Bilobalide inhibits 6-OHDA-induced activation of NF-κB and loss of dopaminergic neurons in rat substantia nigra1
Introduction
Parkinson’s disease (PD) is a motor function disorder caused primarily by the loss of the neurotransmitter dopamine (DA) in the central nervous system (CNS) as a result of selective degeneration of nigral dopaminergic neurons[1-3]. It is generally believed that both genetic and environmental factors play roles in PD, but the exact mechanisms of neuronal death have not been fully understood. Several scientific reports demonstrate that apoptosis of the neurons in the substantia nigra (SN) plays an important role in the development of PD. A number of studies suggest that oxidative stress contributes to dopaminergic neuron degeneration. In previous studies, we found that the transcription factor NF-κB plays a pro-apoptotic role in the excitotoxin-induced apoptotic death of neurons in the SN par compact (SNpc), possibly through upregulating p53 and c-Myc[4-6]. Increased NF-κB immunoreactivity has been reported in the SNpc of patients with PD, which suggests that NF-κB plays a role in oxidative stress-induced dopaminergic neuron degeneration in PD[7].
Although various treatments are successfully used to alleviate the symptoms of PD, none of them prevents or halts the neurodegenerative process of the disease. Clinical studies have shown that ginkgo extracts, which have been widely used as a dietary supplement in the United States, exhibit several beneficial effects in a variety of CNS disorders, including Alzheimer’s disease (AD) and PD[8-11].
The active components of Ginkgo biloba are considered to be the ginkgo flavone glycosides, the polycyclic lactone ginkgolides A, B, C, J, and bilobalide[12]. EGb 761, a standard extract of Ginkgo biloba (EGb) with potent antioxidant properties, shows great benefits on the CNS, being able to enhance peripheral and cerebral circulation[13], and protecting neurons against a variety of insults.
Several studies have reported that EGb exerts beneficial effects in AD and PD models. A recent study indicated that that administration of EGb 761 can reduce β-amyloid oligomers and restore cAMP respond element binding protein (CREB) phosphorylation in the hippocampus of a transgenic mouse model of AD[14]. EGb pretreatment is able to reverse β-amyloid-peptide-induced isoprostane production in the rat brain in vitro[15] and inhibit cerebral monoamine oxidase (MAO) activity in vivo[16]. Wu and Zhu[17] demonstrated that EGb could attenuate 1-methyl-4-phenyl-1,2,3,6-tetrahydropyridine (MPTP)-induced nigrostriatal dopaminergic neurotoxicity in C57 mice. Another study suggested that EGb offers dose-dependent protection against 6-hydroxydopamine (6-OHDA)-induced parkinsonism in rats. The pretreatment of rats with EGb leads to a restoration of compromised behavioral activity, levels of tyrosine hydroxylase (TH), and neurotransmitter DA[18]. Cao et al[19] found that the combined use of EGb with levodopa reduces the toxic effects of levodopa, and thus may be a therapeutic strategy in the management of motor function in PD.
Bilobalide, a sesquiterpene lactone, which constitutes of approximately 3% EGb 761, is quantitatively the major single chemical constituent of EGb 761. The first pharmacological action detected was a beneficial effect on cytotoxic brain edema caused by triethyltin[20]. Bilobalide has now been demonstrated to inhibit delayed ischemic neuronal death and reduce infarct volume after focal cerebral ischemia and ischemia-induced neuronal damage in rodents[21-24]. Zhou et al[25] demonstrated that bilobalide could protect against β-amyloid toxicity and reactive oxygen species (ROS)-induced apoptosis in PC12 cells. It has also been demonstrated that bilobalide has potent inhibitory actions on the N-methyl-D-aspartate-induced activation of phospholipase A2 and the associated phospholipid breakdown in the brain[26].
These studies suggest that bilobalide is a main active component in the neuroprotective actions of EGb, but it has not been reported whether bilobalide has a protective effect on the neurodegeneration in PD or not. In the present study, we explored the neuron protective effects of bilobalide in a rat model of PD induced by 6-OHDA. We report that neuron pathology and behavioral changes can be restored effectively by pretreatment with bilobalide, suggesting that bilobalide may be a candidate to alleviate the Parkinson-related pathology.
Materials and methods
Animals Male Sprague–Dawley rats were obtained from the Center for Experimental Animals, Soochow University (Suzhou, China), and weighed 250–280 g at the start of the experiment. The rats were housed under standardized light/dark cycle (12 h) conditions with access to food and water ad libitum. All procedures were performed in accordance with the NIH Guidelines for the Care and Use of Laboratory Animals.
Treatment with bilobalide Bilobalide was provided by Zhong-liang CHEN (Department of Phytochemistry, Shanghai Institute of Materia Medica, Chinese Academy of Sciences, Shanghai, China), and the purity of this compound was 98% (HPLC). Bilobalide was dissolved in DMSO and then diluted with 0.9% sodium chloride. The final concentration of DMSO was 1% (v/v). Bilobalide was administered by intraperitoneal injection (ip) to rats once daily at doses of 5, 10, and 20 mg·kg–1·d–1 for 7 successive days, respectively.
PD model induced by 6-OHDA After 7 d of bilobalide treatment, the rats were anesthetized with 400 mg/kg chloral hydrate (ip), and then placed on a stereotaxic instrument (Stoelting, Wood Dale, IL, USA). A hole was drilled through the skull to the top of the dura, and a 29 gauge stainless steel needle was lowered to the left SNpc (coordinates: –5.2 mm from bregma, 2.1 mm from midline, 7.8 mm from surface). A solution of 6-OHDA with 0.1% ascorbic acid–saline (Sigma, St Louis, MO, USA) was dissolved in 0.9% NaCl (w/v) to a concentration of 4 µg/µL, and then infused unilaterally into the left SNpc by a microinfusion pump delivering 2 µL (8 µg) over a 5 min period. After infusion, the needle was kept in place for 5 min to ensure diffusion. Sham-operated animals received an equal volume of 0.9% NaCl delivered by the same method.
Animal grouping 1 The rats were divided into 6 groups, each consisting of 10 animals. Group 1 included sham-operated rats pretreated with vehicle (S); group 2 included sham-operated rats pretreated with 20 mg/kg bilobalide (S+H–BB); group 3 included PD model rats pretreated with vehicle (M); group 4 included PD model rats pretreated with 5 mg/kg bilobalide (M+L–BB); group 5 included PD model rats pretreated with 10 mg/kg bilobalide (M+M–BB); and group 6 included PD model rats pretreated with 20 mg/kg bilobalide (M+H–BB). The animals were used to evaluate the effect of pretreatment with bilobalide on the behavioral changes and neuron survival in 6-OHDA-lesioned rats.
