aseptic loosening and consequent joint revision operation after total joint arthroplasty[2]. Wear debris stimulate peri-prosthetic inflammation, activate phagocytic cells to secrete inflammatory cytokines at a high level, such as interleukin (IL)-1, IL-6, and TNF-α, which are osteoclastogenic, stimulate osteoblasts and stromal cells expressing high levels of the receptor activator of NF-κB ligand (RANKL), and decrease the ratio between osteoprotegrin and RANKL[3–5]. Wear particles were observed to cause adverse biological reactions which seem to occur in the following order: inflammation, increased osteoclastogenesis, and subsequent osteoclastic bone resorption[6].
Increased osteoclastogenesis and activated osteoclast function are known to be central to wear particles induced by peri-prosthetic osteolysis and implant aseptic loosening[7]. Cytokines, such as TNF-α and IL-1, play important roles in wear particles induced by osteolysis according to knowledge obtained from a mouse model of osteolysis[8,9]. Because the increased osteoclastogenesis and osteoclast activation is the center of wear particles induced by osteolysis, inhibiting osteoclastogenesis and osteoclast function would be important in treating or preventing aseptic loosening after total joint replacement.
Tetracyclines and their non-antimicrobial, chemically-modified analogues have properties that appear to modulate host response by inhibiting the activity of the matrix metalloproteinases that cause collagen destruction. They also inhibit osteoclast function and stimulate osteoblastic bone formation[10]. Doxycycline (DOX) belongs to chemically-modified tetracycline antibiotics. Recently, it was studied for its effect upon bone-related diseases. Previous in vitro research showed that DOX was able to inhibit in vitro osteoclastogenesis and cause apoptosis of mature osteoclasts; inhibiting osteoclasts caused bone resorption[11,12]. These effects extend beyond the mere inhibition of matrix metallo-proteinases (MMP).
In the present study, we utilized in vitro culture of mouse bone marrow monocytes and rabbit mature osteoclasts to study DOX on osteoclastogenesis and mature osteoclast fate. We employed an in vivo mouse calvarial osteolysis model to study whether DOX was able to inhibit wear particles induced by inflammatory osteolysis[13,14]. In doing so, we hoped to provide a foundation for advancing the use of DOX in the treatment or prevention peri-prosthetic osteolysis and aseptic loosening accruing after total joint replace-ment.
Materials and methods
Inhibiting in vitro osteoclastogenesis with DOX Osteoclasts were generated from osteoclast progenitors by culture of primary mouse, non-adherent bone marrow monocytes of 6–8-week-old male C57BL/J6 mice (Shanghai SLAC Laboratory Animal Co, Shanghai, China)[15]. Mouse bone marrow cells were collected from femoral and tibial shafts by flushing with 8 mL cold αMEM (Gibco, Invitrogen, Grand Island, NY, USA) containing 10% FCS (Hyclone, Tauranga, New Zealand), 1% penicillin and streptomycin (Gibco, Invitrogen, Grand Island, NY, USA). Cell suspensions were passed through an 18 g needle in αMEM to disperse the clumps. Bone marrow-adherent cells were removed by incubating at 37 °C with 5% CO2 in a humidified incubator for 24 h in the 10 cm Falcon culture dish. The bone marrow monocytes were isolated from the non-adherent cells in the supernatant by using gradient centrifugal isolation in Ficoll (The Second Chemical Reagent Factory of Shanghai, Shanghai, China). The bone marrow monocytes were then cultured in the continuous presence of both macrophage colony-stimulating factor (M-CSF, 30 ng/mL; Research Diagnostics, Flanders, NJ, USA) and recombinant soluble RANKL (100 ng/mL; Research Diagnostics, USA) for 7 d. The culture medium was changed with fresh M-CSF and RANKL every 48 h. DOX was added to the culture medium at a final concentration of 5, 10, 15 or 20 µg/mL, respectively. Seven days later, the cells were washed with phosphate-buffer solution (PBS) and fixed in 2.5% glutaraldehyde for 5 min at room temperature. The commercial leukocyte acid phosphatase kit was then used for tartrate-resistant acid phosphatase (TRAP) staining (Shanghai Rainbow Medical Reagent Research, Shanghai, China) and TRAP(+) cells were counted[11,12].
