Co-expression of KCNE2 and KChIP2c modulates the electrophysiological properties of Kv4.2 current in COS-7 cells1
Introduction
The transient outward current (Ito) underlies the early repolarization phase of cardiac action potential and contributes to the determination of the overall repolarization phase as well as excitation–contraction coupling via modulating Ca2+ and other K+ currents[1,2]. It is well established that Kv4.x (x=2 and/or 3) are the major α-subunits of Ito channels in the heart[1,2]. The electrophysiological properties of Ito are modulated by several β-subunits, among them the K+-channel interacting protein (KChIP2) is the most thoroughly investigated. KChIP2 can increase peak Ito current density by promoting trafficking of Kv4.x to the cell membrane, slow inactivation, and accelerate recovery from inactivation[3]. Co-expression of Kv4.x and KChIP2 in mammalian cell lines yields current with a time course of inactivation similar to native cardiac Ito, but markedly slows recovery from inactivation and steady-state inactivation in a more positive potential range, suggesting that KChIP2 may not be the only accessory subunit of native Ito in cardiomyocytes[4].
Recently, KCNE2 protein has been found to be readily detectable in the ventricles of humans, dogs, and rats, and forms stable complex with the cardiac Kv4.2 protein[5]. In Xenopus oocyte expression system, KCNE2 slowed the rates of Kv4.2 activation and inactivation and shifted the voltage dependence of channel gating in the positive direc-tion, but did not affect the current amplitude[6]. Our previous data also demonstrated that KCNE2 had a similar modulation effect on Kv4.3 in COS-7 expression system[7]. This evidence indicates that KCNE2 may also serve as a regulatory (b) subunit of Ito channels in the heart.
However, it remains elusive how Kv4.2 current is regulated in the context of cardiac electrophysiology in which both KChIP2 and KCNE2 are expressed. It is unknown whether KCNE2 can still exert its regulation function on Kv4.2 in the presence of KChIP2, the obligatory β-subunit of Ito. To address these questions, we co-expressed these 2 regulatory subunits with Kv4.2, and compared the current properties with Kv4.2+KChIP2c current. We found that co-expression of KCNE2 produced a current profile with slower inactivation and faster recovery from inactivation as compared with Kv4.2+KChIP2c current. Moreover, co-expression of KCNE2 diminished the positive shift in voltage dependence of activation, but had no effect on the positive shift in voltage dependence of inactivation induced by KChIP2c alone.
Materials and methods
Cell culture and transfection Kv4.2 gene was subcloned into pcDNA3.1 vector, and KChIP2c- and c-myc-tagged KCNE2 genes were subcloned into pALTER-MAX vector. All the plasmid cDNA were kindly supplied by Dr Gea-Ny TSENG (Virginia Commonwealth University, Richmond, VA, USA). COS-7 cells were maintained in Dulbecco’s modified Eagle’s medium supplemented with 10% fetal calf serum in a moist 5% CO2 chamber at 37 ℃. Cells were plated at a subconfluence level the day before transfection and were divided into Kv4.2, Kv4.2+KChIP2c, and Kv4.2+KChIP2c+KCNE2 groups. Each 35-mm dish of cells in Kv4.2+KChIP2c+KCNE2 group was transfected with the following amounts of plasmid cDNA: Kv4.2, 2 µg; KChIP2c- and c-myc-tagged KCNE2, 0.58 µg each; and CD8, 0.2 µg, to reach a cDNA molar ratio of 3:1:1:0.5 using Lipofectamine 2000 (Invitrogen, USA) according to the manufacturer’s instructions. In the other 2 groups, the amount of cDNA for the transfection was the same as that in Kv4.2+KChIP2c+KCNE2 group, except neither KCNE2 nor KChIP2c was added in Kv4.2 group, and no KCNE2 was added in Kv4.2+KChIP2c group. Before current recording, the cells were incubated with CD8 monoclonal antibody (mAb)-coated beads (Dynal Biotech, Norway) at room temperature for 10 min, and the cells decorated with CD8 beads were used for patch clamping.
Whole-cell current recording Twenty-four to forty-eight hours after transfection, whole-cell currents were recorded by the patch-clamp method using an Axopatch 200B amplifier (Axon Instruments, USA) at room temperature. The pipette solution contained the following (in mmol/L): KOH 120, aspartic acid 120, KCl 20, ATP(K) 10, EGTA 10, HEPES 10, and MgCl2 1, with pH adjusted to 7.3 with KOH. The pipette tip resistance was 2–5 MΩ when filled with pipette solution. Myocytes were superfused with Tyrode’s solution containing the following (in mmol/L): NaCl 126, MgCl2 0.5, KCl 4, CaCl2 2, and HEPES 5, and dextrose 1 mg/mL, with pH adjusted to 7.3 with NaOH. Currents were low-pass filtered at 1 kHz, stored on a computer, and analyzed offline. Clampfit (Axon, USA), EXCEL (Microsoft), and PeakFit were used for the data analysis.
