Effects of Ginkgo biloba on prevention of development of experimental diabetic nephropathy in rats1
Introduction
In many countries diabetic nephropathy (DN) is a major complication of diabetes. At present, it affects about 15%–25% of type 1 and 30%–40% of type 2 diabetic patients, causing disabilities and a high mortality rate. It is characterized by the thickening of the basement membranes, mesangial expansion and proliferation, and excessive accumulation of extracellular matrix (ECM), and ultimately leads to nodular glomerulosclerosis and chronic renal failure.
However, the mechanisms underlying the pathogenesis of DN are not completely understood. Many scholars consider the progression of DN a result of the interaction of multiple factors, such as high glucose, the polyol pathway, oxidative stress, protein non-enzymatic glycation, and cytokines[1]. Studies have found that high glucose is presumed to be an initiating agent which increases the formation of advanced glycosylation end products (AGE) and induces oxidative stress. It also increases transforming growth factor-β1 (TGF-β1) expressions. TGF-β1 is thought to be the key cytokine involved in the progression of DN[2]. It increases the synthesis of ECM components, including collagens, fibronectin, and laminin, and results in hypertrophy of the mesangial cell and glomerulosclerosis. Connective tissue growth factor (CTGF) has been described as a growth factor that acts downstream of TGF-β1 and is a potent inducer of ECM in the fibrotic process[3,4]. Matrix metalloproteinases (MMP) are the major physiological regulator of ECM degradation in the glomerulus. A balance between ECM synthesis and degradation is a prerequisite for maintaining the structural and functional integrity of the glomerulus. MMP and tissue inhibitors of metalloproteinase (TIMP) keep this balance together[5]. For the complexity of mechanisms, there are no definitive drugs to delay the development of DN. Therefore, it is necessary to develop new drugs for DN that can deal with more than 1 pharmacological target in this intricate mechanism.
The tree Ginkgo biloba has long been believed to have medicinal properties, and its extracts are among the most widely-sold herbal supplements in the world. Ginkgo biloba extract (GbE), extracted from Ginkgo biloba leaves, is a defined, complex mixture containing 24% Ginkgo flavone glycoside (quercetin, kaempferol, and isorhamne) and 6% terpene lactones (ginkgolides and bilobalide). It has been used as a therapeutic agent in some cardiovascular and neurological disorders[6,7]. Although the exact mechanism is unknown, evidence accumulated in vitro and in vivo shows that GbE has a number of benefits, including ameliorating hemodynamics, suppressing the platelet-activating factor, scavenging reactive oxygen species (ROS), and relaxing vascular smooth muscles[8]. All of these offer us a pharmacological foundation of GbE for DN therapy. However, there are still few published reports that focus on the protective mechanisms of GbE on DN. Therefore, it is worthwhile for us to explore its potential effects in preventing the progression of DN.
To evaluate the effects of GbE on DN, in our present work we used captopril (CAP) as an antifibrotic control drug[9,10], and studied the possible influences of GbE on the parameters that indicate protective effects against the progress of DN, such as blood glucose, AGE, TGF-β1, MMP-2, TIMP-2, CTGF, collagen IV and laminin in the kidney cortex, anti-oxidases in the serum, and the thickness of the glomerular base membrane (GBM) on early experimental DN rats, and observed the morphological changes on DN rats.
Materials and methods
Drugs GbE (Lot N
Animals Male Sprague-Dawley rats (Certificate N
Induction of DN model and study protocol Diabetes mellitus was induced in the male Sprague–Dawley rats, by ip injection of 60 mg/kg of the beta-cell toxin STZ (dissolved in pH 4.5 citrate buffer immediately before the injection), while controlled normal standard rats (NS group, n=13) received 6 mL/kg citrate buffer. The induction of the diabetic state was confirmed by the blood glucose level measurement on the third day after STZ administration. The rats with fasting blood glucose concentrations=13.88 mmol/L were randomly allotted into 5 groups: DN rats were treated with 1% CMC solution (DN group, n=13); DN rats with 50, 100, and 200 mg/kg GbE for the GL group (low dose, n=12), the GM group (moderate dose, n=14), and the GH group (high dose, n=14), respectively; the DN rats were treated with 10 mg/kg of captopril (CAP group, n=11). The same volume of CMC solution was administered to the NS group (n=13). The animals housed in the barrier environment refer to breed specific pathogen-free grade animals, and they were allowed food and water ad libitum. After 12 weeks, the urine and blood samples were collected. After the animals were sacrificed, fresh kidney cortices were stored in formaldehyde solution for immunohistochemical measurements, and 1 mm×1 mm×1 mm cubes of kidney cortices were fixed in 2.5% glutaraldehyde for electron microscopic measurement. The rest of the kidneys were stored at –80 oC for the later analysis.
Measurement of renal function and biochemical parameters Blood glucose was measured by the glucose oxidase method with kits purchased from Dong-Ou Bioengineering (N
Collagen IV and laminin, the main component of ECM, were measured by radioimmunoassay kits from Shanghai High Biotech Center (Lot N
Total antioxidative capability (T-AOC), catalase (CAT), total superoxidase dismutase (T-SOD) and glutathione-peroxidase (GSH-Px) activities in the serum were measured by spectrophotometry, using kits from Jiancheng Bioengineering Institute (Lot N
AGE plays a critical role in diabetic nephropathy by stimulating ECM synthesis. AGE either in the renal cortex or in the serum was measured by fluorescence spectrophotometry (fluorospectrophotometer F-4500, Hitachi, Tokyo, Japan), and the concentration of AGE was represented by the fluorescence optical density. The final value of AGE in the tissue was modulated by the total protein in the tissue which was measured by the Lowry method[11].
Immunohistochemical measurements of MMP-2, TIMP-2, and CTGF The glass slides were sealed with 10% polylysine. The 4 µm renal tissue sections were used to perform immunohistochemical staining for MMP-2, TIMP-2, and CTGF. The renal tissue sections were incubated with rabbit polyclonal anti-MMP-2 (Lot N
RT-PCR for the relative quantities of TGF-β1 mRNA in the kidney cortex[12] A RT-PCR procedure was performed to determine the relative quantities of TGF-β1 mRNA in the kidney cortex, while β-actin mRNA, a housekeeping gene, was used as an internal control. The total RNA was extracted from the kidney cortex with the Promega Total RNA Isolation System (Lot N
Morphological observation and measurement of the thickness of GBM The kidney cortex samples stored in formaldehyde solution were embedded with paraffin and stained with periodic acid-Schiff (PAS). Every PAS-stained sample in each group was observed under light microscope. Three kidney samples from each experimental group were randomly chosen for electron microscopic observation. The specimens were embedded in epoxy resin and cut into ultra-thin sections and then stained with plumbum citrate for ultrastructural observation under a transmission electron microscope (H600A-2, Hitachi, Japan). Five photos were taken at different views for each kidney sample. The images were amplified 6 K and the photos were scanned into a computer so that the thickness of GBM was measured by an image analysis system (Leica Qwin Standard V2.6, Leica Microsystems, Germany).
Statistical analysis Statistical analysis was performed to compare the effects of GbE on early DN rats using ANOVA and Dunnett’s t-test (2-side) for different groups using SPSS 10.0 (Chicago, USA). Data were expressed as mean±SD. Differences were considered to be significant at P<0.05.
Results
Effects of GbE on physical behaviors, blood glucose, urinary protein, Cr, BUN, and the kidney index In our experiment, the rats in the DN group had hypopraxia, cachexia, polyuria/polydipsia, yellowish and damp fur, kyphosis, and tardy weight gain; the rats in the NS and GH groups were vibrant and vigorous, had white and tidy fur, and weight gain.
