(5R)-5-hydroxytriptolide (LLDT-8) protects against bleomycin-induced lung fibrosis in mice1
Introduction
Idiopathic pulmonary fibrosis is a chronic, progressive form of interstitial lung disease, associated with an extremely poor prognosis for survival in most patients. Most patients die of progressive respiratory failure within 3–8 years of the onset of symptoms. Considerable experimental evidence implicated both increased collagen production and reduced degradation, leading to an irreversible distortion of normal tissue architecture and loss of function[1–3]. However, the pathogenesis of idiopathic pulmonary fibrosis still remains unknown; early lung inflammatory response and subsequent fibrotic changes are well appreciated in the time course of this disease, and until now, there has been no satisfactory treatment for this disease. Bleomycin, a mixture of glycopeptides derived from Streptomyces verticillus, is a potent chemotherapeutic agent used for the treatment of lympho-mas, head and neck cancers, and various tumors. Moreover, bleomycin is also known to produce lung injury and fibrosis in humans as well as in experimental animals[4].
The Chinese traditional herb Tripterygium wilfordii Hook F and its extracts have been widely used in the treatment of autoimmune diseases, including rheumatoid arthritis and systemic lupus erythematosus[5–8]. Triptolide is identified as the most active component accounting for the immunosuppressive effects of Tripterygium wilfordii Hook F[9]. However, the strong toxicity of triptolide limits its application to a great extent[10]. Recently, (5R)-5-hydroxytriptolide (LLDT-8), a new compound derived from triptolide, was synthesized and showed similar immunosuppressive activity to that of triptolide, but its toxicity was greatly reduced in vitro and in vivo. In vitro, LLDT-8 significantly inhibited mitogen-induced T and B cell proliferation, and mixed lymphocyte reaction, inflammatory, and Th1 type cytokines release[11]. In vivo, LLDT-8 suppressed the bovine type II collagen-induced arthritis in DBA/1 mice[12] and adjuvant-induced arthritis in Wistar rat (our unpublished observations). LLDT-8 prevented graft-versus-host disease and prolonged allogeneic cardiac transplantation survival in mice[13,14]. LLDT-8 attenuated the concanavalin A-induced liver hepatitis[15] and prolonged mice survival in the MRL-lpr/lpr murine model of systemic lupus erythematosus (our unpublished observa-tions).
The purpose of the present study was to extend our findings of LLDT-8 to an in vivo mouse lung fibrosis model for further exploration of its anti-inflammatory and antifibrosis action.
Materials and methods
Animals Female C57BL/6 mice (6–8 weeks old, 20–22 g) were purchased from the Shanghai Experimental Animal Center of the Chinese Academy of Sciences (Shanghai, China). The animals were housed in specific pathogen-free condi-tions. All mice were allowed to acclimatize in our facility for 1 week before any experiments were started. All experiments were carried out according to the National Institutes of Health Guide for the Care and Use of Laboratory Animals, and were approved by the Bioethics Committee of the Shanghai Institute of Materia Medica (Shanghai, China).
Test compound LLDT-8 was synthesized from triptolide that was separated from Tripterygium wilfordii Hook F. LLDT-8 is a white, amorphous powder with 99% purity by reverse phase, high-performance liquid chromatography. The stock solution of LLDT-8 (5 mg/mL) was prepared in 2-methyl-1,3-propanediol, stored at 4 °C, and diluted to a desired concentration with saline (10 mL/kg, ip). The final concentration of 2-methyl-1,3-propanediol in the dosing solution was 4%.
Experimental model of bleomycin-induced lung fibrosis Lung fibrosis was induced as described by previous studies with minor modifications[4,16]. Briefly, after the body weight was recorded, the mice were anesthetized via intraperitoneal injection of 30 mg/kg pentobarbital sodium. A midline incision was made in the neck, and the trachea was exposed by blunt dissection. Bleomycin hydrochloride (Nippon Kayaku, Tokyo, Japan), was dissolved in 0.1 mL saline and injected into the animals’ lungs via the 0.25 mL syringes at a dose of 7.5 mg/kg body weight. The normal control received an equal volume of sterile saline. After bleomycin or saline were injected into the trachea, the animal operating plate was erected and shaken to facilitate distribution of the solution throughout the lungs. The day of bleomycin injection was considered d 0 and the weight of the animals was recorded every 3–4 d.