Inhibiting mature osteoclast survival and function with DOX Mature osteoclasts were isolated from 7 d old New Zealand white rabbits (Shanghai SLAC Laboratory Animal, China) as previously reported[16]. Briefly, after cervical dislocation, the rabbits were sterilized in 75% ethanol for 5 min. Long bones were taken out and soft tissues attached to bones were removed. The bones were minced in αMEM containing 10% FCS plus 1% penicillin and streptomycin. The cells were dissociated from the bone fragments by vortexing for 30 s and the fragments were allowed to sediment for 1 min. The cells in the supernatant were collected and used as unfractionated bone cells. 5×106 bone marrow cells were seeded into 1 well of the 24-well plate and incubated at 37 °C with 5% CO2 for 3 d. For the bone resorption assay, 5×106 bone marrow cells were seeded onto 5×5 mm bovine bone slices. DOX was added to the culture medium at a final concentration of 5, 10, 15, or 20 µg/mL, respectively. Three days later, the cells were washed with PBS and fixed in 2.5% glutaraldehyde for 5 min at room temperature. A commercial leukocyte acid phosphatase kit (Shanghai Rainbow Medical Reagent Research, China) was used for TRAP staining. TRAP(+) cells with more than 3 nuclei were counted [11,12]. After fixation, the cells on the bone slices were washed off with 25% ammonium hydroxide and sonicated 3 times for 5 min. The bone slices were then dehydrated through an ethanol gradient and stained with 1% toluidine blue and Mayer-hematoxylin. Positively stained areas were counted as bone resorption pits and the resorption area was calculated with Image Pro-Plus 5.0 (Media Cybernetics, Bethesda, MD, USA).
Inhibiting wear particles induced by in vivo osteolysis with DOX In accordance with the original model of wear particulate induced by calvarial osteolysis[14], polymethyl methacrylate (PMMA) or ultra-high molecular weight polyethylene (UHMWPE) particles were applied to 8-week-old, healthy C57BL/J6 male mice (Shanghai SLAC Laboratory Animal Co, China) according to the official guidelines of the Shanghai Jiaotong University School of Medicine (Shanghai, China). The weight of the mice was 22±3 g. The animals were randomly classified into 7 groups as follows with 6 mice in each group: (i) control group, where the mice underwent sham operation; (ii) PMMA group, where the mice received 30 mg PMMA particle implantation; (iii) PMMA+ 2DOX group, where the mice received 30 mg PMMA particles plus 2 mg/kg DOX intraperitoneal injection every day from d 1 to 7; (iv) PMMA+10DOX group, where the mice received 30mg PMMA particles plus 10 mg/kg DOX intraperitoneal injection every day from d 1 to 7; (v) UHMWPE group, where the mice received 30 mg UHMWPE particle implantation; (vi) UHMWPE+2DOX group, where the mice received 30 mg UHMWPE particles plus 2 mg/kg DOX intraperitoneal injection every day from d 1 to 7; and (vii) the UHMWPE+10DOX group, where the mice received 30 mg UHMWPE particles plus 10 mg/kg DOX intraperitoneal injection every day from d 1 to 7. The animals had free access to water and food and were kept in 12 h on/12 h off specific pathogen-free animal room.
The PMMA particles (provided by Dr Yong from Technical Institute of Physics and Chemistry of China Academy of Sciences, Beijing, China) were smaller than 10 µm. The UHMWPE particles (a generous gift from Dr John, University of Alabama, Birmingham, AL, USA) had a mean particle diameter of 3.6 µm, with a range of 2.0–23 µm. The particles were washed continuously in absolute ethanol for 48 h in a shaker (Shanghai Centrifuge Institute, Shanghai, China) with a speed of 200 r/min to remove adherent endotoxins according to a previous report[17]. The particles were resuspended in sterile PBS solution at a concentration of 300 mg/mL. The particles’ endotoxin level was lower than 0.1 EU/mL, as determined using a commercial detection kit (Chromogenic end-point TAL with a Diazo coupling kit (Xiamen Houshiji, Fujian, China). The PMMA or UHMWPE particles were stored at 4 °C before use.
The mice were anesthetized with 1% pentobarbital sodium (Shanghai Chemical Reagent Co, Shanghai, China) as an intraperitoneal injection at a dosage of 45 mg/kg. After shaving, the skin was sterilized. An incision was made between the tow external ears. As previously reported, a 1 cm middle sagittal incision was made, the subcutaneous bursa was entered, and about a 1×1 cm2 area was exposed, with care not to disrupt the periostrium[14]. 30 mg PMMA or UHMWPE particles in 100 µL sterile PBS was uniformly spread over the exposed periostrium. The skin was closed with simple disrupted suture to prevent leakage of the particles. In the control group, the animals underwent operation and 100 µL sterile PBS without particles. After the operation, the mice were warmed up, recovered, and sent back to the animal room.