Table 1 shows that the fasting blood glucose level, urine protein, Cr, BUN, and the kidney index of the DN group were significantly higher than those of the NS group (P<0.01), suggesting that our early DN model was successful. Low doses of GbE markedly reduced blood glucose and Cr in the DN rats (P<0.05), but was of no significant difference in reducing BUN, urine protein, and the kidney index. Moderate and high doses of GbE and captopril significantly reduced blood glucose, urine protein, Cr, BUN, and kidney index levels in the DN rats (P<0.01).
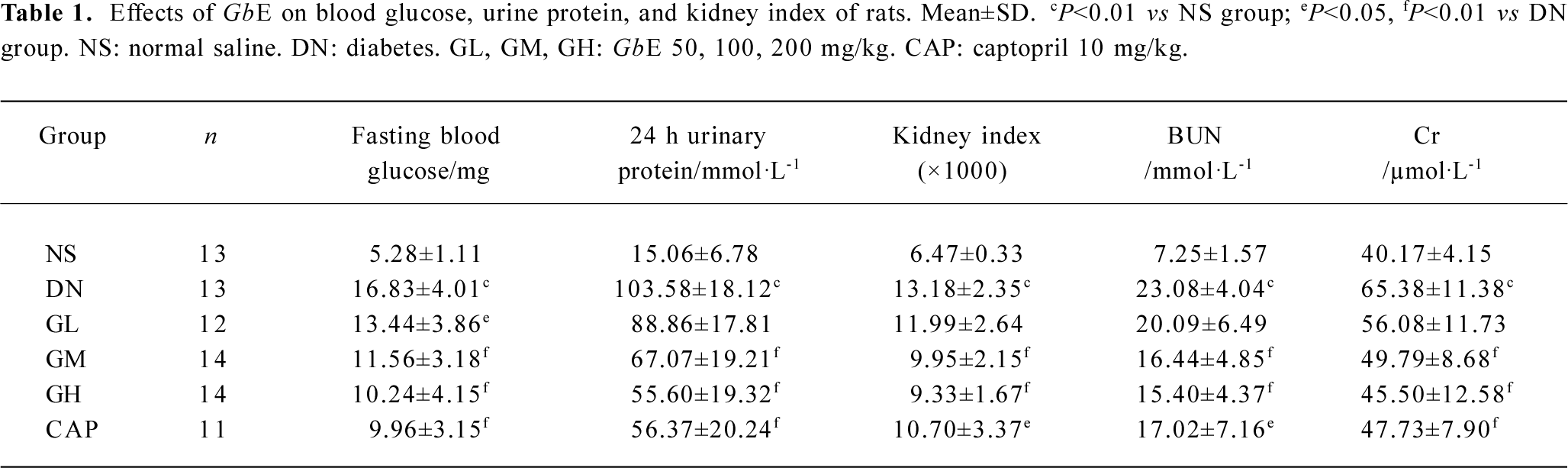
Full table
Effects of GbE on oxidative stress The activities of the CAT, GSH-Px, T-AOC, and T-SOD of the DN group were all lower than those of the NS group (P<0.01), suggesting that the DN group exhibited oxidative stress. We also found that low, moderate, and high doses of GbE increased these 4 anti-oxidase activities (P<0.05 or P<0.01). These results indicated that GbE could ameliorate the oxidative stress state of DN rats. Captopril also significantly increased CAT and GSH-Px activities (P<0.05), but it had no evident effect on T-AOC and T-SOD (P>0.05; Figure 1).
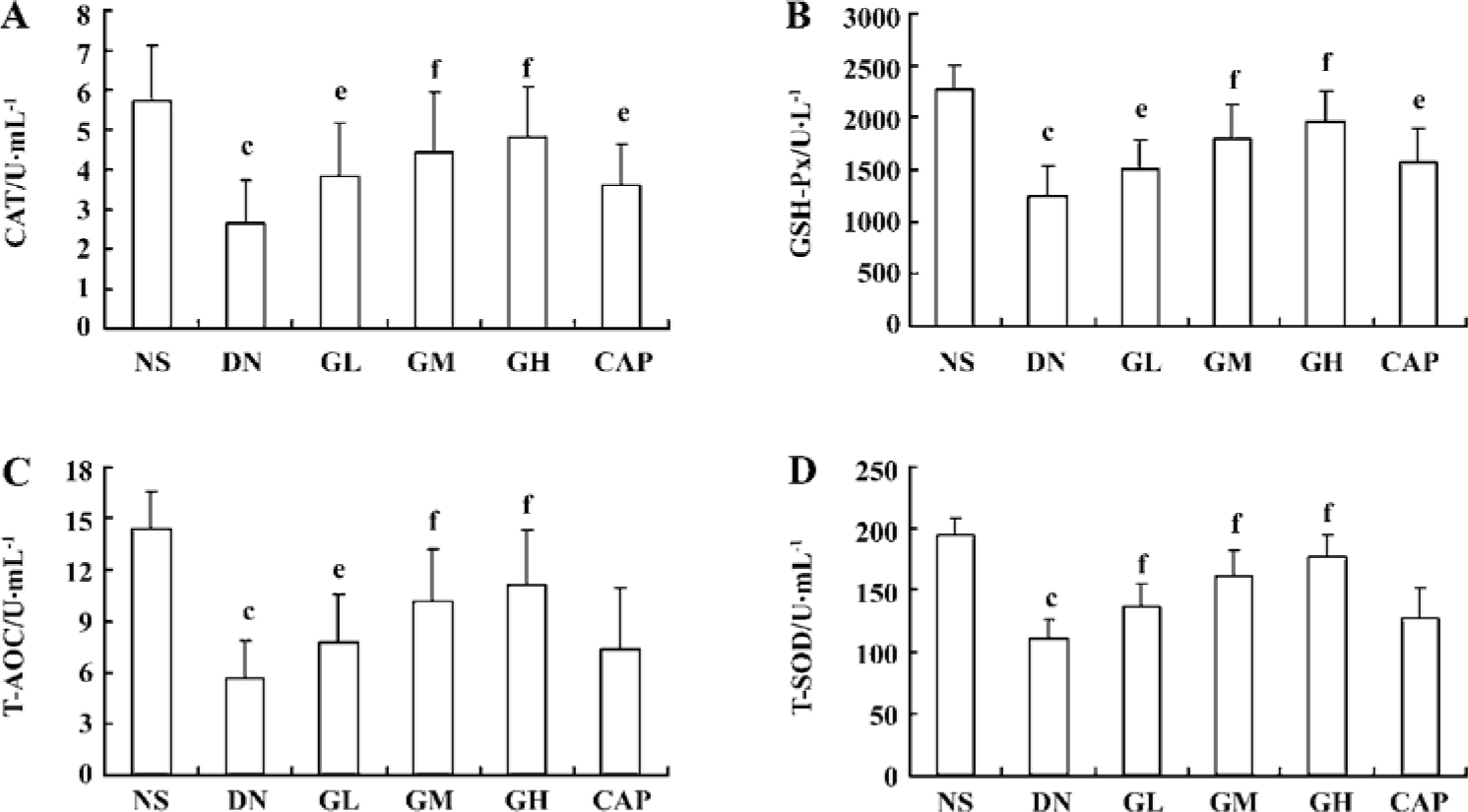
Effects of GbE on collagen IV and laminin level in the kidney cortex The levels of collagen IV and laminin in the kidney cortex of early DN rats significantly increased when compared with those of the normal rats (P<0.01). The collagen IV levels of the GM, GH, and CAP groups were strikingly lower than those of the DN group (P<0.05 or P<0.01). The laminin levels of the GL, GM, GH, and all the CAP groups decreased (P<0.01). These results suggested that GbE could decrease the expressions of collagen IV and laminin of DN rats, and that captopril’s capability of decreasing expressions of collagen IV was between that of GbE’s moderate and low dose (Figure 2).