Group assignment and drug administration The mice were randomly assigned to 5 body weight-matched groups: normal control, bleomycin, and the bleomycin with LLDT-8 treatment groups (LLDT-8 at 2 mg/kg, 1 mg/kg and 0.5 mg/kg). In most of the experiments, each group consisted of 8 mice, except the bronchoalveolar lavage assay, and 3 animals per group were analyzed. LLDT-8 was daily administered to the mice by ip from d 1. The normal and bleomycin control group were daily injected with vehicle solution by ip.
Bronchoalveolar lavage analysis On d 7 after bleomycin treatment, the mice were sacrificed. The thorax was opened by a median incision and the trachea was cannulated with a plastic catheter attached to a 2 mL syringe. Bronchoalveolar lavage was performed in 4 mL sterile saline with gentle massaging of the lungs. The bronchoalveolar lavage fluid were collected and centrifuged at 150×g for 10 min at 4 °C. The total number of cells in the lavage fluid was counted with trypan blue staining. The cell subsets were counted with Giemsa staining by examining 200 cells per animal. The cell numbers of macrophages, neutrophils, and lymphocytes in the lavage fluid was calculated according to their respective percentages in the total cells.
Lung tissue preparation and biochemical assay One hour after LLDT-8 or vehicle administration on d 7 or 14, the mice were sacrificed by bleeding. The lungs were removed and weighed, washed twice with cold saline, and then each lung was divided into 2 parts: the right one was fixed in 10% formalin solution for histological examination and the left one was prepared for biochemical assay and cytokine detection.
The lung samples were prepared as 10% homogenate in 0.9% saline by homogenizer on ice according to their respective weight. Then the homogenate was centrifuged, and the supernatant was collected and diluted. The assay of superoxide dismutase (SOD), malondialdehyde, and hydroxyproline levels followed the manufacturer’s instructions (Nanjing Jiancheng Bioengineering Institute, Nanjing, China).
Histological examination of fibrosis The lung samples were washed and fixed in buffered 10% formalin solution. After embedded in paraffin, 5 µm sections were stained with hematoxylin-eosin (HE), and examined by 2 pathologists who were blinded to the experiment.
Measurement of tumor necrosis factor-α (TNF-α), interleukin-4 (IL-4), and transforming growth factor-β (TGF-β) The lung homogenate (10%) was centrifuged at 10 600×g for 30 min at 4 °C to remove debris, and the supernatants were assayed for TNF-α, IL-4, and TGF-β concen-trations. The levels of TNF-α and IL-4 were determined using sandwich ELISA kits from PharMingen (San Diego, CA, USA) following the manufacturer’s instructions. The TGF-β level was determined using a Mv1Lu cell proliferation assay[17]. Briefly, the Mv1Lu cells (2×104/well) were cultured in the presence of diluted acidified samples or recombinant TGF-β (R&D Systems, Minneapolis, MN, USA) in 96-well plates for 24 h at 37 °C in an incubator with 5% CO2. The cells were pulsed with 0.5 mCi of [3H]-thymidine for 8 h and harvested onto glass fiber filters. The incorporated radioactivity was then counted using a Beta Scintillation Counter (MicroBeta Trilux, Perkin-Elmer Life Sciences, Boston, MA, USA). The concentration of TGF-β in the lung homogenate was calculated according to the rTGF-β inhibitory standard curve. The anti-TGF-β 1,2,3 antibody (Genzyme, Framingham, MA, USA) was used to confirm the specific inhibition by TGF-β in the Mv1Lu cells. The sample was acidified to pH 2 with 1 mol/L HCl for 30 min on ice and then neutralized with 1 mol/L NaOH.
Statistical analysis Data were expressed as mean±SEM or mean±SD where indicated. Statistical differences were analyzed according to the analysis of variance, followed by post-hoc multiple comparison tests (LSD). P<0.05 was considered to be significant.