Animal grouping 2 The rats were divided into 3 groups, each consisting of 10 animals. Group 1 included sham-operated rats pretreated with vehicle (control); group 2 included PD model rats pretreated with vehicle (6-OHDA); and group 3 included PD model rats pretreated with 10 mg/kg bilobalide (6-OHDA+M–BB). These animals were used to explore the mechanism involved in the effects of bilobalide in vivo.
Behavior studies On d 14 after a stereotaxic injection of 6-OHDA, the motor activity of the animals was tested for locomotor activity in a computerized animal activity video analyzer (Shanghai Jiliang Software Technology, Shanghai, China). Each rat was placed in the chamber and its locomotor activity was monitored by the activating camera and viewed on the screen. The activities of the animals at 5 min periods were recorded, and the data of the locomotion time were collected by individuals who were trained in behavioral observation.
On d 14 and 21 after infusion of 6-OHDA, the animals were subject to rotational behavior testing[27]. The rats were administered R-(2)-apomorphine hydrochloride (0.5 mg/kg in 0.1% ascorbic acid–saline, subcutaneously) and placed in a transparent cylindrical cage. Contralateral rotations (360°, in short axis) over a 30 min interval from the initiation of rotation were recorded.
Immunofluorescence of the brain sections Twenty four days after the infusion of 6-OHDA, the rats were anaesthetized and then perfused transcardially with 200 mL precooled 0.01 mol/L phosphate-buffered saline (PBS; pH 7.4), followed by 200 mL perfusate containing 4% paraformaldehyde in 0.1 mol/L phosphate buffer (PB, pH 7.4). The brains were postfixed overnight in the same paraformaldehyde fixative and then transferred to 20% sucrose solution until they sank to the bottom of the containers. The brains were snap frozen and sectioned at 30 mm thickness with a cryostat (Leica Microsystems GmbH, Wetzlar, Germany). Free-floating sections were washed in 0.01 mol/L PBS 3 times for 10 min each and incubated in PBS with 0.1% Triton X-100 for 1 h at room temperature (RT).The brain sections were then blocked with 1% bovine serum albumin (BSA) for 1 h at RT and then incubated with mouse monoclonal antibody TH (1:3000; Sigma, USA), a primary antibody recognizing TH, in PBS containing 0.1% Triton X-100 at 4 °C for 48 h. Sections were subsequently rinsed in PBS and incubated for 1 h with the secondary antibody (Cy3-conjugated donkey antimouse immunoglobulin G [IgG]; Jackson ImmunoResearch, West Grove, PA, USA). Sections were mounted on glass slides, coverslipped with antifade mountant, and then observed with a fluorescence microscope (Nikon, Tokyo, Japan).
Nissl’s staining The brain sections were stained with 0.75% cresyl violet, dehydrated twice through graded alcohols (70%, 95%, and 100%), cleared in xylenes 3 times for 5 min each, coverslipped with resinous mountant, and then observed with a light microscope.
Double immunofluorescence Approximately 6–24 h after the 6-OHDA infusion, the rats were perfused, and the brains were then frozen and sectioned according to the protocol described earlier. The brain sections were rinsed with PBS, incubated in PBS with 0.1% Triton X-100, blocked with 1% BSA in PBS, and then incubated with primary and secondary antibodies sequentially. To examine if the activation of NF-κB p65 occurs in nigral neurons, the brain sections were incubated with a mouse monoclonal antibody against TH (1:3000) and rabbit polyclonal antibody against NF-κB p65 (1:500; Chemicon, Temecula, CA, USA), and then incubated with the secondary antibodies (fluorescein-isothiocyanate [FITC]-conjugated donkey antimouse IgG, 1:1000 and Cy3-conjugated donkey antirabbit IgG, 1:1000). Sections were washed in PBS, mounted on glass slides, coverslipped, and then examined with a laser confocal system (Leica Microsystems GmbH, Wetzlar, Germany).
Terminal deoxynucleotidyl transferase-mediated dUTP nick-end labeling The low molecular weight DNA fragments as well as the single strand breaks (“nicks”) in high molecular weight DNA can be identified by labeling free 3'-OH termini with modified nucleotides by an enzymatic reaction with terminal deoxynucleotidyl transferase, which catalyzes polymerization of nucleotides to free 3'-OH DNA ends in a template-independent manner. This method labels these hydroxyl groups with fluorescein-conjugated deoxynucleotides. Fluorescein generates an intense signal that can be detected by a fluorescence microscope.
Twenty four hours after the 6-OHDA infusion, the rat brain sections were prepared according to the protocol described earlier. The brain sections were incubated with a mouse monoclonal antibody against TH, and reacted with a secondary antibody (Cy3-conjugated donkey antimouse IgG). Then the brain sections were mounted on polylysine-coated glass slides. DNA damage was detected using a Fluorescein FragEL DNA fragmentation detection kit (Calbiochem, San Diego, CA, USA) according to the protocol of the manufacturer. The sections were then washed in PBS, counterstained with 4',6'-diamidino-2-phenylindole dihydrochloride (DAPI), and coverslipped. To determine the relationship between NF-κB p65 and DNA damage, the brain sections were incubated with rabbit polyclonal antibodies against NF-κB p65, and subsequently reacted with secondary antibodies (Cy3-conjugated donkey antirabbit IgG). DNA damage was then detected as described earlier. Slides were observed with a laser confocal system.
Cell counting Staining with a TH antibody can delineate the SNpc in coronal sections. The sections were immunofluorescent labeled for TH. Stained cells were counted within the outlines, and total estimates were obtained. Labeled profiles were counted only if the first recognizable profile of the cell soma came into focus within the counting frame[28]. Using every coronal section, the analysis was performed starting with the first appearance of TH-positive neurons, extending to the most caudal parts of the SNpc and including both hemispheres. Sections were viewed under an inverted fluorescence microscope. Each group included 6 rats, and 6 brain sections from each rat were counted.
An estimation of the percentages of TH-positive neurons having undergone recombination with NF-κB p65 was obtained by montage images (FITC and Cy3) of the left SNpc under a laser confocal system. The total number of TH-positive neurons and total number of TH/NF-κB double-positive neurons were counted for each section. The number of double-labeled neurons divided by the total number of TH-positive neurons was the percentage of recombination, which showed the activation of NF-κB in each group. Each group included 6 rats, and 6 brain sections from each rat were counted.