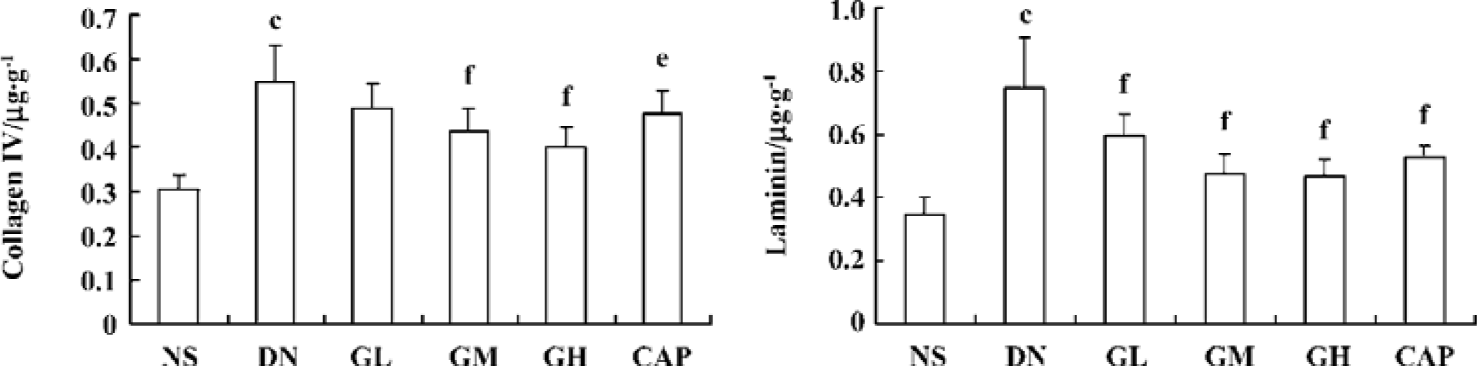
Effects of GbE on AGE and the thickness of GBM The AGE levels in the kidney cortex and in the serum of the DN rats were greatly higher than those of normal rats (P<0.01). In the kidney cortex and in the serum, there was significant decrease in the AGE levels of the GL, GM, and GH groups when compared with those of the DN group (P<0.05 or P<0.01), whereas captopril significantly decreased the AGE levels (P<0.01).
There was a significant difference in the thickness of the GBM between the NS group and DN group (P<0.01). The thickness of GBM decreased as the doses of GbE increased. There was significant decrease in the thickness of the GBM of the GL, GM, GH, and CAP groups, compared with that of the DN group (P<0.05 or P<0.01; Table 2).
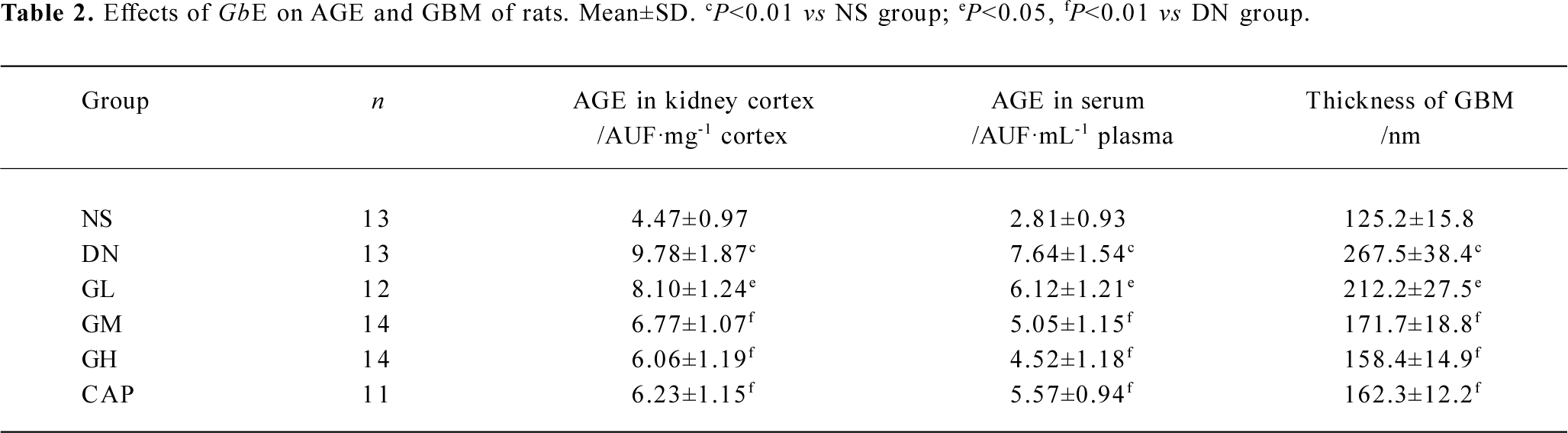
Full table
Immunocytochemical analysis of MMP-2, TIMP-2, and CTGF MMP-2 and TIMP-2 are mainly expressed in the cytoplasm of mesangial cells and renal tubular epithelial cells. The color of the stained MMP-2 and TIMP-2 protein was brown. The staining intensity of TIMP-2 of the DN group highly increased. On the contrary, MMP-2 markedly decreased when compared with those of the NS group. We used gray scale analysis to quantify MMP-2 and TIMP-2 proteins and found that the levels of MMP-2/TIMP-2 of the DN group was significantly different from that of the NS group (P<0.01). With the increased concentration of GbE, the expressions of MMP-2/TIMP-2 in the GM group and the GH group significantly increased/decreased (P<0.05 or P<0.01), respectively. The expressions in the CAP group had the same changes (P<0.01), and the levels of MMP-2 and TIMP-2 in the CAP group was between those of the GH group and GM group (Figures 3,4). Like TIMP-2, the levels of CTGF in the GL, GM, GH, CAP groups significantly decreased (P<0.01 or P<0.05; Figure 5). All of these results suggested that GbE had a potent influence on the expressions of MMP-2, TIMP-2, and CTGF (Figure 6).