Results
LLDT-8 attenuated bleomycin-induced lung injury Compared with the normal control, the body weight in the bleomycin-treated animals decreased gradually and reached the lowest level at d 7 after bleomycin injection, and then tended to recover. LLDT-8 displayed protective effects on the loss of body weight (Figure 1). Moreover, LLDT-8 was well tolerated in the bleomycin-treated mice, showing no change in mobility, skin hair, and respiration throughout the experiment.
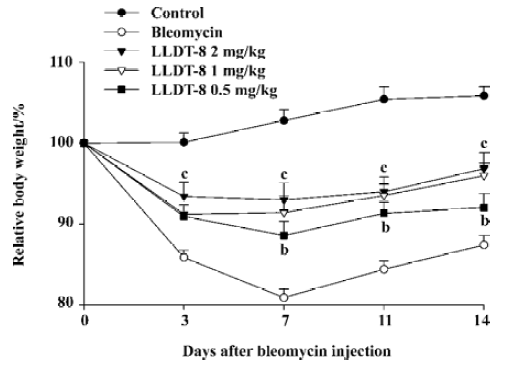
In contrast to the loss of body weight, the weight of the lungs increased obviously in the bleomycin-treated mice, resulting in augmentation of the lung index (lung weight versus body weight). LLDT-8 treatment dose-dependently inhibited the increase of the lung index as compared with that of the vehicle-treated bleomycin group on d 7 and 14 (Figure 2).
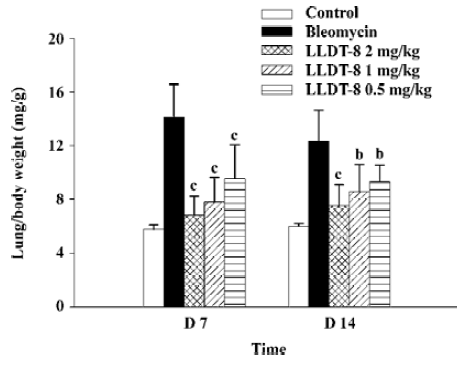
The lungs were examined histologically on d 14 after bleomycin injection. Data are shown in Figure 3. The lung architecture appeared intact in the normal control group. In the bleomycin control group, there were multifocal diffuse changes consisting of some combinations of thickened alveolar septa, interstitial hyperplasia, intra-alveolar fibrosis with myofibroblasts, occasional foci of dense fibrosis, increased alveolar macrophages, and some infiltration of inflammatory cells. However, in the LLDT-8-treated mice, there was a marked decrease in inflammation and fibrosis. No obvious fibrotic focal was observed in the 2 mg/kg LLDT-8-treated group.
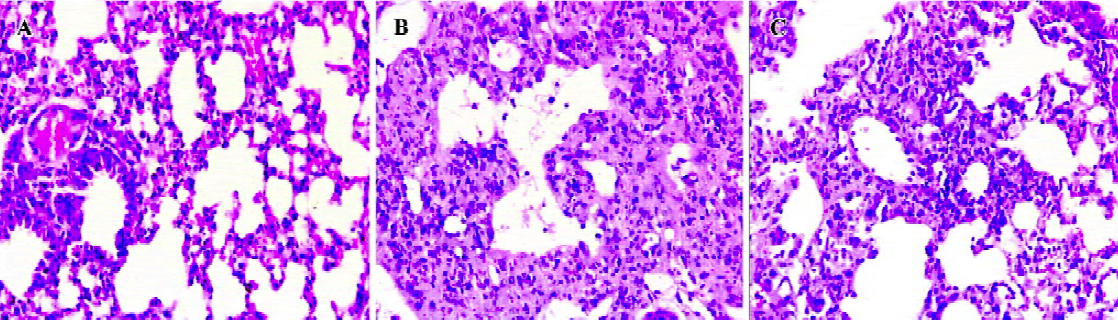
LLDT-8 suppressed lung inflammatory cell expansion induced by bleomycin treatment The number of total cells and the subsets including macrophages, lymphocytes, and neutrophils in the bronchoalveolar lavage fluid were elevated markedly in response to bleomycin on d 7 (Table 1). LLDT-8 administration dose-dependently reduced the numbers of total cells, neutrophils, as well as lymphocytes, but weakly affected the macrophages number.