Statistical analysis Data are presented as mean±SEM. One-way ANOVA or the Student’s unpaired two-tailed test was used for the statistical analysis. Statistical significance was set at P<0.05.
Results
Bilobalide restored 6-OHDA-induced impairment on motor activity In the PD model group, the motor activity was reduced significantly as compared to the sham-operated group (S). The time spent on locomotion was significantly decreased (76.9%). Different doses of bilobalide (L–BB, M–BB, and H–BB) remarkably restored the locomotion time (23.2%, 45.8%, and 58.0%) as compared to the PD model group (M) respectively (P<0.05; Table 1). However, no significant effects on motor activity were observed in the sham-operated group treated with 20 mg/kg bilobalide as compared to the sham-operated group (S).
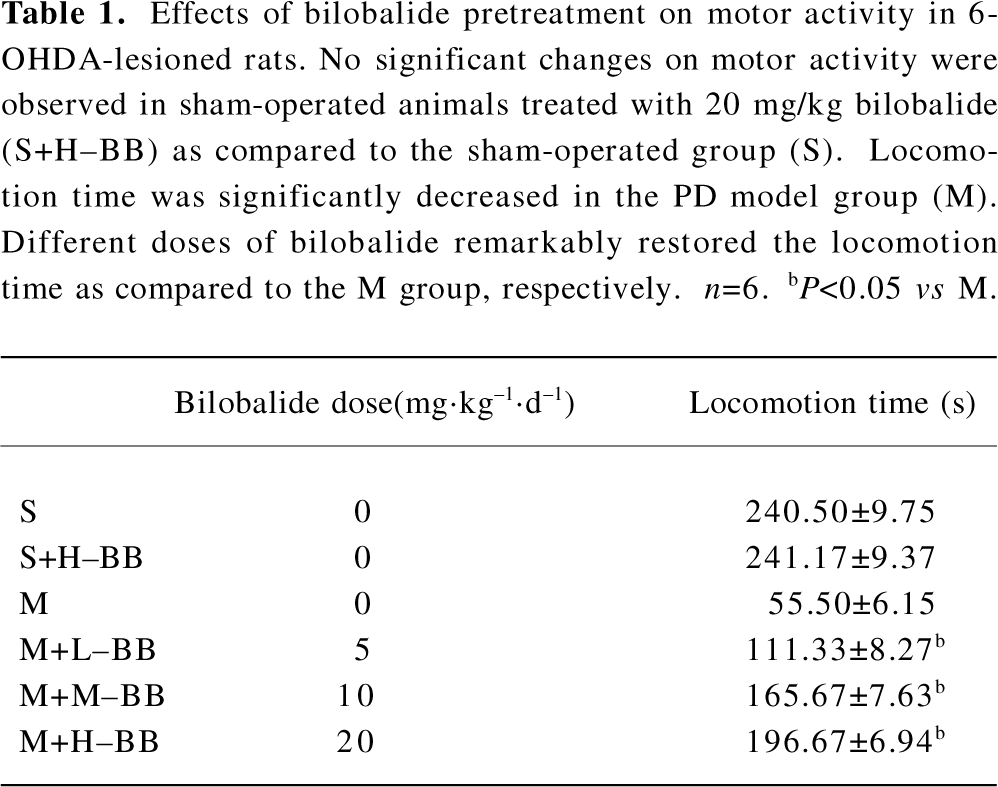
Full table
Bilobalide inhibited apomorphine-induced circling behavior The sham-operated animals failed to exhibit rotational behavior upon apomorphine challenge. The PD model rats exhibited contralateral rotations after the administration of apomorphine (430±40 turns/30 min). Pretreatment with different doses of bilobalide significantly reduced contralateral rotations by a dose-dependent manner in the M+L–BB, M+M–BB, and M+H–BB groups, as compared to the PD model rats (P<0.05; Table 2). Similar inhibitory effects of bilobalide on rotational behavior were observed at 2 and 3 weeks after 6-OHDA damage.
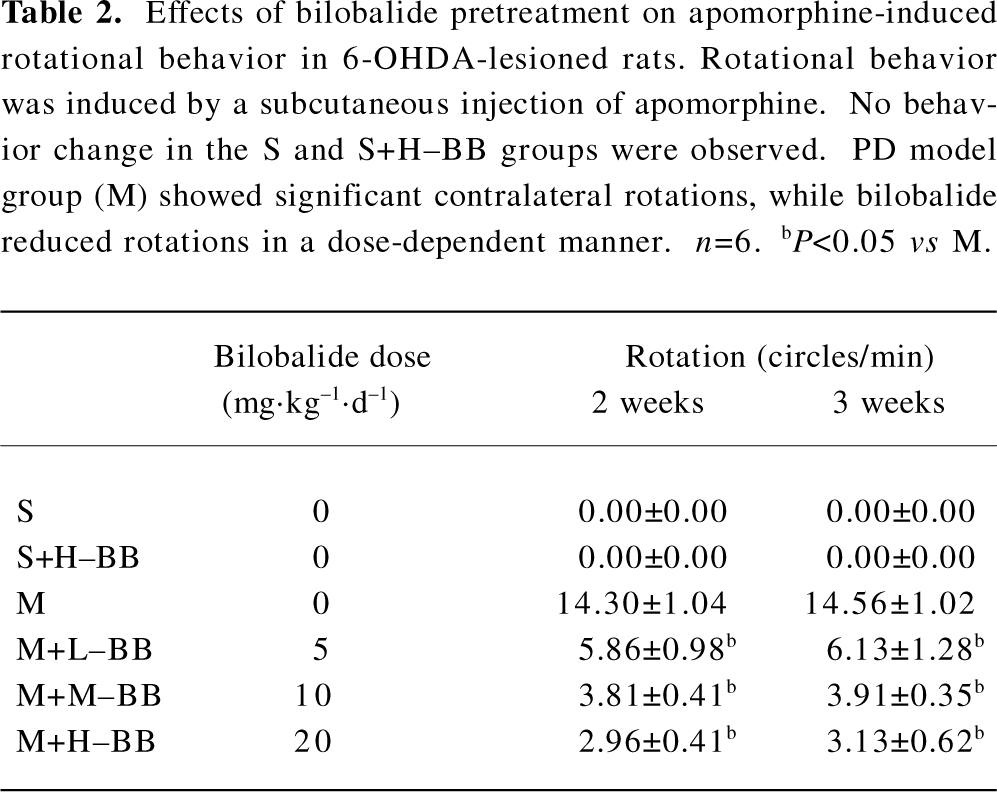
Full table
Bilobalide reduced 6-OHDA-induced loss of dopaminergic neurons The loss of dopaminergic neurons in the SNpc was examined with TH immunofluorescence and Nissl’s staining after 6-OHDA treatment. The infusion of 6-OHDA caused a rapid and consistent loss of TH immunoreactivity in the SNpc. By 24 d after 6-OHDA administration, a significant loss of TH immunoreactivity was observed. Pretreatment with bilobalide significantly reduced the 6-OHDA-induced loss of TH-positive neurons in the SNpc (Figure 1).