Immunocytochemistry and confocal experiments COS-7 cells cotransfected with Kv4.2, KChIP2c and KCNE2 plasmids were fixed on coverslips with 4% paraformaldehyde and permeabilized by 0.5% Triton X-100. The cells were incubated with anti-c-myc-tagged mAb (Cell Signaling, Danvers, MA, USA) at a 1:2000 dilution and an anti-KChIP2 antibody (Santa Cruz Biotechnology, Santa Cruz, CA, USA) at a 1:50 dilution overnight at room temperature. The slides were then washed 3 times with phosphate-buffered saline and incubated with secondary antibodies (goat antimouse immunoglobulin G [IgG] labeled with fluorescent Alexa Flour-488 for c-myc KCNE2 and donkey antigoat IgG with Alexa Flour-594 for KChIP2c). Immunofluorescence was imaged with a fluorescent microscope. The excitation wave length for Alexa Flour-488 and Alexa Flour-594 was 488 nm and 543 nm, emitting fluorescence at >505 nm and >605 nm, respectively.
Statistics Data were expressed as mean±SEM. Two-tailed unpaired Student’s t-test and one-way ANOVA were applied when appropriate. P<0.05 was considered statistically significant.
Results
Effects of co-expression of KCNE2 and KChIP2c on Kv4.2 current density and gating kinetics Before patch-clamp experiment, we needed to address whether the cells studied by patch-clamp expressed all the proteins encoded by the cDNAs. Given that cells not expressing Kv4.2 yield no current, we needed to determine whether COS-7 cells transfected with Kv4.2, KChIP2c, and KCNE2 cDNA co-expressed KChIP2c and KCNE2 proteins. These 2 proteins were simultaneously probed with