Seven days later, the mice were killed with an overdose of anesthesia and the calvariae were removed with care not to split the sagittal suture. The specimens were freed off soft tissue and the underlying brain was fixed in 4% neutralized paraformaldehyde for 12 h and decalcified in 12.5% EDTA (pH 7.4) at 4 °C for 2 weeks. The decalcification solution was changed every 3 d. Then the specimens were treated in graded ethanol for dehydration and embedded in paraffin. Every specimen was sectioned with a 7 µm thickness on the sagittal plane.
For the middle suture osteolysis area analysis, 5 consecutive sections of each specimen were stained with hematoxylin-eosin (Shanghai Rainbow Medical Reagent Research, China). Photos were taken at a magnification of 10× with use of a Nikon Eclipse 80i microscope (Nikon, Tokyo, Japan) attached to a computer. The middle suture was centered in each image. The non-osseous tissue area adjacent and in continuity with the midline suture was taken as the osteolysis of the calvarial bone, traced by hand, and calculated with Image Pro-Plus 5.0 (Media Cybernetics, USA).
For the osteoclastogenesis analysis, 5 consecutive sections of each sample were stained using a commercial leukocyte acid phosphate staining kit (Shanghai Rainbow Medical Reagent Research, China). After removing the paraffin in xylene, the sections were incubated in working solution for 90 min at 37 °C. After washing with water, the samples were counterstained with 1% methyl green for 5 min at room temperature. The sections were mounted in neutral balsam. Red-stained cells in the middle suture and adjacent non-osseous osteolsysis area were counted as TRAP(+) osteoclasts.
Statistical analysis All results were expressed as mean±SD. The results were analyzed by ANOVA, followed by Student’s t-test to determine significance. P<0.05 was considered significant.
Results
DOX suppressed osteoclastogenesis in vitro Bone marrow osteoclast precursors were induced into mature osteoclast with RANKL and M-CSF added into the culture medium. Many TRAP(+), multinucleated cells (with more than 3 nuclei) formed compared to the control. No RANKL and M-CSF were added into the culture medium (Figure 1). In the osteoclastogenic medium, approximately 94.6±16.8 TRAP(+) osteoclasts formed 7 d later. There were no TRAP(+) cells in the control wells. While DOX, applied at a concentration of 5 µg/mL, significantly inhibited osteoclastogenesis induced by RANKL and M-CSF, it completely abolished osteoclast formation at the concentration of 20 µg/mL (P<0.001; Figures 1 and 2A).
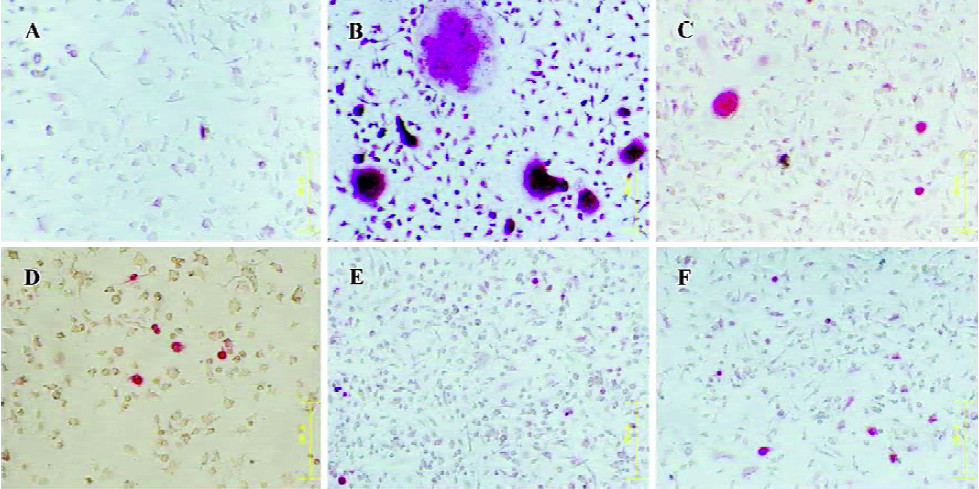
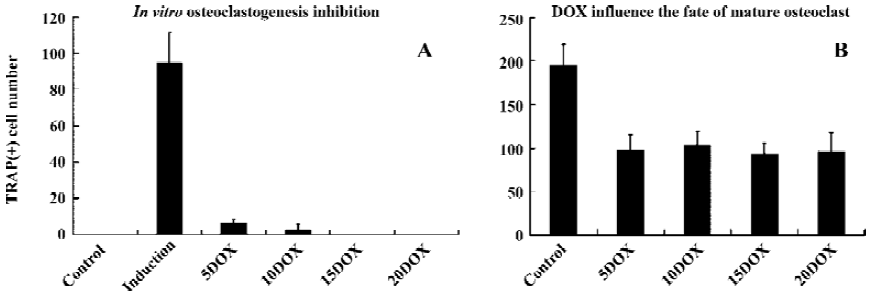
DOX influenced the survive of mature osteoclasts in vitro After 3 d culture of unfractionated, newborn rabbit marrow cell, TRAP staining showed that DOX strongly influenced the survival of mature osteoclasts. However, we did not observe a concentration gradient inhibition phenomenon as previously reported[11]. At a concentration of 5 µg/mL, DOX treatment reduced the survival rate of mature osteoclasts to approximately 50% compared with the control levels, and the effect was significant (P<0.001; Figure 2B). DOX at high concentrations up to 20 µg/mL did not increase the inhibition effect.