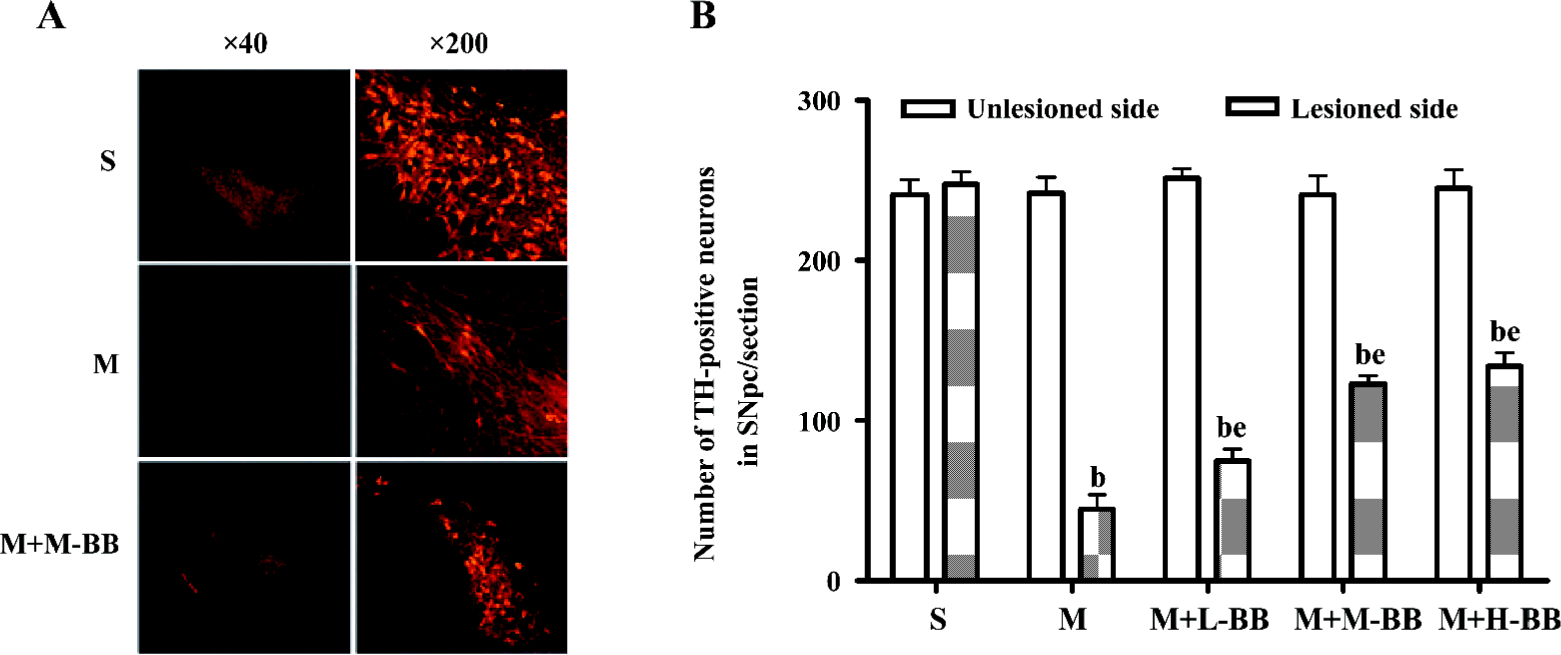
We extended our observations with Nissl’s staining 24 d after 6-OHDA treatment. The results showed a remarkable loss of Nissl’s body in the 6-OHDA-lesioned SNpc, and bilobalide substantially recovered the loss of nigral neurons (Figure 2).
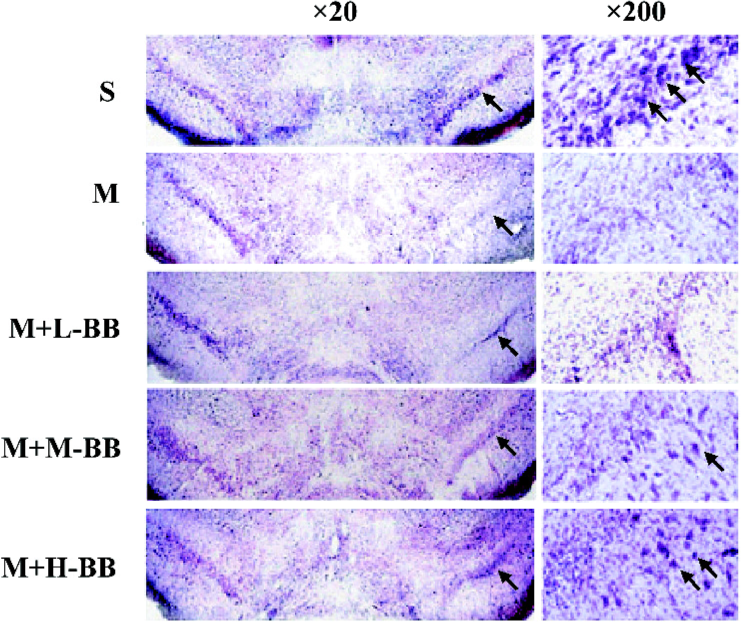
Bilobalide blocked 6-OHDA-induced activation of NF-κB NF-κB is known as an important transcriptional factor, playing a central role in the regulation of many immune and inflammatory responses, as well as the control of cell apoptosis. Recent evidence demonstrated the activation of NF-κB in neuronal cells during neurodegenerative processes.
The activation of NF-kB was examined in situ using immunofluorescence. The nuclear translocation of p65, a family member of NF-κB, was detected with immunofluorescence in dopaminergic neurons 24 h after 6-OHDA injection. The results showed that 6-OHDA induced a higher expression of NF-κB p65 in the nuclei of TH-positive neurons. Pretreatment with bilobalide at a dose of 10 mg or higher effectively blocked 6-OHDA-induced NF-κB p65 nuclear translocation as revealed by immunofluorescence (Figure 3A). A quantitative analysis showed that the percentages of NF-κB p65-positive dopaminergic neurons significantly decreased from 37.5%±2.6% to 18.6%±1.7% by pretreatment with bilobalide (P<0.05; Figure 3B).