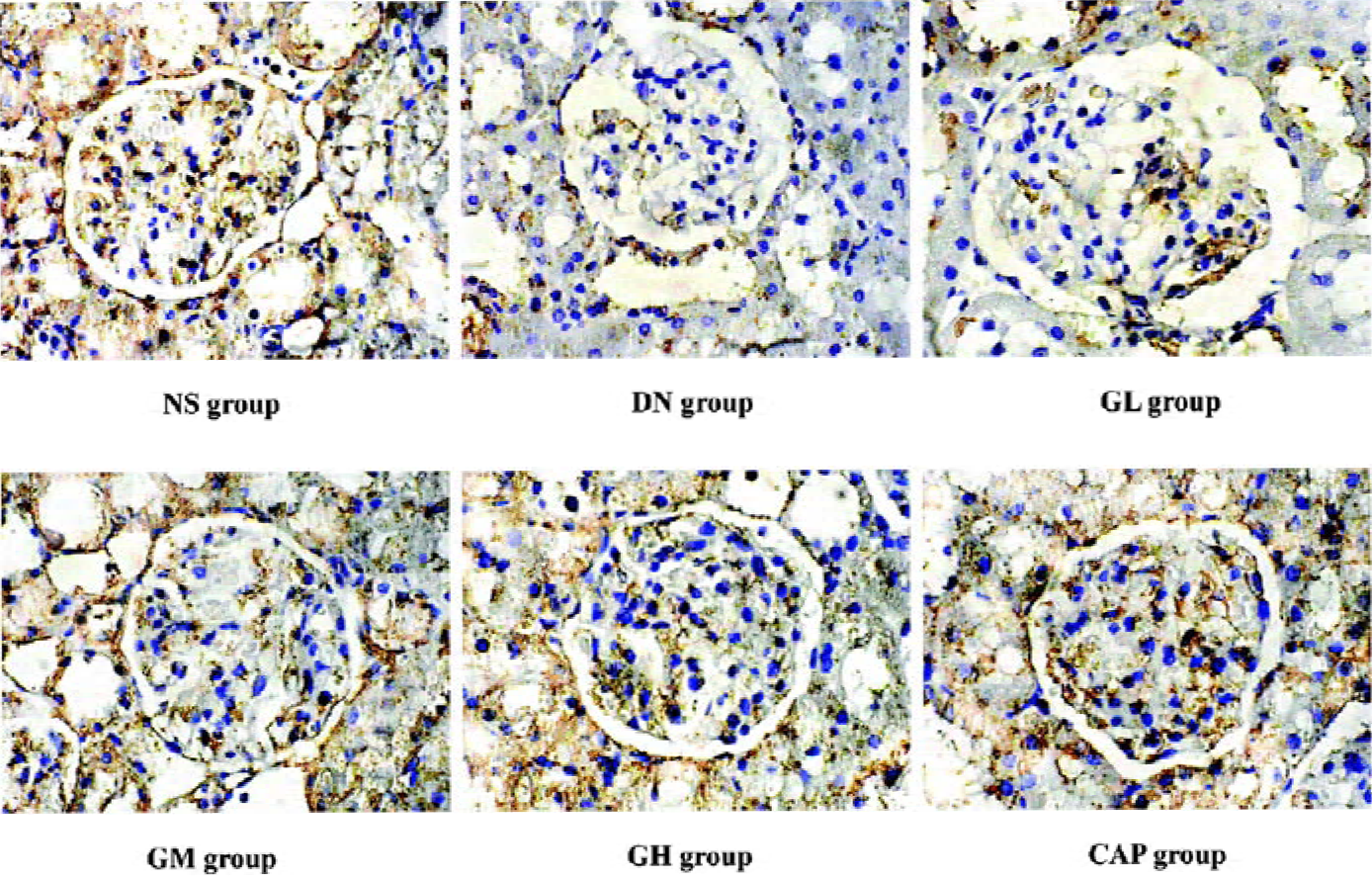
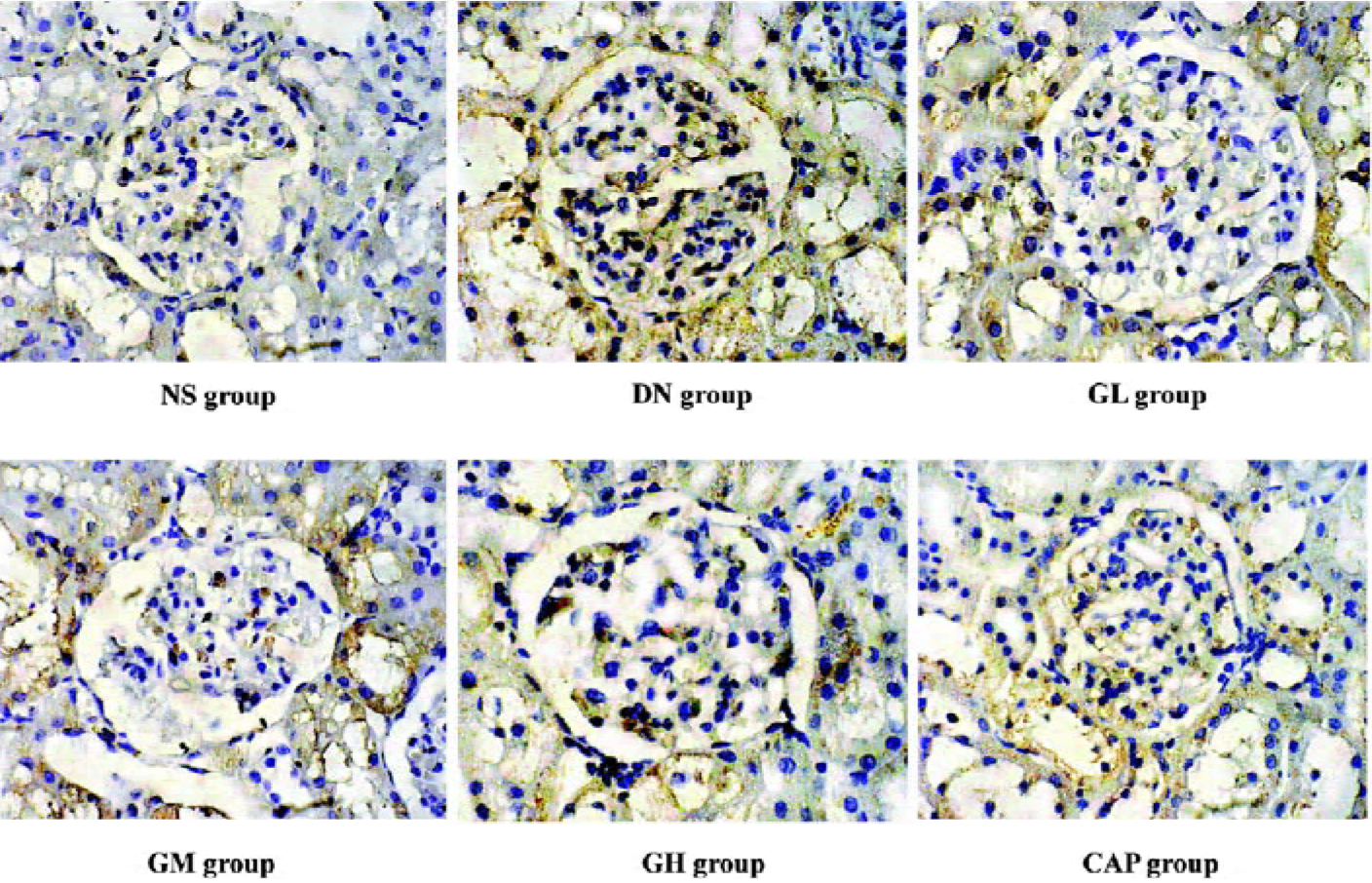
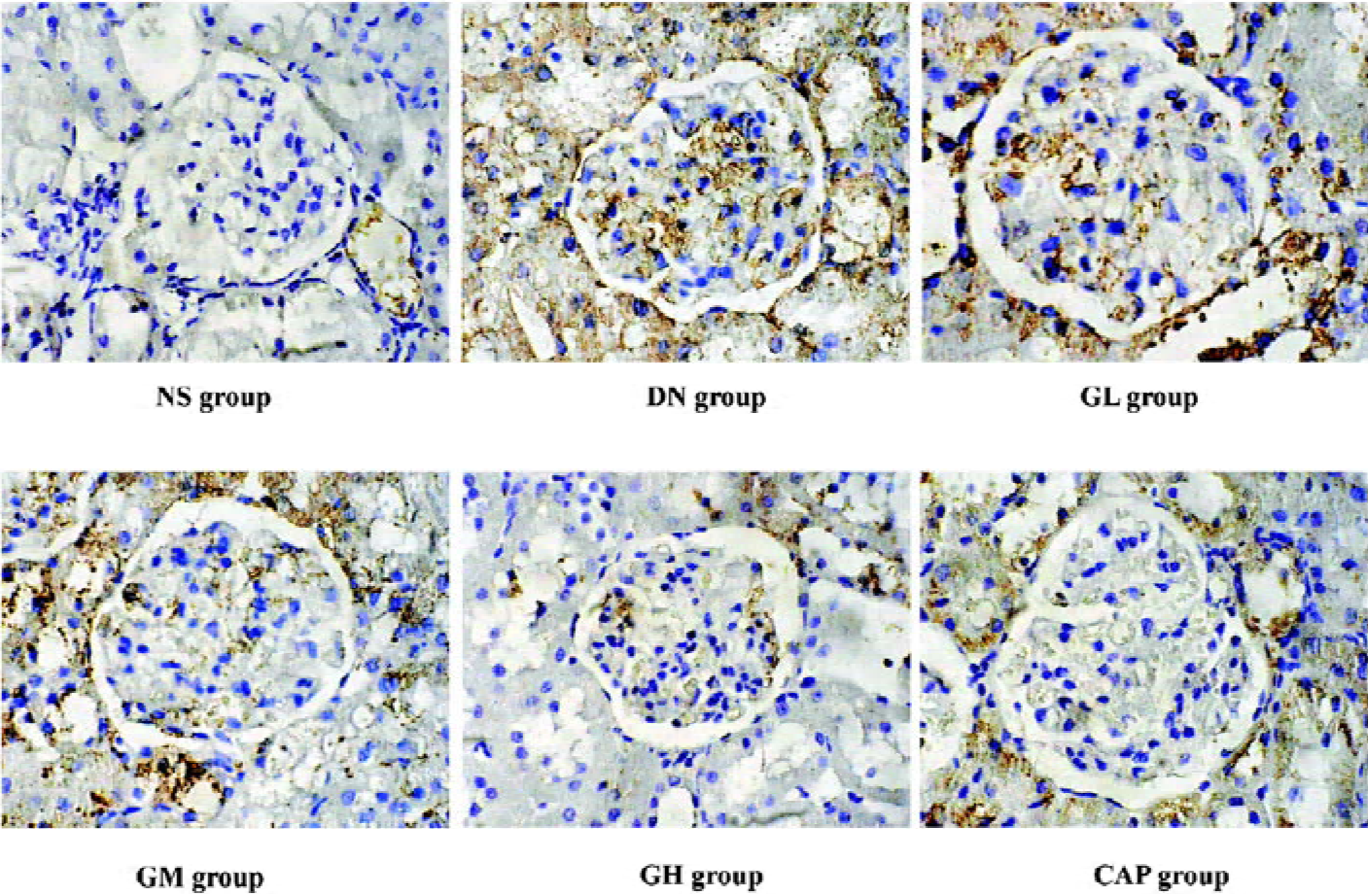
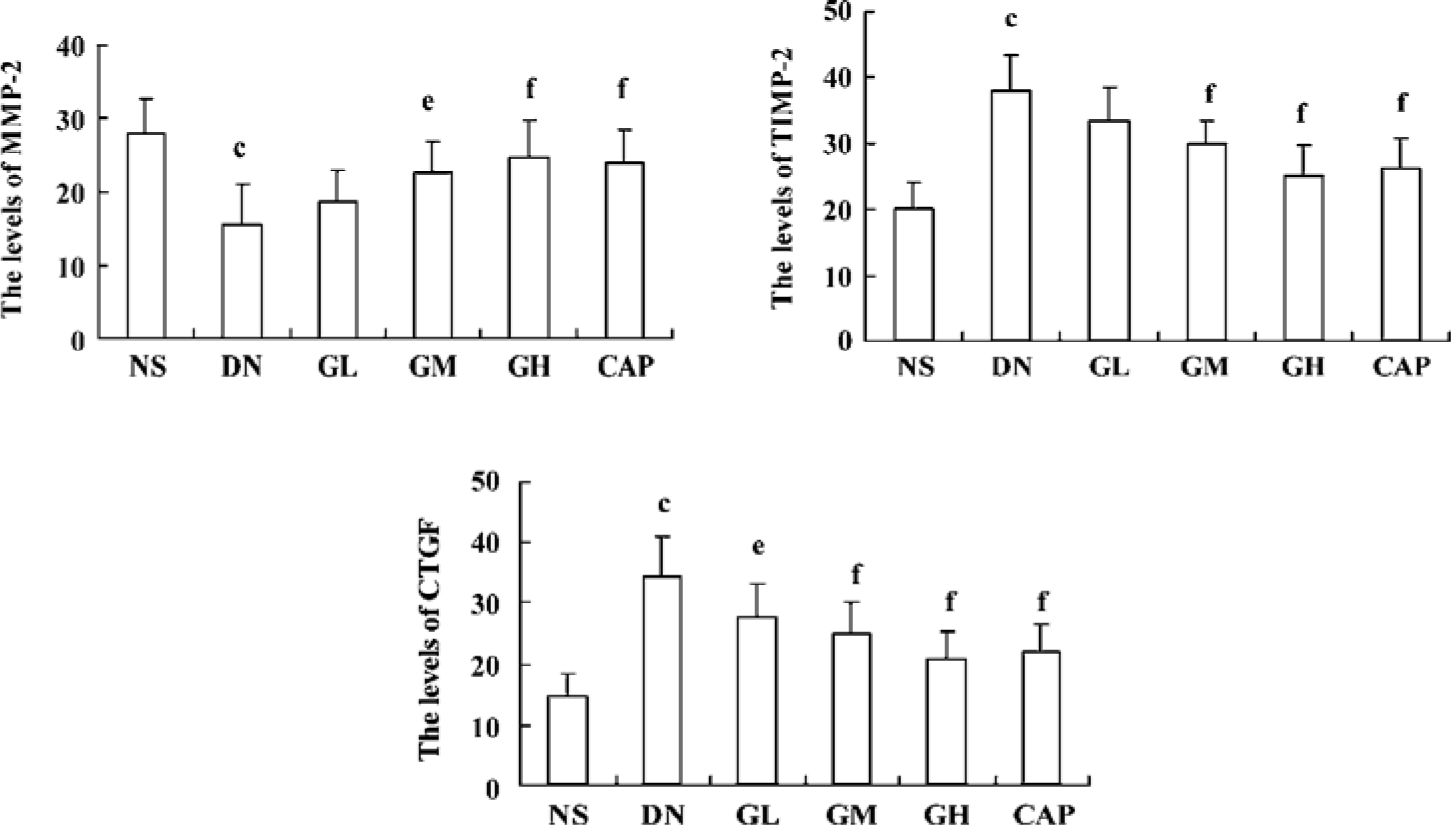
Effect of GbE on the relative quantity of TGF-β1 mRNA in the kidney cortex The RT-PCR products of TGF-β1 were separated by 1% agarose electrophoresis, after which we could see distinct bands (Figure 7A). The relative quantity of TGF-β1 mRNA in the kidney cortex of the DN group was greatly higher than that of the NS group (P<0.01). The TGF-β1 mRNA level of the GM, GH, and CAP groups strikingly decreased when compared with that of the DN group (P<0.05 or P<0.01). The level of the GH group was similar to that of the CAP group. The results suggested that a high dose of GbE had the same effect as captopril in decreasing the expression of TGF-β1 mRNA (Figure 7B).