DOX suppressed mature osteoclast bone resorption After culture in αMEM on bovine cortical bone slices for 3 days, the bone resorption pits were counted and the resorption area was measured. Osteoclast degraded bone matrix and bone resorption appeared as the formation of many resorption lacunae after staining with toluidine blue and Mayer-hematoxylin (Figure 3C). The resorption pit number was about 150.3±4.5 and the treatment of DOX, at a concentration of 5 µg/mL, significantly reduced the resporption lacunae number to 54.6±24.7 (P<0.001; Figure 3A). DOX at high concentrations of up to 20 µg/mL further reduced the number of resorption lacunae to 14±9 (P<0.001; Figure 3A). The resorption area measurement also showed that DOX significantly reduced the resorption area on cortical slices (P<0.001; Figure 3B). In the control, the resorption was (34.7±4.8)×106 µm2. After adding DOX to the culture medium with a final concentration of 5 µg/mL, the resorption area was reduced to (9.2±4.4)×106 µm2. DOX, at high concentrations of up to 20 µg/mL, further reduced the resorption area to (3.7±2.3)×106 µm2.
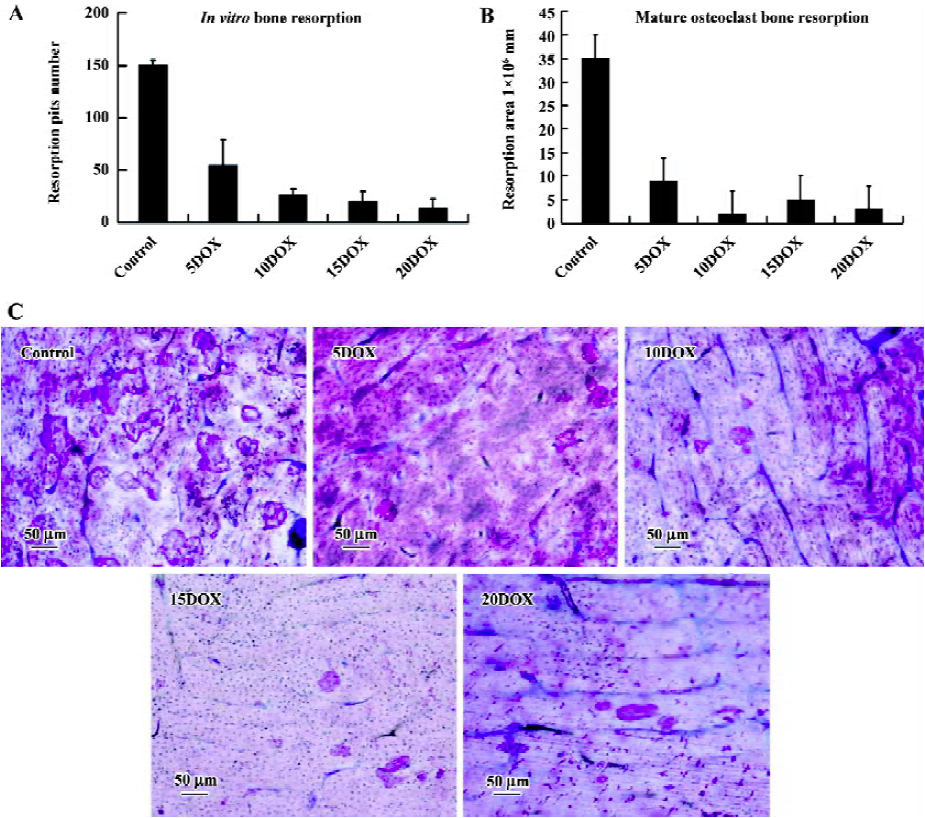
DOX inhibited in vivo osteolysis and osteoclasto-genesis caused by wear particles
Sagittal suture resorption area No obvious resorption was observed in the sagittal suture in group i (Figure 4). The suture and adjacent non-osseous area increased in group c and v in the animal receiving PMMA or UHMWPE particle implantations (Figure 4). In the DOX-treated groups, bone destruction and resorption were inhibited. The sagittal suture resorption area in control group was 0.068±0.012 mm2. The sagittal suture resorption area in PMMA group was 0.335±0.130 mm2, significantly higher than that of the control group (P<0.01; Figure 5A). In PMMA+2DOX group, the mean sagittal suture area was 0.077±0.023 mm2, and in PMMA+10DOX group, the area was 0.079±0.029 mm2. PMMA+2DOX and PMMA+10DOX group had an obviously smaller sagittal suture area than PMMA group (P<0.001 and P<0.001; Figure 5A). In UHMWPE group, the resorption area was 0.408±0.099 mm2, much greater than that of the control group (P<0.001). The suture area in group UHMWPE+ 2DOX and UHMWPE+10DOX were 0.110±0.021 mm2 and 0.084±0.017 mm2, significantly smaller than that of UHMWPE group (P<0.001 and P<0.001). PMMA or UHMWPE particles were seen to induce obvious inflammatory osteolysis. Thus, DOX treatment effectively inhibited inflammatory osteolysis induced by wear particles.