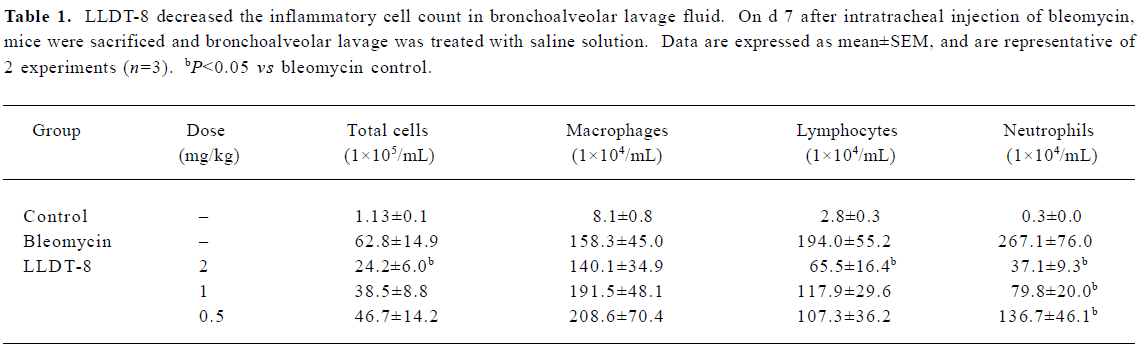
Full table
LLDT-8 reduced hydroxyproline and malondialdehyde production but enhanced SOD activity in bleomycin-treated mice To assess the total collagen content and fibrotic process, we determined the lung hydroxyproline level. Data are presented in Table 2. In the lung homogenates from the bleomycin control mice, the hydroxyproline level increased on d 7 and 14. Administration of LLDT-8 reduced the hydroxyproline production. On d 14, LLDT-8 at 2 mg/kg, 1 mg/kg, and 0.5 mg/kg decreased the hydroxyproline level by 30.4%, 23.9%, and 15.1%, respectively. Moreover, LLDT-8 at 2 mg/kg effectively reduced the hydroxyproline production to the basal level as that in the normal control.
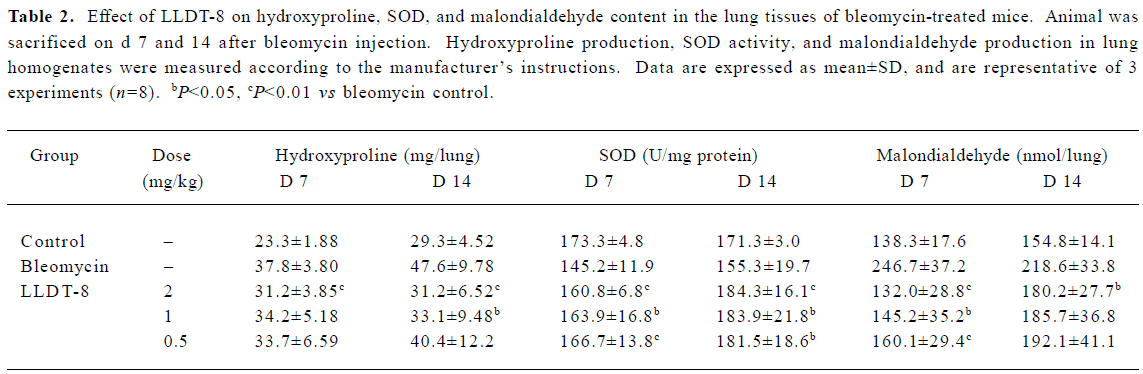
Full table
The deprivation of antioxidant enzyme SOD indirectly reflects reactive oxygen species production in response to bleomycin. As shown in Table 2, LLDT-8 significantly prevented the decrease of SOD activity on d 7. On d 14, SOD activity tended to recover in the bleomycin control group; meanwhile, LLDT-8 still showed an enhancing effect on SOD activity.