specific antibodies using immunocytochemistry. KChIP2c and KCNE2 were co-expressed in the same cells, as shown in Figure 1. The statistical analysis revealed 96% cells (n=200) with fluorescence were positive for both KChIP2c and KCNE2, whereas only 1% and 3% of cells were positive for KChIP2c and KCNE2 alone, respectively. It implicates that the currents we recorded in Kv4.2+KChIP2c+KCNE2 expressing cells were mainly Kv4.2+KChIP2c+KCNE2 currents, and the interference of Kv4.2+KChIP2c or Kv4.2+KCNE2 current can be ignored.
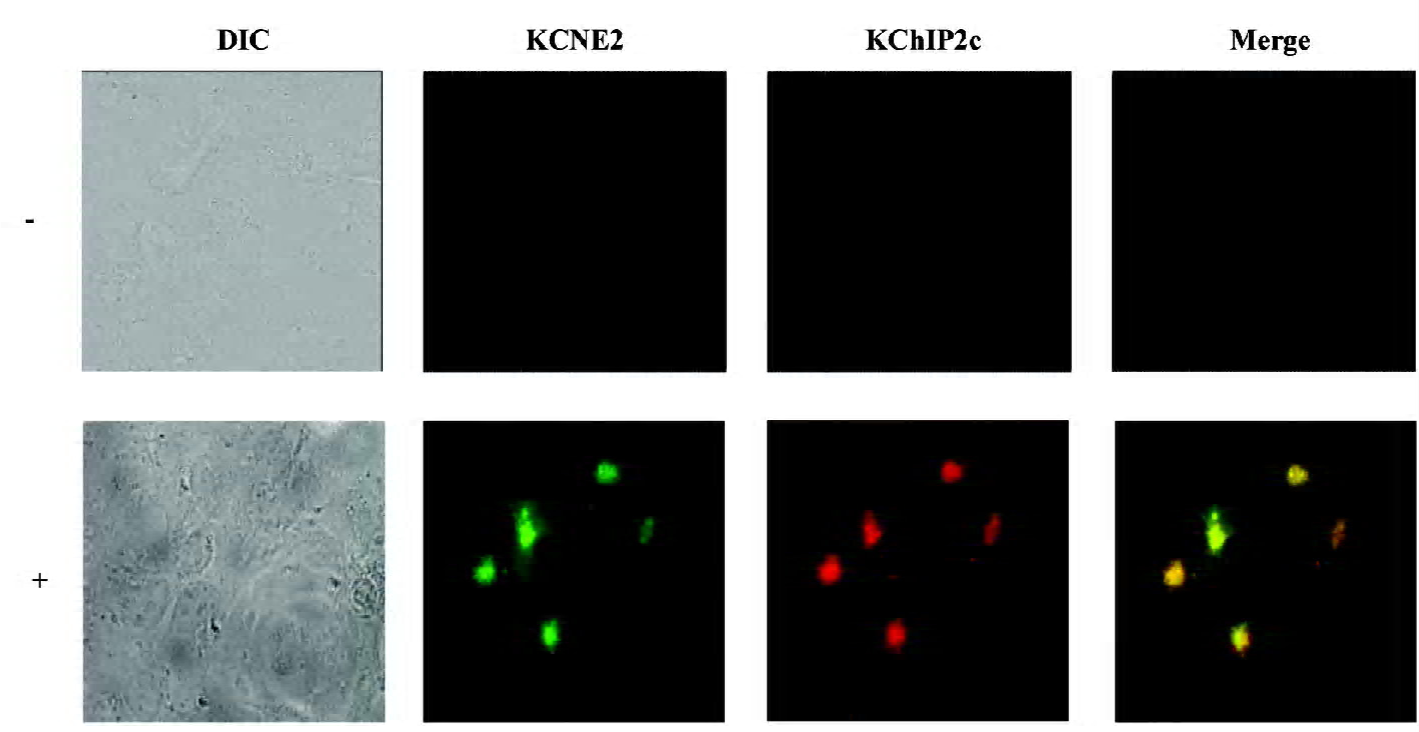
It is known that KChIP dramatically increases Kv4.2 current density, whereas KCNE2 has no significant effect on it. We first examined how the current density was regulated when both KChIP2c and KCNE2 were expressed with Kv4.2. Consistent with previous studies, KChIP2c alone significantly increased Kv4.2 current density (Figure 2A and 2B). The current density was increased by 1.3-fold, from 194.7±30.5 pA/pF (n=20) in Kv4.2 group to 449.0±40.5 pA/pF in Kv4.2+KChIP2c group (n=37, P<0.01). In comparison with the effect of KChIP2c alone, co-expression of KCNE2 did not change the current density. In Kv4.2+KChIP2c+KCNE2 group, the current density was 409.2±90.5 pA/pF (n=15), not significantly different from that in Kv4.2+KChIP2c group (P>0.05). These data suggest that KCNE2 might not interfere with KChIP2c regulation of Kv4.2 current density. In other words, KChIP2c dominantly regulates Kv4.2 current density when it is co-expressed with KCNE2.
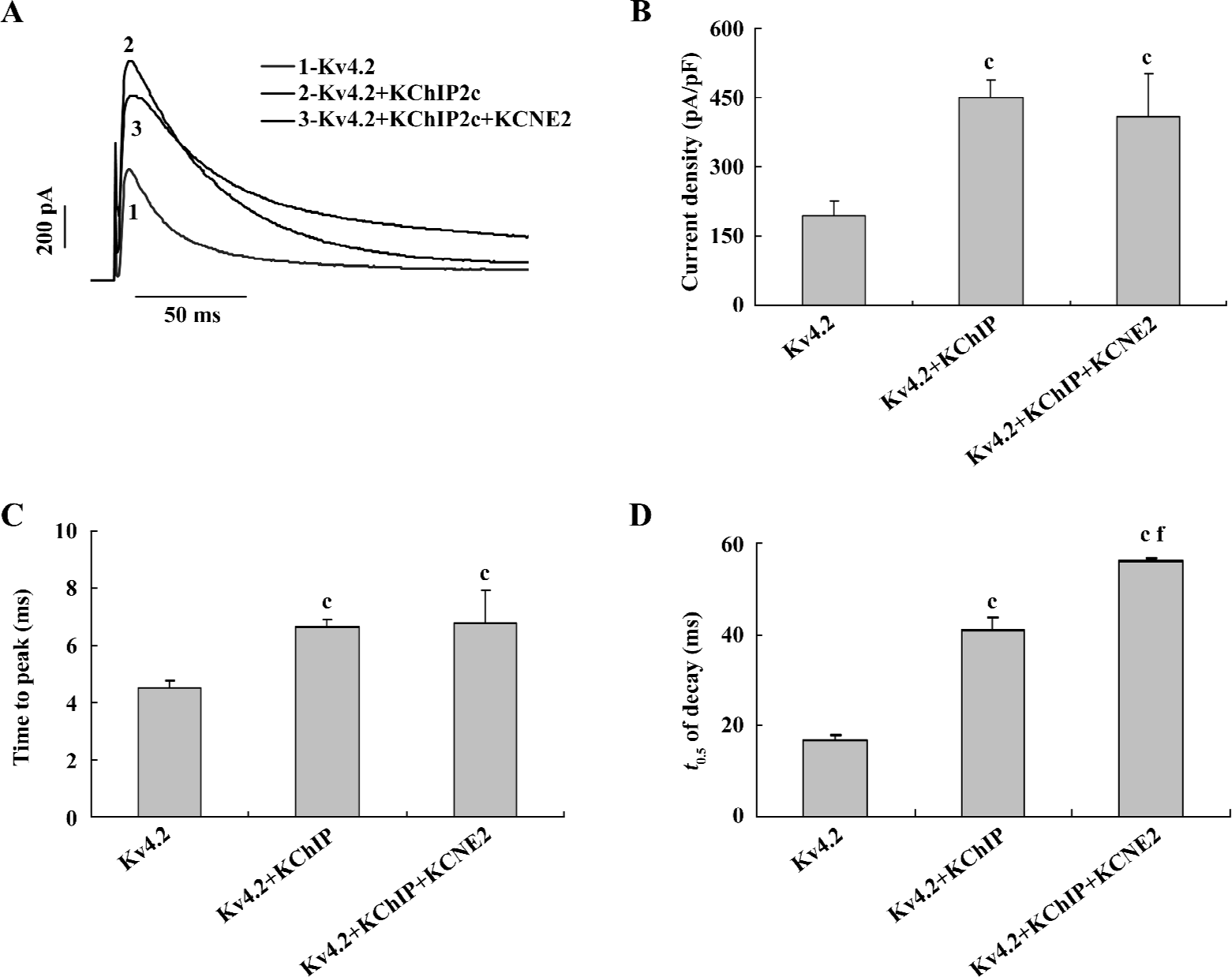