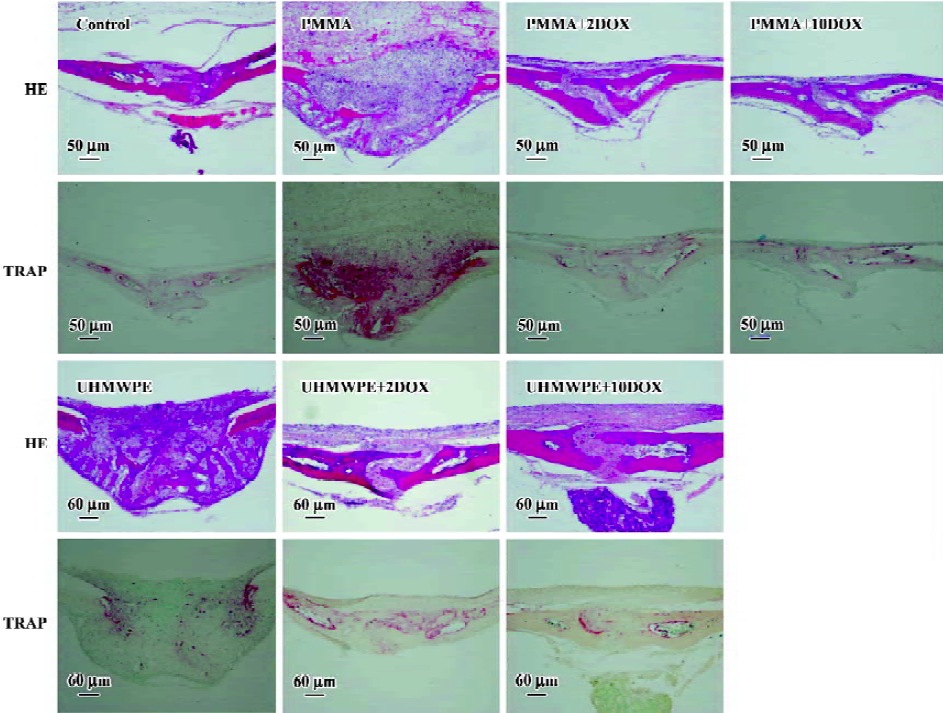
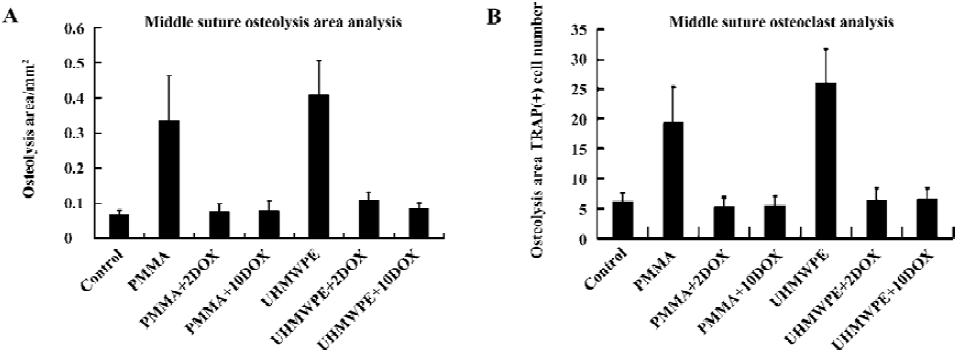
Osteoclast number The osteoclast number within the experimental areas in control group was 6.2±1.4. In PMMA group, the number rose to 19.3±5.9. In PMMA+2DOX group the TRAP(+) number was 5.3±1.5, while in PMMA+10DOX group it was 5.4±1.6. In UHMWPE group, the number was 26.1±5.1, while in UHMWPE+2DOX and UHMWPE+10DOX group it was 6.5±1.9 and 6.6±1.8, respectively (Figure 5B). There was a significant difference in the osteoclast number between the control and PMMA or UHMWPE particle implantation groups (P<0.001, P<0.001). DOX treatment strongly suppressed osteoclasto-genesis induced by PMMA (P<0.001and P<0.001) or UHMWPE (P<0.001and P<0.001) particles.
Discussion
Total joint replacement is a major advance in the treatment of end-stage arthritis affecting main load-bearing joints due to its effectiveness in improving joint function and alleviating disabling pain[18]. Aseptic loosening due to peri-prosthetic loosening is the major cause affecting the long-term result of total joint replacement and the major reason for complex joint revision arthroplasty operations[19,20]. Small wear particles resulting from implant components fretting stimulate a cascade of adverse biological reactions and osteolysis, which finally results in arthroplasty aseptic loosening.
Osteoclast is the center and final pathway of wear particles induced by inflammatory osteolysis. So inhibiting osteoclastogenesis and inflammation is an important step in treating wear particles induced by osteolysis. Many efforts have been made to treat or prevent wear particle osteolysis[6,13,21–26]. Until now, only etanercept, a TNFα receptor antagonist and bisphosphonate, has been applied in clinical situations after total joint arthroplasty[26–28]. The results have been unsatisfactory and no treatment consensus has been reached. A great deal remains to be done.