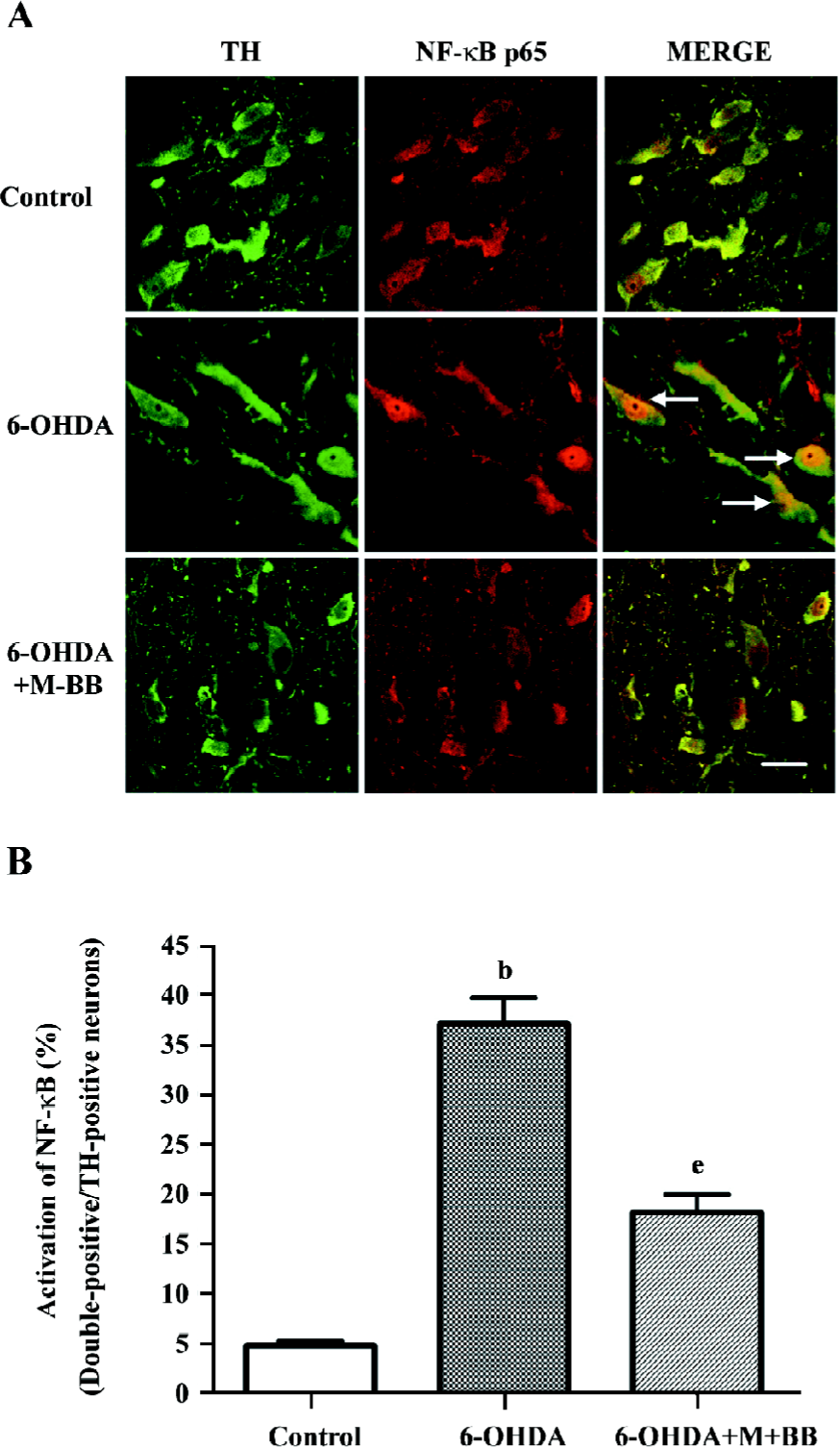
Bilobalide reduced the numbers of terminal deoxynucleotidyl transferase-mediated dUTP nick-end labeling-positive nuclei in the SNpc There were few terminal deoxynucleotidyl transferase-mediated dUTP nick-end labeling (TUNEL)-positive nuclei in the contralateral SN of PD model animals or in the ipsilateral SN of sham-operated animals. Increased numbers of TUNEL-positive nuclei were observed 24 h after 6-OHDA infusion, which revealed apoptosis in the SNpc (Figure 4A). A quantitative analysis showed that the percentages of apoptosis in TH-positive neurons increased from 2.8%±0.4% to 55.7%±1.9% (P<0.05; Figure 4B). We detected the co-expression of TUNEL and NF-κB p65 nuclear translocation by double immunofluorescence. Elevated NF-κB p65 nuclear translocation and TUNEL-positive nuclei were observed 24 h after 6-OHDA damage (Figure 5). TUNEL and p65 staining were colocalized in the nuclei of the SNpc neurons. We found that pretreatment with bilobalide produced a significant decrease of NF-κB p65 and TUNEL-positive nuclear staining.