KCNE2 and KChIP have both been reported to slow the rates of Kv4.2 activation and inactivation. We next explored whether KCNE2 would further slow Kv4.2 activation and inactivation kinetics in the presence of KChIP2c. The activation and inactivation rates of Kv4.2, as indexed by the time to peak (TTP) and half-time (t0.5) of decay recorded at +60 mV potential were slowed down by the expression of KChIP2c alone (Figure 2C,2D). The TTP and t0.5 of decay in Kv4.2+KChIP2c group were 6.7±0.2 ms and 40.9±2.7 ms (n=37), much longer than 4.5±0.2 ms and 17.0±1.2 ms in Kv4.2 group (n=20, P<0.01). When co-expressed with KChIP2c, KCNE2 did not further slow the Kv4.2 activation rate. The TTP was 6.8±0.9 ms in Kv4.2+KChIP2c+KCNE2 group, not significantly different from that in Kv4.2+KChIP2c group (P>0.05). In contrast, co-expression of KCNE2 further slowed Kv4.2 inactivation kinetics. The t0.5 of the current decay was 56.0±0.7 ms in Kv4.2+KChIP2c+KCNE2 group, significantly longer than that in Kv4.2+KChIP2c group (P<0.01). These data indicate that KCNE2 does not homogenously regulate Kv4.2 current activation and inactivation kinetics in the presence of KChIP2c.
Effects of co-expression of KCNE2 and KChIP2c on voltage dependence of Kv4.2 current activation Previous studies have demonstrated that both KCNE2 and KChIP shift the voltage dependence of Kv4.2 current activation in a positive direction[4,5]. We examined how the voltage dependence of Kv4.2 current activation was regulated when both KCNE2 and KChIP2c were co-expressed. Voltage dependence of activation