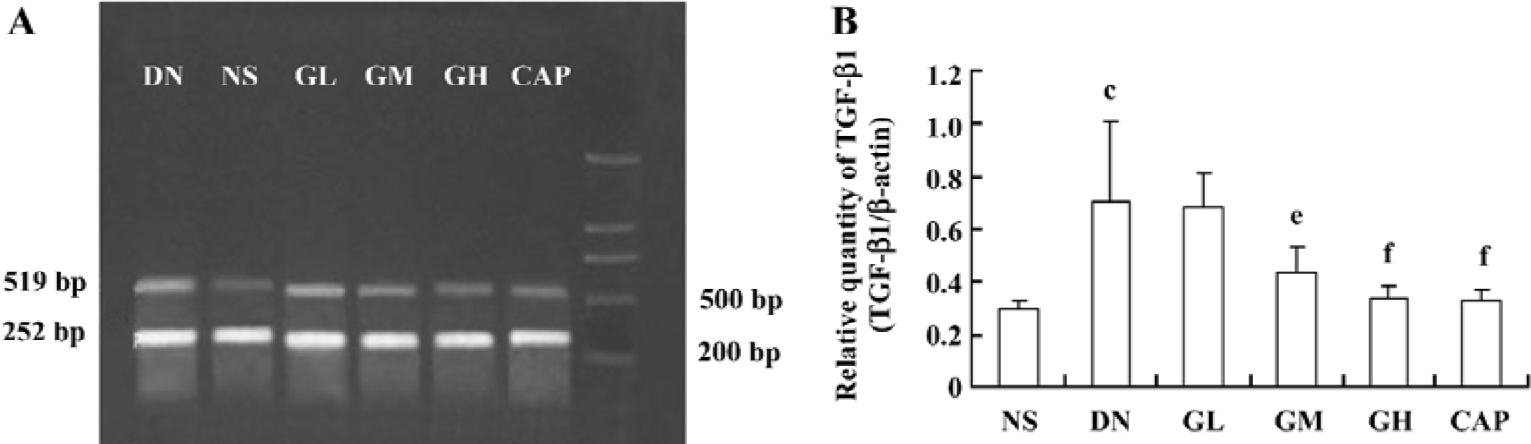
Effects of GbE on morphological change in kidneys The light microphotograph showed the existence of glomerular mesangial hyperplasia (Figure 8). In the transmission electron micrographs, the ultrastructure of glomerulus of the DN rat was changed. The GBM was wrinkled and partly thicken-ed, but in the GH group, the thickness of the GBM appeared to be almost normal (Figure 9).
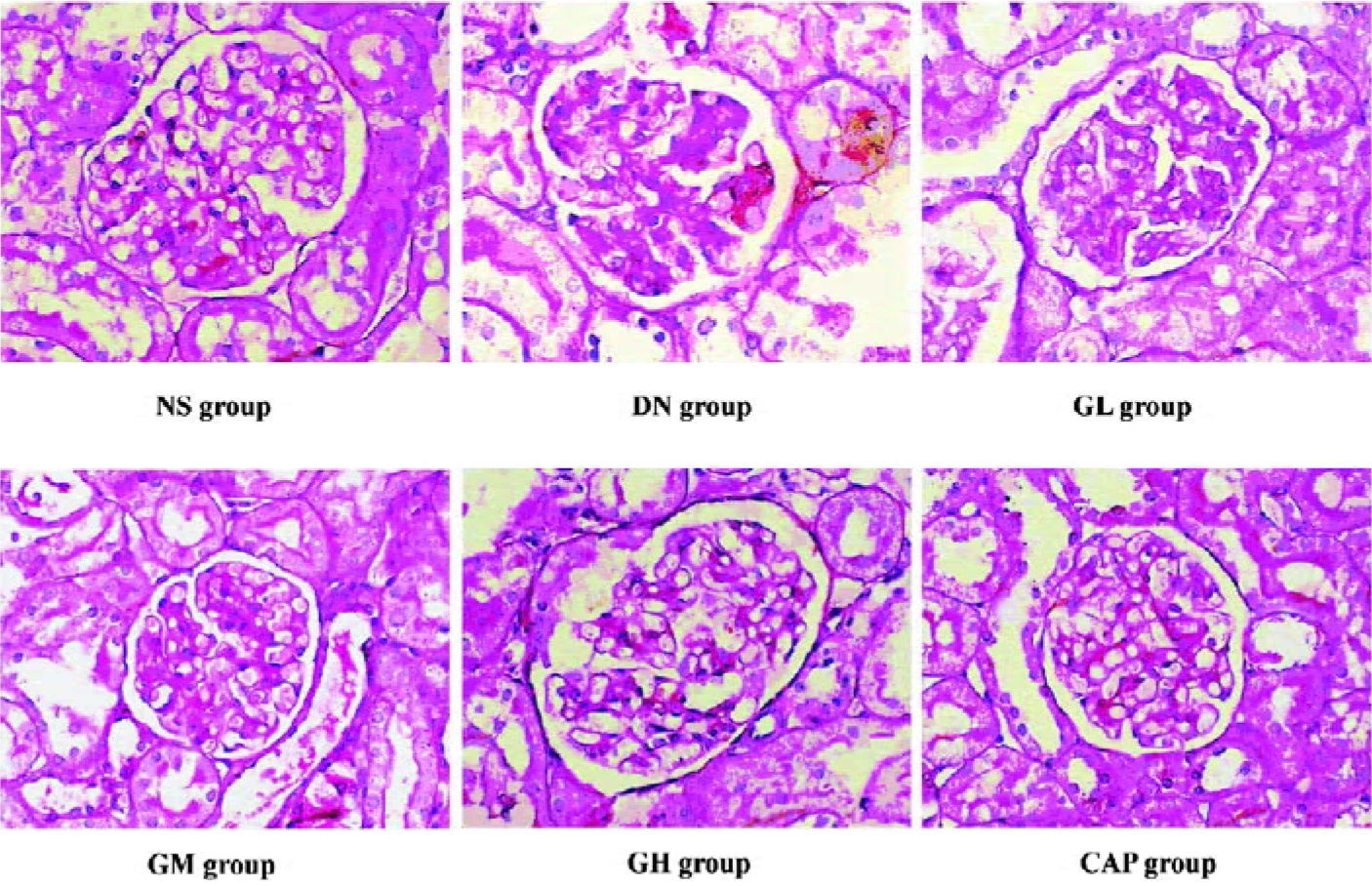
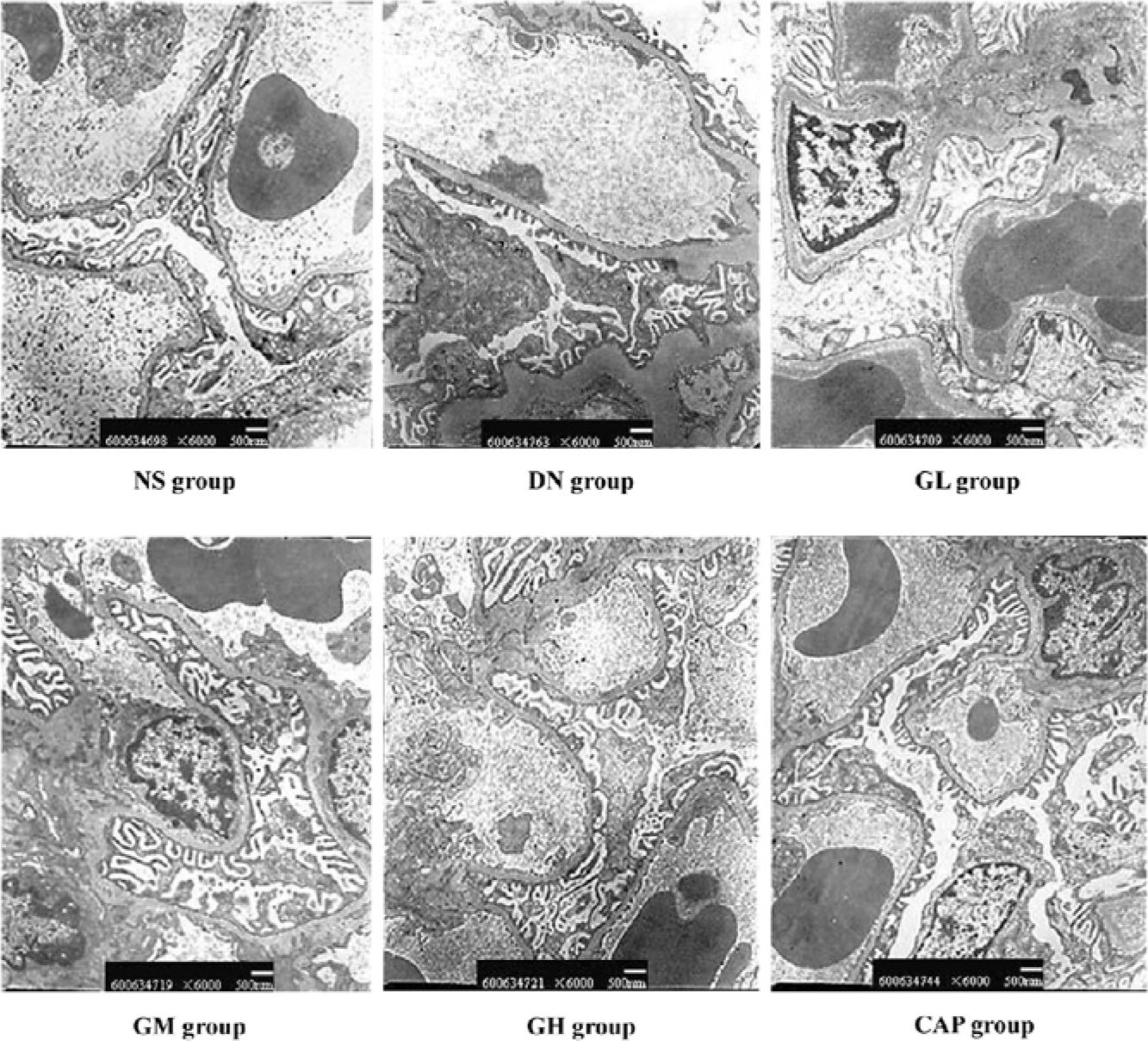
Discussion
DN is the leading cause of end-stage renal disease and the characteristics of this diabetic complication include macrovascular and microvascular damage, ECM accumu-lation, and eventually chronic fibrosis. Several decades of extensive research has elucidated various pathways implicated in the development of diabetic kidney disease.
Oxidative stress has been known to play an important role in the development and progression of DN, and the formation of ROS is a direct consequence of hyperglycemia. It has been shown that ROS activate the protein kinase C (PKC), mitogen-activated protein kinase (MAPK), and JAK-STAT pathways[13,14], which lead to the activation of redox-sensitive transcription factors including NF-κB, AP-1 (Fos and Jun proteins), STAT, and Egr-1[15]. All of these enhance the transactivation of genes coding for cytokines such as TGF-β1 and CTGF, which upregulate ECM protein expression[16]. Therefore, antioxidant treatment is a potential antifibrotic therapy for DN. Moreover, the intensity and durability of oxidative stress facilitate the formation of AGE. AGE are the biochemical end products of non-enzymatic glycosylation that are formed irreversibly. AGE are elevated in serum and in many tissues in patients with diabetes[17,18]. They can covalently crosslink and biochemically modify protein structure and affect protein functions, particularly collagen. Additionally, in recent years, cell surface receptors for AGE (RAGE) have been identified[19], and post-receptor signaling pathways are being defined[20]. Through an AGE receptor-dependent mechanism, AGE induction of cytokines and growth factors has been implicated in contributing to end-organ changes that occur in tissues of patients with diabetes[21]. At the same time, the interactions between AGE and RAGE induce the activation of oxidative stress and stimulate the production and release of cytokines, which amplify tissue damage. Thus, oxidative stress and AGE interact mutually and upregulate each other, which can lead to ECM accumulation and mesangial cell hypertrophy. In our study, after the DN rats were treated with GbE, the activities of T-AOC, T-SOD, CAT, and GSH-Px (common indicators of changes in the anti-oxidation system) all increased significantly, strongly suggesting that GbE has a potent antioxidative capability in vivo. Furthermore, we also found that GbE, even in low doses, significantly decreased AGE levels both in the kidney cortex and in the serum. These results are consistent with a former report in which GbE inhibited oxidized low density lipoprotein (LDL)-stimulated fibronectin production through an antioxidant action in rat mesangial cells[22]. These data strongly suggest that GbE has the characteristics of antioxidant and anti-AGE, and this could be of benefit for the prevention of DN.