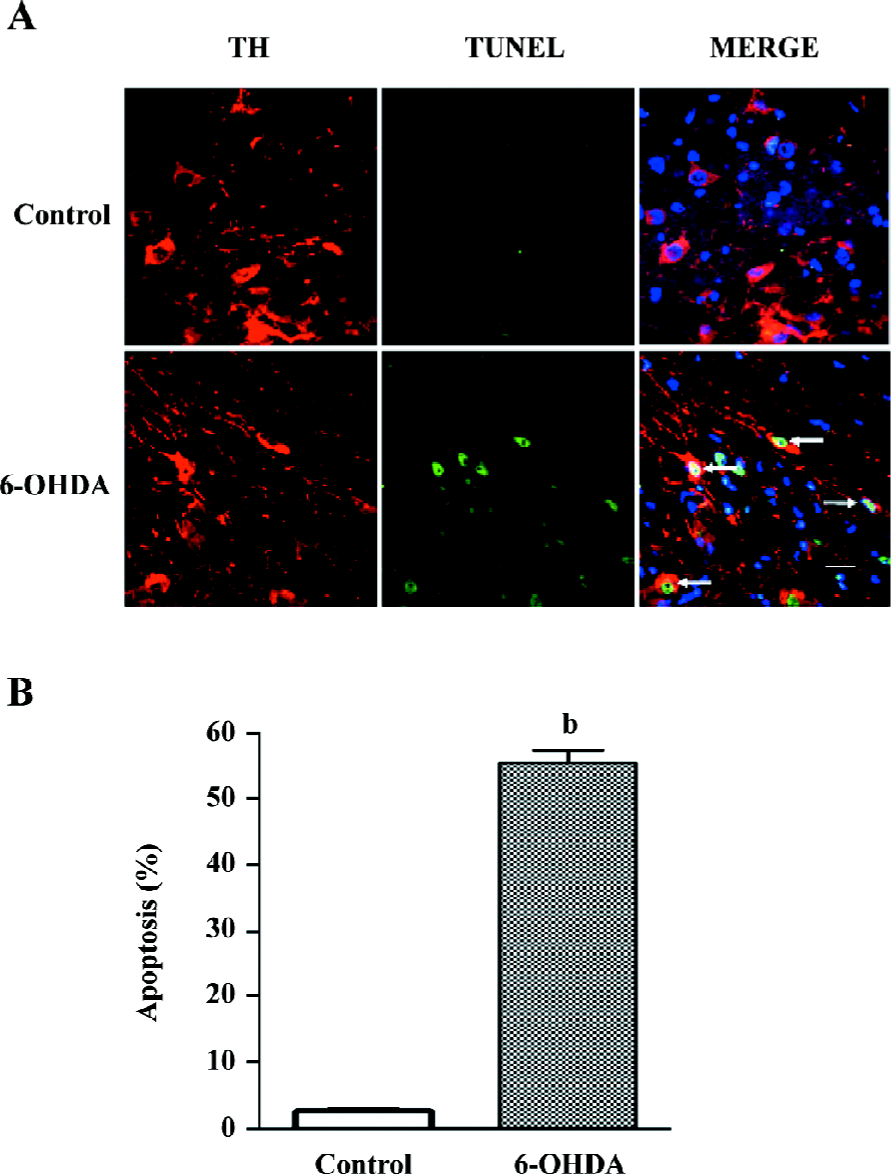
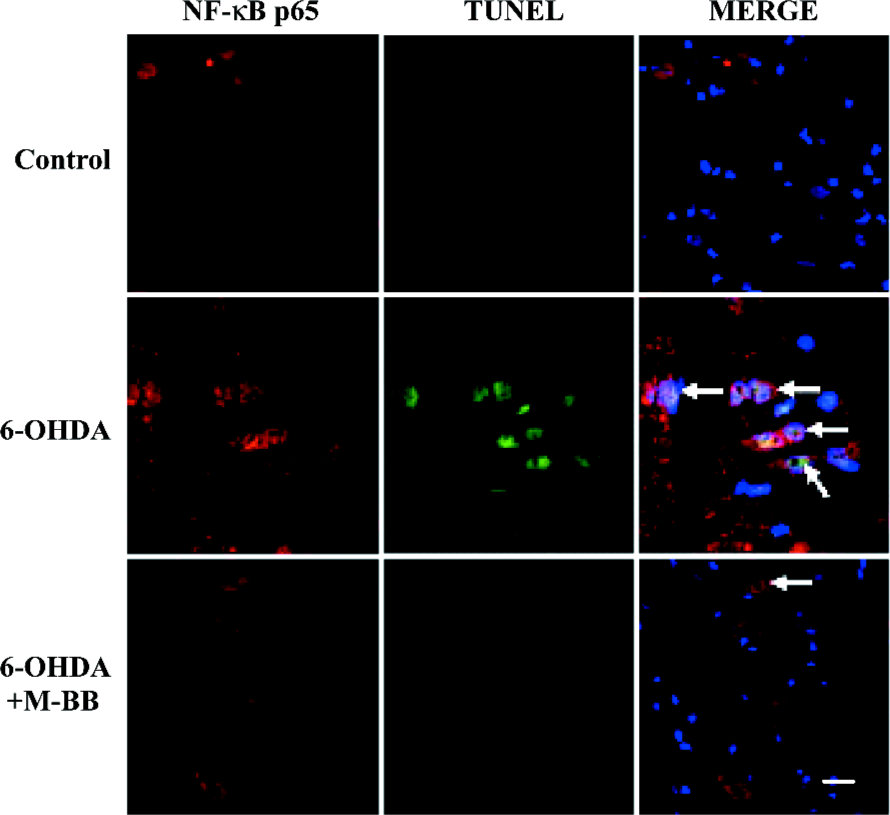
Discussion
A number of reports indicate that EGb has wide pharmacological actions, such as anti-anemia[29], anti-edema[30], anti-inflammation[31], anti-hypoxia[32] and anti-oxidative stress[33]. Wettstein et al[34] reported that EGb should be considered equally effective with second-generation cholinesterase inhibitors in the treatment of mild to moderate Alzheimer’s dementia. Chandrasekaran et al[22] reported that bilobalide exhibits protection against delayed ischemic neuronal death similar to that observed with the administration of ginkgo extracts. Consistent with this finding, Mdzinarishvili et al[35] also demonstrated that bilobalide can prevent ischemia-induced edema formation in vitro and in vivo. Bilobalide could be an important active constituent of the extract. We attempted to find out whether bilobalide is able to inhibit neuronal necrosis and apoptosis induced by 6-OHDA in the SNpc of rats. Our study demonstrates that bilobalide can restore the locomotion, inhibit rotational behavior, and reduce loss of dopaminergic neurons, suggesting that bilobalide has neuroprotective effects and is able to improve the pathological symptoms in PD model rats.
At present, the mechanisms by which bilobalide protects neurons remain to be determined. In vitro and in vivo studies indicate that bilobalide has multiple actions that may be associated with neuroprotection, including preservation of mitochondrial ATP synthesis[36,37], inhibition of apoptotic damage induced by staurosporine or by serum-free media[38], suppression of hypoxia-induced membrane deterioration in the brain[39], and regulation of mitochondrial gene expression[23]. Zhou and Zhu[25] demonstrated that bilobalide could attenuate ROS-induced apoptosis in PC12 cell lines, suggesting that bilobalide might be acting as a free-radical scavenger.