was obtained by normalizing steady-state activation (gm) curves calculated from current-voltage (I-V) curves elicited by test steps (200 ms) from –50 to 60 mV from a holding potential (Vh) of –80 mV, assuming an reversal potential (Erev) of –60 mV (Figure 3A,3B). The voltage of half activation (V0.5, act) was estimated by fitting the activation curves with Boltzman function. As shown in Figure 3C, the expression of KChIP2c caused an 8.5 mV positive shift of Kv4.2 current activation (V0.5,act was –12.7±1.8 mV, n=5 in Kv4.2 group, and –4.2±1.6 mV, n=10 in Kv4.2+KChIP2c group), which was consistent with a previous report[4]. When KCNE2 was co-expressed, the effect of KChIP2c on the voltage dependence of activation was weakened. The V0.5,act in Kv4.2+KChIP2c+KCNE2 group was –10.0±3.8 mV (n=5), which was only a 2.7 mV positive shift versus the value in Kv4.2 group (P>0.05).
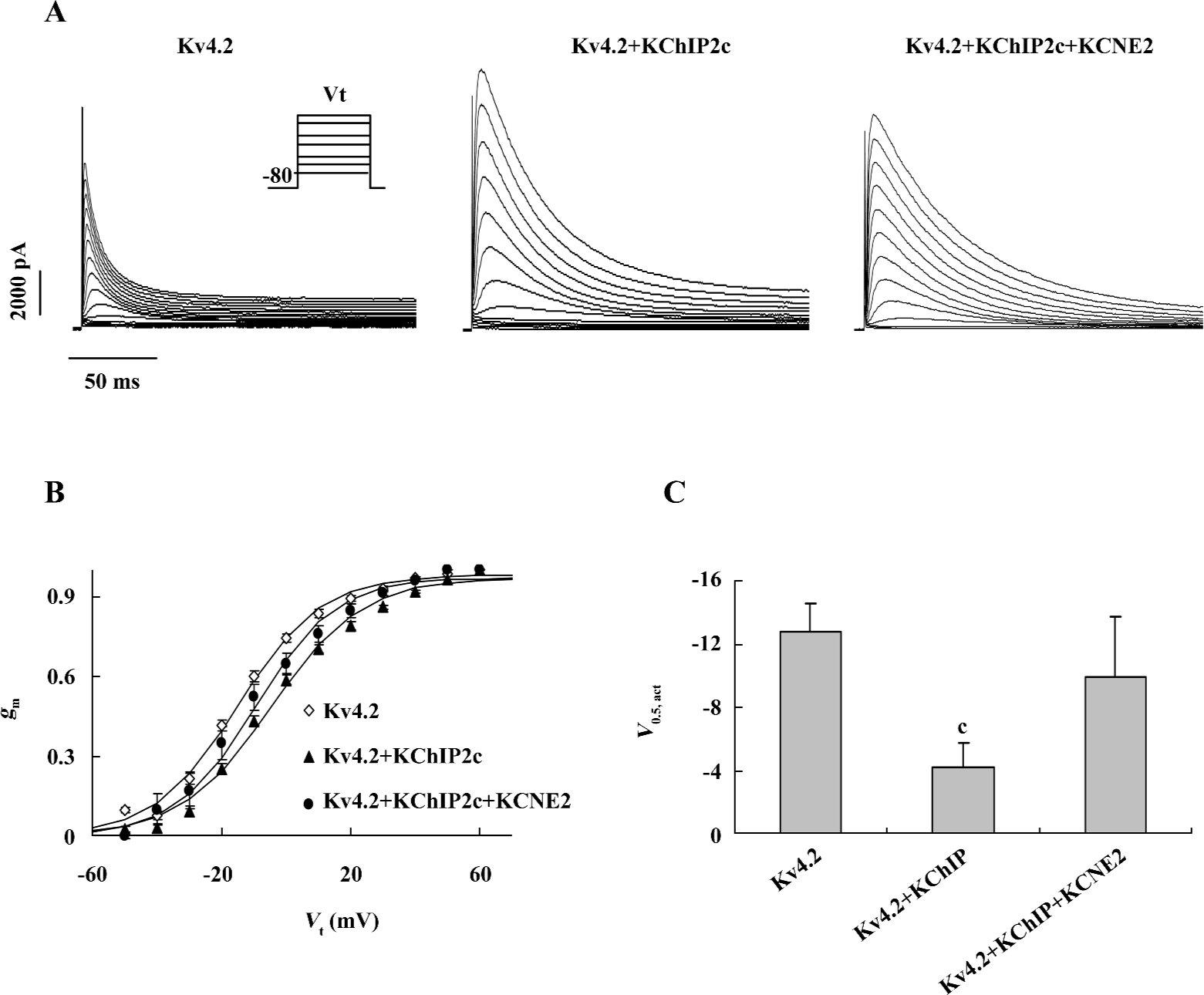
Effects of co-expression of KCNE2 and KChIP2c on voltage dependence of Kv4.2 current inactivation KChIP2c and KCNE2 have also been reported to cause a significant positive shift in the voltage dependence of Kv4.2 current inactivation. We next investigated whether KCNE2 influenced KChIP2c regulation of voltage-dependent Kv4.2 inactivation in a similar way as it did on voltage-dependent Kv4.2 activation. The voltage-dependent inactivation was estimated by V0.5,inact calculated by fitting the inactivation curve with Boltzman function (Figure 4A). In comparison with the effect of KChIP2c, co-expression of KCNE2 caused no further alteration in the voltage dependence of inactivation. V0.5,inact was –42.8±1.6 mV in Kv4.2+KChIP2c+KCNE2 group (n=10), not significantly different from –39.6±1.8mV in Kv4.2+KChIP2c group (n=15, P>0.05; Figure 4B).