Tetracyclines and their chemically-modified analogues can inhibit the activity of MMP, which are considered to be central for the inhibition of bone destruction[29]. Recently, DOX was found to have some effect beyond inhibiting MMP activities; it can inhibit osteoclastogenesis, cause apoptosis of mature osteoclast, and inhibit the mature osteoclast bone resorption function[11,12]. Previous in vitro research has shown that DOX was able to inhibit peri-prosthetic interface cells caused bone resorption through the inhibition the activity of MMP in in vitro mouse calvarial organ culture system[30]. However, the relationship between bone destruction and MMP activity inhibition was not investigated. In this in vitro mouse calvarial organ culture system, osteolysis was attributed to increased osteoclastic bone resorption[7]. In the present work, we used in vitro and in vivo experiments to show that DOX can inhibit wear particles induced osteoclastogenesis and osteolysis.
When culturing mouse bone marrow monocytes with the stimulation of RANKL and M-CSF in vitro, many TRAP(+), multinuclear osteoclasts formed[15]. DOX effectively inhibited RANKL and M-CSF induced by osteoclastogenesis and reduced TRAP(+), multinuclear osteoclasts. High concentrations not only abolishes TRAP(+) osteoclast formation, but inhibited TRAP(+) mono-nuclear cell formation. This evidence strongly supports the conclusion that DOX can effectively inhibit osteoclastogenesis. This is important for treating wear particles induced by osteolysis with DOX, given that increased osgteoclastogenesis is central to particle inflammation reaction, as cytokines released by macrophages can support the survival and activate the function of mature osteoclasts[31,32]. So reducing the survival and inhibiting the function of mature osteoclasts can also inhibit wear particles induced by osteolysis.
Mature osteoclasts were isolated from newborn rabbits. After culturing unfractionated bone marrow cells for 3 d in vitro, TRAP staining showed that there were many mature osteoclasts. DOX affected the fate of the mature osteoclasts. After adding DOX to the culture medium, the number of TRAP(+), multinuclear cells decreased significantly. Seeding unfractionated newborn rabbit bone marrow cells on bovine cortical bone slices, many resorption pits formed as shown by toluidine blue-Mayer hematoxylin staining. The resorption lacuna was big and deeply stained. DOX significantly inhibited mature osteoclasts caused by bone resorp-tion. It also reduced the number of resorption pits and the total resorption area.