Of the complicated mechanisms, the pathway of hyperglycemia-oxidative stress TGF-β1-ECM is assuredly very important. ROS are considered the activators of overall signaling pathways. TGF-β1 is the key cytokine mediating the production of ECM proteins. Hyperglycemic conditions generate ROS. ROS and AGE interact with and upregulate each other and activate the TGF-β1/Smad signaling path-way[23]. Furthermore, ROS generated intracellularly from the glucose metabolism, and the AGE-RAGE interaction also activate PKC (together with DAG) and MAPK pathways. These, together with activated Smads, coordinate the transcription of a wave of genes, including angiotensinogen, thrombo-spondin-1 (TSP-1), and CTGF. Angiotensin II (Ang (II) stimulates further generation of ROS and the expression of TGF-β1. More recently, Ang II blockade is rapidly becoming a standard antifibrotic therapy in renal diseases because ACE inhibitors block TGF-β1 induced by Ang II[24]. In our study, we also found that an ACE inhibitor (captopril) decreased the relative quantity of TGF-β1 mRNA and the level of CTGF. CTGF is another prosclerotic cytokine and has also been shown to be involved in both the early and later stages of DN[25]. Secreted CTGF works in concert with TGF-β1 activated by TSP-1 and ROS to transactivate subsequent waves of genes, including those encoding structural proteins whose accumulation leads to glomerulosclerosis in DN. It is becoming clear that the coordinated expression of TGF-β1 and CTGF is crucial for the induction of ECM proteins and thus, for the development of DN. Numerous studies indicate that hyperglycemia induces an increase in TGF-β1 expression at both the mRNA and protein levels in experimental and human diabetes, as well as in cultured mesangial cells, and that increased signaling by TGF-β1 is also markedly influenced by CTGF. However, the expression of some ECM proteins, such as fibronectin, is CTGF-dependent, and its promoter region does not contain any Smad-binding elements. Thus, CTGF may mediate the induction of the ECM protein expression both directly and indirectly by potentiating the TGF-β1/Smad signaling pathway. In other words, CTGF is a crucial mediator for the TGF-β1-stimulated matrix protein expression. In our experiment, after the DN rats were treated with GbE, the relative quantity TGF-β1 mRNA in the kidney cortex of the DN rats decreased significantly, strongly suggesting that GbE has an inhibitive effect on TGF-β1 mRNA in the kidney cortex. This is consistent with a previous report about the effect of GbE on TGF-β1[26]. We also found that the expression of CTGF on GbE-treated DN rats markedly decreased. Therefore, in this aspect, GbE can be a prime candidate for the prevention and treatment of DN.