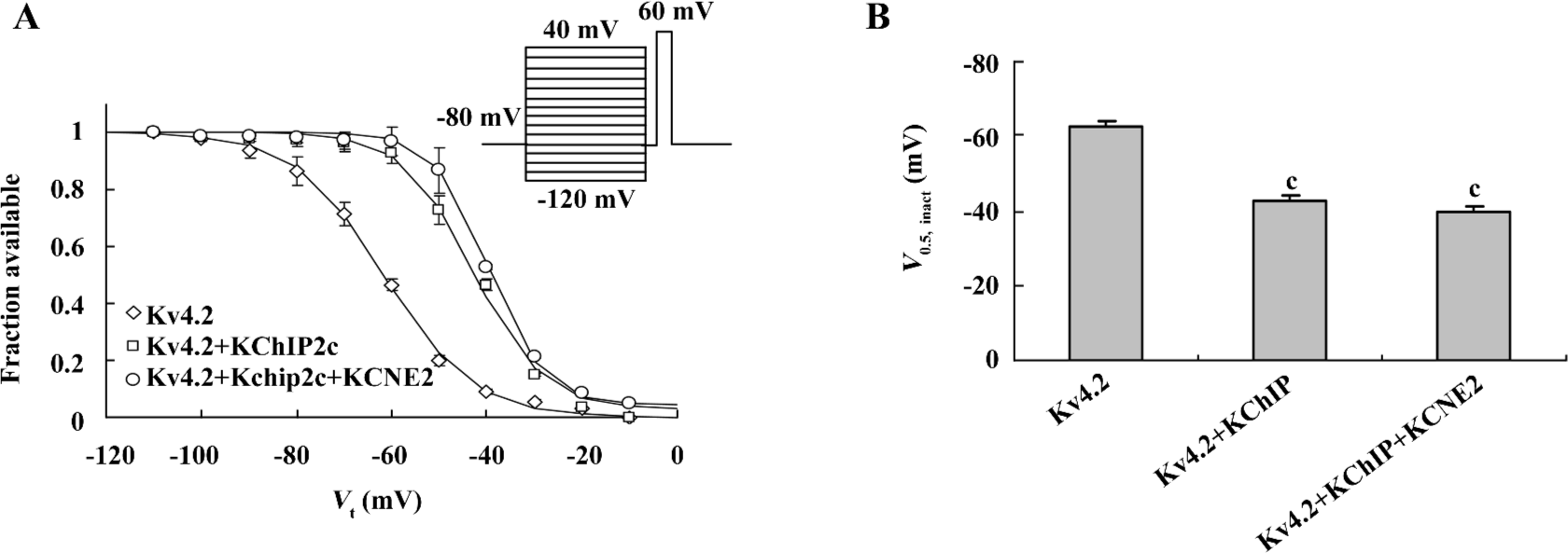
Effects of co-expression of KCNE2 and KChIP2c on voltage dependence of Kv4.2 current recovery from inactivation Double pulses of depolarization stimulation (V1 and V2, +60 mV) with different time intervals ranging from 12 ms to 2 s were applied (Figure 5) for studying the time course of Kv4.2 recovery from inactivation. The peak Kv4.2 current amplitude recovered from inactivation gradually with the prolongation of the V1-V2 interval, in a monoex-ponential function (Figure 5A and 5C). Consistent with previous reports, KChIP2c accelerated Kv4.2 recovery from inactivation. The time constant (Tau) of recovery was shortened from 344.9±36.8 ms (n=9) in Kv4.2 group to 66.7±8.9 ms (n=9) in Kv4.2+KChIP2c group. When KCNE2 was co-expressed, the recovery was further accelerated. The time constant of recovery was reduced to 34.1±5.5 ms in Kv4.2+KChIP2c+KCNE2 group (n=6), significantly shorter than that in Kv4.2+KChIP2c group (P<0.05; Figure 5D).
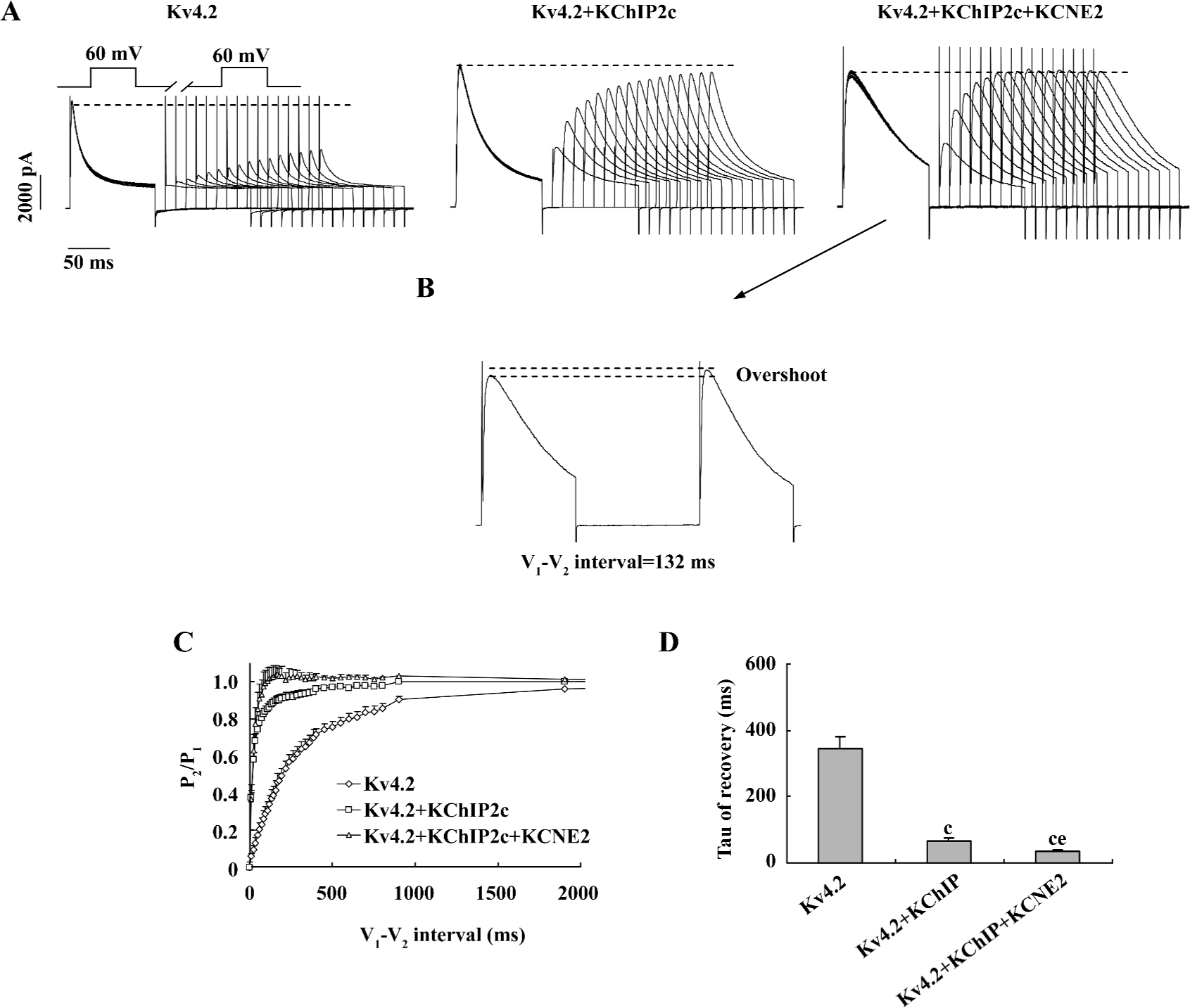
It is noteworthy that the co-expression of KCNE2 induced an overshoot of Kv4.2 peak current amplitude during recovery from inactivation, that is, the peak V2 current amplitude (P2) was approximately 4% larger than the V1 current amplitude (P1; Figure 5B). This phenomenon was not found in Kv4.2 and Kv4.2+KChIP2c-expressing cells.