NF-κB appeared to be elevated in the SN of the post-mortem brains of PD cases[40,41]. The relationship of NF-κB activation to the disease process, however, is unclear. Several in vitro studies have reported the activation of NF-κB in response to 6-OHDA treatment[42]. The intrastriatal administration of dopamine also produced oxidative damage to striatal neurons and a robust activation of NF-κB[43], but evaluations of the role of NF-κB in in vitro PD models have not yielded consistent results[44,45]. Some studies reported that the neuroprotective effect offered by some pharmacological agents is associated with the blockade of NF-κB activation, suggesting that NF-κB plays a pro-apoptotic role in PD[46–48]. In our previous study, 6-OHDA induced an increase in the binding activity of NF-κB, which provided the first biochemical evidence of the activation of NF-κB in animal models of PD.
In this study, 6-OHDA-induced apoptosis of dopaminergic neurons was accompanied by NF-κB activation. It was implied that the activation of NF-κB p65 contributed to the apoptosis of nigral neurons. A decrease in total cell numbers and increase in apoptotic cells induced by 6-OHDA were significantly attenuated by bilobalide pretreatment. NF-κB activation and an increase in DNA fragmentation induced by 6-OHDA were also significantly inhibited. Bilobalide may block apoptosis of dopaminergic neurons through the suppression of the expression of the NF-κB p65 protein and decrease its nuclear translocation in the SNpc of rats.
In summary, the present study shows that apoptosis is involved in 6-OHDA-induced dopaminergic neuronal death, and that the activation of NF-κB plays an important role in apoptosis. Pretreatment with bilobalide effectively prevents the activation of NF-κB and produces a marked protective effect on dopaminergic neurons against the toxicity induced by 6-OHDA in the SNpc. Bilobalide may thereby provide a therapeutic approach to rescue the dopaminergic neurons in the process of PD.
Acknowledgment
Bilobalide was provided by Prof Zhong-liang CHEN from the Department of Phytochemistry, Shanghai Institute of Materia Medica, Chinese Academy of Sciences (Shanghai, China).
References
- Chinta SJ, Andersen JK. Dopaminergic neurons. Int J Biochem Cell Biol 2005;37:942-6.
- Ziv I, Barzilai A, Offen D, Nardi N, Melamed E. Nigrostriatal neuronal death in Parkinson’s disease––a passive or an active genetically-controlled process? J Neural Transm Suppl 1997;49:69-76.
- Barzilai A, Melamed E, Shirvan A. Is there a rationale for neuroprotection against dopamine toxicity in Parkinson’s disease? Cell Mol Neurobiol 2001;21:215-35.
- Qin ZH, Wang Y, Nakai M, Chase TN. Nuclear factor-κB contributes to excitotoxin-induced apoptosis in rat striatum. Mol Pharmacol 1998;53:33-42.
- Qin ZH, Chen RW, Wang Y, Nakai M, Chuang DM, Chase TN. Nuclear factor κB nuclear translocation upregulates c-Myc and p53 expression during NMDA receptor-mediated apoptosis in rat striatum. J Neurosci 1999;19:4023-33.
- Liang ZQ, Li YL, Zhao XL, Han R, Wang XX, Wang Y, et al. NF-κB contributes to 6-hydroxydopamine-induced apoptosis of nigral dopaminergic neurons through p53. Brain Res 2007;1145:190-203.
- Panet H, Barzilai A, Daily D, Melamed E, Offen D. Activation of nuclear transcription factor κB (NF-κB) is essential for dopamine-induced apoptosis in PC12 cells. J Neurochem 2001;77:391-8.
- DeFeudis FV, Drieu K. Ginkgo biloba extract (EGb 761) and CNS functions: basic studies and clinical applications. Current Drug Targets 2000;1:25-58.
- Ahlemeyer B, Krieglstein J. Neuroprotective effects of Ginkgo biloba extract. Cell Mol Life Sci 2003;60:1779-92.
- Ahlemeyer B, Krieglstein J. Pharmacological studies supporting the therapeutic use of Ginkgo biloba extract for Alzheimer’s disease. Pharmacopsychiatry 2003;36 Suppl 1:S8-14.
- Grundman M, Grundman M, Delaney P. Antioxidant strategies for Alzheimer’s disease. Proc Nutr Soc 2002;61:191-202.
- DeFeudis FV. extract (EGb761): Pharmacological activities and clinical applications. Paris: Elsevier; 1991.
- Zhang J, Fu S, Liu S, Mao T, Xiu R. The therapeutic effect of Ginkgo biloba extract in SHR rats and its possible mechanisms based on cerebral microvascular flow and vasomotion. Clin Hemorheol Microcirc 2000;23:133-8.
- Tchantchou F, Xu Y, Wu Y, Christen Y, Luo Y. E. Gb 761 enhances adult hippocampal neurogenesis and phosphorylation of CREB in transgenic mouse model of Alzheimer’s disease. FASEB J 2007;21:2400-8.
- Brunetti L, Orlando G, Menghini L, Ferrante C, Chiavaroli A. Ginkgo biloba leaf extract reverses amyloid beta-peptide-induced isoprostane production in rat brain in vitro. Planta Med 2006;72:1296-9.
- Pardon MC, Joubert C, Perez-Diaz F, Christen Y, Launay JM, Cohen-Salmon C. In vivo regulation of cerebral monoamine oxidase activity in senescent controls and chronically stressed mice by long-term treatment with Ginkgo biloba extract (EGb 761). Mech Ageing Dev 2000;113:157-68.
- Wu WR, Zhu XZ. Involvement of monoamine oxidase inhibition in neuroprotective and neurorestorative effects of Ginkgo biloba extract against MPTP-induced nigrostriatal dopaminergic toxicity in C57 mice. Life Sci 1999;65:157-64.
- Ahmad M, Saleem S, Ahmad AS, Yousuf S, Ansari MA, Khan MB, et al. Ginkgo biloba affords dose-dependent protection against 6-hydroxydopamine-induced parkinsonism in rats: neurobehavioural, neurochemical and immunohistochemical evidences. J Neurochem 2005;93:94-104.
- Cao F, Sun S, Tong ET. Experimental study on inhibition of neuronal toxical effect of levodopa by Ginkgo biloba extract on Parkinson’s disease in rats. J Huazhong Univ Sci Tech Med Sci 2003;23:151-3.
- Otani M, Chatterjee SS, Gabard B, Kreutzberg GW. Effect of an extract of Ginkgo biloba on triethyltin-induced cerebral edema. Acta Neuropathol 1986;69:54-65.
- Chandrasekaran K, Mehrabian Z, Spinnewyn B, Drieu K, Fiskum G. Neuroprotective effects of bilobalide, a component of the Ginkgo biloba extract (EGb 761), in gerbil global brain ischemia. Brain Res 2001;922:282-92.