The ECM is a complex structure that influences the behavior of its resident cells, and its accumulation correlates closely with renal impairment in diabetes and ultimately leads to glomerular scarring. Therefore, a balance between ECM synthesis and degradation is a prerequisite for maintaining the structural and functional integrity of the glomerulus. MMP are a group of zinc dependent endopeptidases with similar biochemical natures that are capable of degrading all components of ECM and basement membranes. Thus, changes in the MMP expression or activity will directly translate into altered ECM turnover. MMP-2 is the main MMP responsible for the degradation of collagen IV. It is secreted in an inactive form that becomes activated on the cell surface by a membrane type 1 MMP. The activity of MMP-2 is also regulated by specific tissue inhibitors of MMP (TIMP-1 and TIMP-2), that were modulated directly and indirectly by TGF-β1. So the balance between MMP and TIMP is important in the fibrotic process. MMP is one of the main proteinases that participates in the degradation of ECM. In our study, the levels of MMP-2 and TIMP-2 in the DN group conspicuously changed. The expression of MMP-2 greatly decreased and TIMP-2 increased in the DN group. After treatment with GbE, the level of MMP-2 increased and TIMP-2 decreased. This result strongly suggests that GbE has a potent inhibitory effect on the accumulation of ECM.
For the complexity of the mechanisms of DN, it is necessary to develop new drugs to deal with more than 1 pharmacological target. In our STZ–induced, early DN rat model, we found that GbE reduced the blood glucose level, decreased the level of AGE, the intensity of oxidative stress, the level of TGF-β1 mRNA, TIMP-2, CTGF, increased MMP-2, further lowered the levels of collagen IV and laminin in the kidney cortex, and deceased the thickness of GBM, and therefore ameliorated the morbidity in physical behavior and morphology. Therefore, GbE has protective effects on several pharmacological targets in the complicated pathology mechanism of DN. This means that GbE may exert a protective effect on the early development of DN in STZ-induced diabetic rats.
References
- King GL, Wakasaki H. Theoretical mechanisms by which hyperglycemia and insulin resistance could cause cardiovascular diseases in diabetes. Diabetes Care 1999;22:C31-7.
- Sharma K, McGowan TA. TGF-beta in diabetic kidney disease: role of novel signaling pathways. Cytokine Growth Factor Rev 2000;11:115-23.
- Sakharova OV, Taal MW, Brenner BM. Pathogenesis of diabetic nephropathy: focus on transforming growth factor-beta and connective tissue growth factor. Curr Opin Nephrol Hypertens 2001;10:727-38.
- Lam S, van der Geest RN, Verhagen NA, van Nieuwenhoven FA, Blom IE, Aten J, et al. Connective tissue growth factor and IGF-I are produced by human renal fibroblasts and cooperate in the induction of collagen production by high glucose. Diabetes 2003;52:2975-83.
- Woessner JF. Matrix metalloproteinases and their inhibitors in connective tissue remodeling. FASEB J 1991;5:2145-54.
- Ross R. Atherosclerosis–an inflammatory disease. N Engl J Med 1999;340:115-26.
- Springer TA. Traffic signals for lymphocyte recirculation and leukocyte emigration: the multistep paradigm. Cell 1994;76:301-14.
- Akisu M, Kultursay N, Coker I, Huseyinov A. Platelet-activating factor is an important mediator in hypoxic ischemic brain injury in the newborn rat. Flunarizine and Ginkgo biloba extract reduce PAF concentration in the brain. Biol Neonate 1998;74:439-44.
- Aminorroaya A, Janghorbani M, Rezvanian H, Aminian T, Gharavi M, Amini M. Comparison of the effect of pentoxifylline and captopril on proteinuria in patients with type 2 diabetes mellitus. Nephron Clin Pract 2005;99:C73-7.
- Yavuz D, Kucukkaya B, Haklar G, Ersoz O, Akoglu E, Akalin S. Effects of captopril and losartan on lipid peroxidation, protein oxidation and nitric oxide release in diabetic rat kidney release in diabetic rat kidney. Prostaglandins Leukot Essent Fatty Acids 2003;69:223-7.
- Blownlee M, Vlassara H, Cerami A. Nonenzymatic glycosylation products on collagen covalently trap low-density lipoprotein. Diabetes 1985;34:938-41.
- Wang JY, Yin XX, Wu YM, Tang DQ, Gao YY, Wan MR, et al. Ginkgo biloba extract suppresses hypertrophy and extracellular matrix accumulation in rat mesangial cells. Acta Pharmacol Sin 2006;27:1222-30.
- Suzuki YJ, Forman J, Sevanian A. Oxidants as stimulators of signal transduction. Free Radic Biol Med 1997;22:269-85.
- Simon AR, Rai U, Fanburg BL, Cochran BH. Activation of the JAK-STAT pathway by reactive oxygen species. Am J Physiol 1998;275:C1640-52.
- Palmer HJ, Paulson KE. Reactive oxygen species and antioxidants in signal transduction and gene expression. Nutr Rev 1997;55:353-61.
- Nath KA, Grande J, Croatt A, Haugen J, Kim Y, Rosenberg ME. Redox regulation of renal DNA synthesis, transforming growth factor-beta1 and collagen gene expression. Kidney Int 1998;53:367-81.
- Jerums G, Panagiotopoulos S, Forbes J, Osicka T, Cooper M. Evolving concepts in advanced glycation, diabetic nephropathy, and diabetic vascular disease. Arch Biochem Biophys 2003;419:55-62.
- Beisswenger PJ, Makita Z, Curphey TJ, Moore LL, Jean S, Brinck-Johnsen T, et al. Formation of immunochemical advanced glycosylation end products precedes and correlates with early manifestations of renal and retinal disease in diabetes. Diabetes 1995;44:824-9.
- Thornalley PJ. Cell activation by glycated proteins, AGE receptors, receptor recognition factors and functional classification of AGEs. Cell Mol Biol 1998;44:1013-23.
- Schmidt AM, Hori O, Brett J, Yan SD, Wautier JL, Stern D. Cellular receptors for advanced glycation end products. Implications for induction of oxidant stress and cellular dysfunction in the pathogenesis of vascular lesions. Arterioscler Thromb 1994;14:1521-8.
- Pugliese G, Pricci F, Romeo G, Pugliese F, Mene P, Giannini S, et al. Upregulation of mesangial growth factor and extracellular matrix synthesis by advanced glycation end products via a receptor-mediated mechanism. Diabetes 1997;46:1881-7.
- Akiba S, Chiba M, Mukaida Y, Tamura A, Sato T. The leaf extract of Ginkgo biloba L. suppresses oxidized LDL-stimulated fibronection production through an antioxidant action in rat mesangial cells. Br J Pharmacol 2004;142:419-24.
- Wang JY, Yin XX. Relationship between transforming growth factor-beta 1 signal transduction and renal fibrosis of diabetes. Chin J Pharmacol Ther 2005;10:371-6.
- Ruperez M, Ruiz-Ortega M, Esteban V, Lorenzo O, Mezzano S, Plaza JJ, et al. Angiotensin II increases connective tissue growth factor in the kidney. Am J Pathol 2003;163:1937-47.
- Liu BC, Chen Q, Luo DD, Sun J, Phillips AO, Ruan XZ, et al. Mechanisms of irbesartan in prevention of renal lesion in streptozotocin-induced diabetic rats. Acta Pharmacol Sin 2003;24:67-73.
- Tang L, Zhang MX. The effects of Ginkgo biloba extract on TGF-β1 and c-fos expression in kidney of streptozotocin-induced diabetic rats. Acta Med Sin 2002;15:726-9.