Discussion
KChIP2 is abundantly expressed in the heart and has a prominent effect on increasing peak Ito current density. It is reported that KChIP2 expression in the ventricular wall of the heart parallels the gradient in transient outward current Ito[8-10]. Thus KChIP2 is well accepted as the obligatory β-subunit of Ito. Although KChIP2 can associate with Kv4.x and modulates the current gating, endowing Kv4.x electrical properties similar to Ito, co-expression of Kv4.x with KChIP2 cannot fully recapitulate the electrophysiological properties of native Ito in cardiac myocytes, suggesting that Ito is complicatedly regulated by multiple factors, rather than a single factor in the context of cardiac myocytes. KCNE2 is also expressed in ventricles and has been suggested to be a potential regulatory subunit of Ito in cardiac myocytes[5-7]. In the present study, we found that co-expression of KCNE2 with KChIP2c further slowed Kv4.2 current inactivation kinetics, but diminished KChIP2c-induced positive shift of voltage-dependent activation of Kv4.2 current. Importantly, co-expression of KCNE2 accelerated the current recovery from inactivation and caused an “overshoot” of peak current amplitude during Kv4.2 current recovery, a phenomenon which has been uniquely described for human Ito[11]. This evidence collectively suggests that KChIP2c and KCNE2 are simultaneously involved in the recapitulation of the electrophysiological properties of native Ito in the context of cardiac myocytes.
It was noteworthy that co-expression of KCNE2 failed to change some of the current properties in comparison with the current modulated by KChIP2c alone. There were no significant differences in the Kv4.2 current activation rate and voltage dependence of inactivation between Kv4.2+KChIP2c and Kv4.2+KChIP2c+KCNE2 groups, even though KCNE2 alone can slow the rate of Kv4.2 activation and induce a positive shift of voltage-dependent inactivation. Moreover, co-expression of KCNE2 did not change Kv4.2+KChIP2c current density in our expression system. A possible explanation for these results might be that KCNE2 does not homogenously regulate Kv4.2 gating properties when transfected at a lower concentration with KChIP2c (molar ratio for Kv4.2: KCNE2 was 3:1), that is, it exerts a regulatory effect on the inactivation rate and recovery from inactivation, but not on the activation rate and voltage-dependent inactivation of Kv4.2 current. Furthermore, the finding that co-expression of KCNE2 diminished, rather than enhanced the KChIP2c-induced positive shift of voltage-dependent activation suggests that co-expression of KChIP2c and KCNE2 might change the pattern of KCNE2 and/or KChIP2c modulation of the Kv4.2 current.
A recent study has reported the KCNE2 modulation of Ito current properties in CHO cells constantly expressing both Kv4.3 and KChIP2[12]. In this cell model, Radicke et al revealed that KCNE2 had no effect on current density, similar to our finding. However, in contrast to our study, these authors reported that KCNE2 had no effect on inactivation kinetics, but caused a negative shift in the voltage-dependent inactivation of Kv4.3+KChIP2c current. Although KCNE2 induced a unique “overshoot” of the peak current during recovery from inactivation, it did not increase the recovery from inactivation, which is much slower than native Ito. The different findings between the study from Radicke et al and the present one were likely to be related to the following reasons. First, Kv4.3 was used in the study of CHO cell expression, whereas Kv4.2 was used in the present study. Although Kv4.2 and Kv4.3 share many similarities in gating properties, the activation and inactivation kinetics, and the recovery of Kv4.3 channel current from inactivation are slower than those of Kv4.2[13]. Second, in our study, KChIP2c and KCNE2 were cotransfected simultaneously, whereas KCNE2 was transiently transfected into CHO cells stably expressing KChIP2 in the study of Radicke et al[12]. Third, stoichiometric transfection with Kv4.2, KChIP2 and KCNE2 was employed in the present study, but not in the study from Radicke et al[12]. In the present study, KChIP2c and KCNE2 were transfected at the same molar ratio and at a lower concentration. There is no report on the stoichiometry of Kv4.x, KChIP2, and KCNE2 in native Ito in a normal heart. In diseased and aging hearts, KChIP2 and/or KCNE2 expression levels have been reported to be downregulated or upregulated[5,13,14]. Therefore, the different results from the previous study and the present one suggest that the electrophysiological properties of Ito might be differentially regulated under physiological and pathological conditions.