- Chandrasekaran K, Mehrabian Z, Spinnewyn B, Chinopoulos C, Drieu K, Fiskum G. Bilobalide, a component of the Ginkgo biloba extract (EGb 761), protects against neuronal death in global brain ischemia and in glutamate-induced excitotoxicity. Cell Mol Biol 2002;48:663-9.
- Chandrasekaran K, Mehrabian Z, Spinnewyn B, Chinopoulos C, Drieu K, Fiskum G. Neuroprotective effects of bilobalide, a component of Ginkgo biloba extract (EGb 761) in global brain ischemia and in excitotoxicity-induced neuronal death. Pharmacopsychiatry 2003;36 Suppl 1:S89-94.
- Ahlemeyer B, Krieglstein J. Neuroprotective effects of Ginkgo biloba extract. Cell Mol Life Sci 2003;60:1779-92.
- Zhou LJ, Zhu XZ. Reactive oxygen species-induced apoptosis in PC12 cells and protective effect of bilobalide. J Pharmacol Exp Ther 2000;293:982-8.
- Weichel O, Hilgert M, Chatterjee SS, Lehr M, Klein J. Bilobalide, a constituent of Ginkgo biloba, inhibits NMDA-induced phospholipase A2 activation and phospholipid breakdown in rat hippocampus. Naunyn Schmiedebergs Arch Pharmacol 1999;360:609-15.
- Carman LS, Gage FH, Shults CW. Partial lesion of the substantia nigra: relation between extent of lesion and rotational behavior. Brain Res 1991;553:275-83.
- West MJ, Slomianka L, Gundersen HJ. Unbiased stereological estimation of the total number of neurons in the subdivisions of the rat hippocampus using the optical fractionator. Anat Rec 1991;231:482-97.
- Sarikcioglu SB, Oner G, Tercan E. Antioxidant effect of EGb 761 on hydrogen peroxide-induced lipoperoxidation of G-6-PD deficient erythrocytes. Phytother Res 2004;8:837-40.
- Biddlestone L, Corbett AD, Dolan S. Oral administration of Ginkgo biloba extract, EGb-761 inhibits thermal hyperalgesia in rodent models of inflammatory and post-surgical pain. Br J Pharmacol 2007;151:285-91.
- Han Y. Ginkgo terpene component has an anti-inflammatory effect on Candida albicans-caused arthritic inflammation. Int Immunopharmacol 2005;5:1049-56.
- Sharma HS, Drieu K, Westman J. Antioxidant compounds EGb-761 and BN-52021 attenuate brain edema formation and hemeoxygenase expression following hyperthermic brain injury in the rat. Acta Neurochir Suppl 2003;86:313-9.
- Smith JV, Luo Y. Elevation of oxidative free radicals in Alzheimer’s disease models can be attenuated by Ginkgo biloba extract EGb 761. J Alzheimers Dis 2003;5:287-300.
- Wettstein A. Cholinesterase inhibitors and Gingko extracts––are they comparable in the treatment of dementia? Comparison of published placebo-controlled efficacy studies of at least six months’ duration. Phytomedicine 2000;6:393-401.
- Mdzinarishvili A, Kiewert C, Kumar V, Hillert M, Klein J. Bilobalide prevents ischemia-induced edema formation in vitro and in vivo. Neuroscience 2007;144:217-22.
- Janssens D, Delaive E, Remacle J, Michiels C. Protection by bilobalide of the ischaemia-induced alterations of the mitochondrial respiratory activity. Fundam Clin Pharmacol 2000;14:193-201.
- Bouaziz N, Redon M, Quere L, Remacle J, Michiels C. Mitochondrial respiratory chain as a new target for anti-ischemic molecules. Eur J Pharmacol 2002;441:35-45.
- Ahlemeyer B, Möwes A, Krieglstein J. Inhibition of serum deprivation- and staurosporine-induced neuronal apoptosis by Ginkgo biloba extract and some of its constituents. Eur J Pharmacol 1999;367:423-30.
- Klein J, Chatterjee SS, Loffelholz K. Phospholipid breakdown and choline release under hypoxic conditions: inhibition by bilobalide, a constituent of Ginkgo biloba. Brain Res 1997;755:347-50.
- Hunot S, Brugg B, Ricard D, Michel PP, Muriel MP, Ruberg M, et al. Nuclear translocation of NF-κB is increased in dopaminergic neurons of patients with Parkinson disease. Proc Natl Acad Sci USA 1997;94:7531-6.
- Soos J, Engelhardt JI, Siklos L, Havas L, Majtenyi K. The expression of PARP, NF-κB and parvalbumin is increased in Parkinson’s disease. Neuroreport 2004;15:1715-8.
- Tarabin V, Schwaninger M. The role of NF-κB in 6-hydroxydopamine- and TNFα-induced apoptosis of PC12 cells. Naunyn Schmiedebergs Arch Pharmacol 2004;369:563-9.
- Lou Y, Hattori A, Munoz J, Qin ZH, Roth GS. Intrastriatal dopamine injection induces apoptosis through oxidation-involved activation of transcription factors AP-1 and NF-κB in rats. Mol Pharmacol 1999;56:254-64.
- Panet H, Barzilai A, Daily D, Melamed E, Offen D. Activation of nuclear transcription factor κB (NF-κB) is essential for dopamine-induced apoptosis in PC12 cells. J Neurochem 2001;77:391-8.
- Park SH, Choi WS, Yoon SY, Ahn YS, Oh YJ. Activation of NF-κB is involved in 6-hydroxydopamine- but not MPP+-induced dopaminergic neuronal cell death: its potential role as a survival determinant. Biochem Biophys Res Commun 2004;322:727-33.
- Dehmer T, Heneka MT, Sastre M, Dichgans J. Protection by pioglitazone in the model of Parkinson’s disease correlates with IκBα induction and block of NF-κB and iNOS activation. J Neurochem 2004;88:494-501.
- Levites Y, Youdim BH, Maor G, Mandel S. Attenuation of 6-hydroxydopamine (6-OHDA)-induced nuclear factor-kappaB (NF-κB) activation and cell death by tea extracts in neuronal cultures. Biochem Pharmacol 2002;63:21-9.
- Youdim MB, Grunblatt E, Mandel S. The pivotal role of iron in NF-κB activation and nigrostriatal dopaminergic neurodegeneration. Prospects for neuroprotection in Parkinson’s disease with iron chelators. Ann N Y Acad Sci 1999;890:7-25.