In vitro results confirmed that DOX treatment not only decreased osteoclastogenesis, but also reduced the fate of mature osteoclasts and their function. This was consistent with previous reports[11,12]. However, the observed effect on mature osteoclasts’ fate was not according to previous reports; we did not observe concentration gradient inhibition phenomena.
In vivo wear particles induced mouse calvarial osteolysis model is convenient for studying the mechanism of wear particles induced by osteolysis and for studying the effect of drugs aimed at interfering this process[13,14]. When PMMA or UHMWPE particles were implanted in the calvariae of C57/BL6 mice, they caused significant middle suture bone resorption and inflammatory reactions as previously reported. The middle suture and adjacent non-osseous osteolysis area increased and the TRAP(+) osteoclast number increased. Middle suture pathology analysis results showed that DOX treatment protected calvarial bones from wear particles induced by inflammatory osteolysis. For the first time, in vivo data showed that DOX can effectively inhibit this process.
The calvarium is a flat bone and no prosthesis is present; it can not truly reflect the relationship of a prosthesis and medullar canal. Therefore, in future studies, large animals with a true prosthesis in the medullary canal are needed to evaluate the effect of DOX in treating wear particles induced by osteolysis. Further work must still be done to evaluate the long-time toxic effect of DOX, because treating wear particles induced by osteolysis and aseptic loosening needs requires long-term use of medication. Although there are shortcomings of the mouse calvarial osteolysis model, it remains an inexpensive and convenient model for studying the mechanism of wear particles induced by osteolysis and for evaluating drugs aimed at intervening in this process.
In conclusion, DOX has been shown to effectively inhibit wear particles induced by osteolysis via the inhibition of osteoclastogenesis and the reduction of mature osteoclast survival and function. The findings of the present study suggest that DOX might successfully applied in the treatment of osteolysis and aseptic loosening occurring after total joint arthroplasty.
Acknowledgements
We would like to thank Dr Yong HUANG from the Technical Institute of Physics and Chemistry of the China Academy of Sciences (Beijing, China) and Dr John CUCKLER, from the University of Alabama, for providing us with PMMA and UHMWPE particles. We also thanked Dr Qi-ming FANG for his kind help for the animal operations, and Ms Xi-feng DONG for her kind help for the pathology processing.
References
- Harris WH. Wear and periprosthetic osteolysis: the problem. Clin Orthop 2001.66-70.
- Bauer TW. Particles and periimplant bone resorption. Clin Orthop 2002.138-43.
- Baumann B, Rader CP, Seufert J, Noth U, Rolf O, Eulert J, et al. Effects of polyethylene and TiAlV wear particles on expression of RANK, RANKL and OPG mRNA. Acta Orthop 2004;75:295-302.
- Koreny T, Tunyogi-Csapo M, Gal I, Vermes C, Jacobs JJ, Glant TT. The role of fibroblasts and fibroblast-derived factors in periprosthetic osteolysis. Arthritis Rheum 2006;54:3221-32.
- Wooley PH, Morren R, Andary J, Sud S, Yang SY, Mayton L, et al. Inflammatory responses to orthopaedic biomaterials in the murine air pouch. Biomaterials 2002;23:517-26.
- Yang SY, Wu B, Mayton L, Mukherjee P, Robbins PD, Evans CH, et al. Protective effects of IL-1Ra or vIL-10 gene transfer on a murine model of wear debris-induced osteolysis. Gene Ther 2004;115:483-91.
- Jacobs JJ, Roebuck KA, Archibeck M, Hallab NJ, Glant TT. Osteolysis: basic science. Clin Orthop 2001.71-7.
- Childs LM, Goater JJ, O’Keefe RJ, Schwarz EM. Efficacy of etanercept for wear debris-induced osteolysis. J Bone Min Res 2001;16:338-47.
- Epstein NJ, Warme BA, Spanogle J, Ma T, Bragg B, Smith RL, et al. Interleukin-1 modulates periprosthetic tissue formation in an intramedullary model of particle-induced inflammation. J Orthop Res 2005;23:501-10.
- Rawal SY, Rawal YB. Non-antimicrobial properties of tetracyclines–dental and medical implications. West Indian Med J 2001;50:105-8.