In summary, we demonstrated the regulation pattern of co-expression of KCNE2 and KChIP2c on Kv4.2 current, which produced a current similar to native Ito. Future experiments need to be conducted to address how Ito is regulated under diseased conditions by changing stoichiometry of α- and β-subunits of Ito to simulate diseased conditions. It is also of interest to investigate how long QT syndrome-related KCNE2 mutations, which predispose the heart to arrhythmia[15], regulate native Ito (with the presence of KChIP2) properties.
Author contributions
Wen-juan LIU and Hai-tang WANG contributed equally to this work; Jie LIU designed research; Wen-juan LIU, Hai-tang WANG, Wei-wei CHEN, Jian-xin DENG, and Yong JIANG performed research; Wen-juan LIU and Jie LIU analyzed data; and Jie LIU wrote the paper.
References
- Barry DM, Trimmer JS, Merlie JP, Nerbonne JM. Differential expression of voltage-gated K+ channel subunits in adult rat heart: relation to functional K+ channels? Circ Res 1995;77:361-9.
- Dixon JE, Shi W, Wang HS, McDonald C, Yu H, Wymore RS, et al. Role of the Kv4.3 K+ channel in ventricular muscle: A molecular correlate for the transient outward current. Circ Res 1996;79:659-68.
- An WF, Bowlby MR, Betty M, Cao J, Ling HP, Mendoza G, et al. Modulation of A-type potassium channels by a family of calcium sensors. Nature 2000;403:553-6.
- Deschênes I, DiSilvestre D, Juang GJ, Wu RC, An WF, Tomaselli GF. Regulation of Kv4.3 current by KChIP2 splice variants: A component of native cardiac Ito? Circulation 2002;106:423-9.
- Jiang M, Zhang M, Tang DG, Clemo HF, Liu J, Holwitt D, et al. KCNE2 protein is expressed in ventricles of different species, and changes in its expression contribute to electrical remodeling in diseased hearts. Circulation 2004;109:1783-8.
- Zhang M, Jiang M, Tseng GN. MinK-related peptide 1 associates with Kv4.2 and modulates its gating function: potential role as ß subunit of cardiac transient outward channel? Circ Res 2001;88:1012-9.
- Liu J, Deng JX, Pan BX, Huang QB. KCNE2 modulates the function of Kv4.3 channel. J South Med Univ 2006;26:1754-6. (in Chinese).
- Patel SP, Campbell DL, Morales MJ, Strauss HC. Heterogeneous expression of KChIP2 isoforms in the ferret heart. J Physiol 2002;539:649-56.
- Rosati B, Grau F, Rodriguez S, Li H, Nerbonne JM, McKinnon D. Concordant expression of KChIP2 mRNA, protein and transient outward current throughout the canine ventricle. J Physiol 2003;548:815-22.
- Rosati B, Pan Z, Lypen S, Wang HS, Cohen I, Dixon JE, et al. Regulation of KChIP2 potassium channel beta subunit gene expression underlies the gradient of transient outward current in canine and human ventricle. J Physiol 2001;533:119-25.
- Wetter E, Amos GJ, Posival H, Ravens U. Transient outward current in human ventricular myocytes of subepicardial and subendocardial origin. Circ Res 1994;75:473-82.
- Radicke S, Cotella D, Graf EM, Banse U, Jost N, Varro A, et al. Functional modulation of the transient outward current Ito by KCNE β-subunits and regional distribution in human non-failing and failing hearts. Cardiovasc Res 2006;71:695-703.
- Wickenden AD, Jegla TJ, Kaprielian R, Backx PH. Regional contributions of Kv1.4, Kv4.2, and Kv4.3 to transient outward K+ current in rat ventricle. Am J Physiol 1999;276:H1599-H1607.
- Liu XS, Jiang M, Zhang M, Tang D, Clemo HF, Higgins RSD, et al. Electrical remodeling in a canine model of ischemic cardio-myopathy. Am J Physiol Heart Circ Physiol 2007;292:H560-71.
- Abbott GW, Sesti F, Splawski I, Buck ME, Lehmann MH, Timothy KW, et al. MiRP1 forms IKr potassium channels with HERG and is associated with cardiac arrhythmia. Cell 1999;97:175-87.