- Bettany JT, Peet NM, Wolowacz RG, Skerry TM, Grabowski PS. Tetracyclines induce apoptosis in osteoclasts. Bone 2000;27:75-80.
- Holmes SG, Still K, Buttle DJ, Bishop NJ, Grabowski PS. Chemically modified tetracyclines act through multiple mechanisms directly on osteoclast precursors. Bone 2004;35:471-8.
- Childs LM, Goater JJ, O’Keefe RJ, Schwarz EM. Effect of anti-tumor necrosis factor-alpha gene therapy on wear debris-induced osteolysis. J Bone Joint Surg Am 2001;83:1789-97.
- Schwarz EM, Benz EB, Lu AP, Goater JJ, Mollano AV, Rosier RN, et al. Quantitative small-animal surrogate to evaluate drug efficacy in preventing wear debris-induced osteolysis. J Orthop Res 2000;18:849-55.
- Yasuda H, Shima N, Nakagawa N, Yamaguchi K, Kinosaki M, Mochizuki S, et al. Osteoclast differentiation factor is a ligand for osteoprotegerin/osteoclastogenesis-inhibitory factor and is identical to TRANCE/RANKL. Proc Natl Acad Sci USA 1998;95:3597-602.
- Sobue T, Hakeda Y, Kobayashi Y, Hayakawa H, Yamashita K, Aoki T, et al. Tissue inhibitor of metalloproteinases 1 and 2 directly stimulate the bone-resorbing activity of isolated mature osteoclasts. J Bone Miner Res 2001;16:2205-14.
- Ragab AA, Van De Motter R, Lavish SA, Goldberg VM, Ninomiya JT, Carlin CR, et al. Measurement and removal of adherent endotoxin from titanium particles and implant surfaces. J Orthop Res 1999;17:803-9.
- Munger P, Roder C, Ackermann-Liebrich U, Busato A. Patient-related risk factors leading to aseptic stem loosening in total hip arthroplasty: a case-control study of 5,035 patients. Acta Orthop 2006;77:567-74.
- Wright TM, Goodman SB. Implant wear in total joint replace-ment: clinical and biologic issues, material and design considera-tions. American Academy of Orthopaedic Surgeons; 2001. ()http://www4.aaos.org/product/productpage.cfm?code=02522
- Porter ML. The long-term results of Charnley low-friction arthroplasty in young patients who have congenital dislocation, degenerative osteoarthrosis, or rheumatoid arthritis. J Bone Joint Surg Am. 1997; 79-Am: 1599–617.
- von Knoch F, Heckelei A, Wedemeyer C, Saxler G, Hilken G, Brankamp J, et al. Suppression of polyethylene particle-induced osteolysis by exogenous osteoprotegerin. J Biomed Mater Res A 2005;75:288-94.
- Carmody EE, Schwarz EM, Puzas JE, Rosier RN, O’Keefe RJ. Viral interleukin-10 gene inhibition of inflammation, osteoclasto-genesis, and bone resorption in response to titanium particles. Arthritis Rheum 2002;46:1298-308.
- Iwase M, Kim KJ, Kobayashi Y, Itoh M, Itoh T. A novel bisphos-phonate inhibits inflammatory bone resorption in a rat osteolysis model with continuous infusion of polyethylene particles. J Orthop Res 2002;20:499-505.
- Wise LM, Waldman SD, Kasra M, Cheung R, Binnington A, Kandel RA, et al. Effect of zoledronate on bone quality in the treatment of aseptic loosening of hip arthroplasty in the dog. Calcif Tissue Int 2005;77:367-75.
- von Knoch F, Heckelei A, Wedemeyer C, Saxler G, Hilken G, Henschke F, et al. The effect of simvastatin on polyethylene particle-induced osteolysis. Biomaterials 2005;26:3549-55.
- Schwarz EM, Campbell D, Totterman S, Boyd A, O’Keefe RJ, Looney RJ. Use of volumetric computerized tomography as a primary outcome measure to evaluate drug efficacy in the prevention of peri-prosthetic osteolysis: a 1-year clinical pilot of etanercept vs placebo. J Orthop Res 2003;21:1049-55.
- Wang CJ, Wang JW, Weng LH, Hsu CC, Huang CC, Chen HS. The effect of alendronate on bone mineral density in the distal part of the femur and proximal part of the tibia after total knee arthroplasty. J Bone Joint Surg Am 2003; 85-Am: 2121–6.
- Talmo CT, Shanbhag A, Rubash HE. Nonsurgical management of osteolysis: challenges and opportunities. Clin Orthop 2006.254-64.