Malondialdehyde is a marker of lipid peroxidation. In this study, malondialdehyde content in the lung tissue was remarkably elevated after bleomycin treatment. The increased percentages were 78.4% and 41.2% on d 7 and 14, respec-tively. LLDT-8 inhibited bleomycin-induced malondial-dehyde production in a dose-dependent manner. In the 2 mg/kg LLDT-8-treated group, its production was reduced to the basal level as that in the normal control on d 7. On d 14, the beneficial effect of LLDT-8 was still observed when given at 2 mg/kg (Table 2).
LLDT-8 inhibited pro-inflammatory and pro-fibrotic cytokine production in lung homogenates To evaluate the roles of key cytokines in lung fibrosis, we detected TNF-α, IL-4, and TGF-β levels in lung homogenates. Data are presented in Figure 4. Administration of LLDT-8 significantly inhibited TNF-α production on d 7 in a dose-dependent manner (Figure 4A). In addition, a remarkable increase of IL-4 levels was seen in the bleomycin control group on d 14 (Figure 4B), and LLDT-8 treatment effectively suppressed this increase.
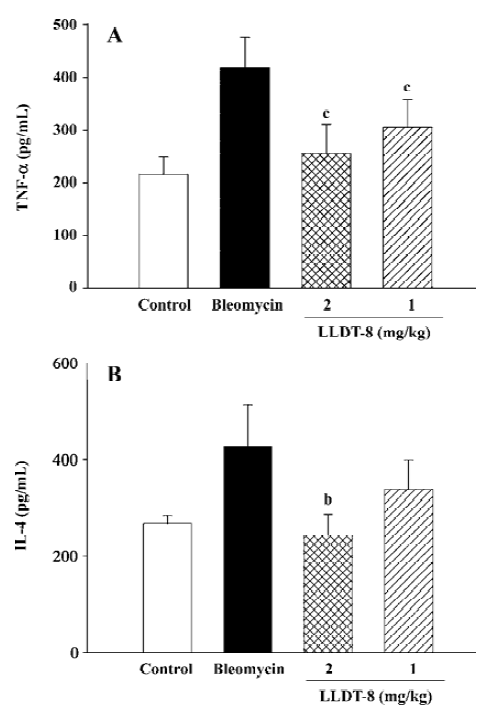
In the Mv1Lu cell proliferation assay, TGF-β concentration in the lung tissue elevated from 580 pg/mL in the normal control group to 880 pg/mL on d 7, and reached 1012 pg/mL on d 14 after bleomycin injection (Figure 5). The increase of the TGF-β level was suppressed after LLDT-8 treatment. In addition, the anti-TGF-β1, 2, 3 antibody was taken to test the specificity of proliferative inhibition by TGF-β. As expected, the suppressed cell proliferation was almost completely restored by this antibody (data not shown).
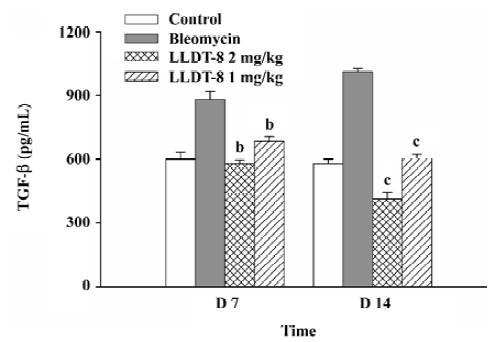
Discussion
Idiopathic pulmonary fibrosis is a progressive lung disease with unknown pathogenesis. The role of the inflammation and anti-inflammatory strategy still remain controversial. It was suggested that in addition to inflammatory cells, alveolar epithelial cells, mesenchymal precursor cells, fibro-blasts, and myofibroblasts played an important role in the diverse processes of fibrosis[2]. Thus, potential therapeutic strategies can be developed at any stage which result in idiopathic pulmonary fibrosis. These include agents that interfere with the action of inflammatory mediators, agents that prevent parenchymal cell damage, agents that prevent the proliferation of fibroblasts and collagen synthesis, agents that downregulate myofibroblast differentiation, and agents that intervene with 1 or more key events in the pathogenesis or signal transduction pathways of idiopathic pulmonary fibrosis[1]. In addition, it is unlikely that any single treatment can be sufficiently effective in the case of lung fibrosis.
Bleomycin-induced animal lung injury has been widely used as a model of human lung fibrosis because some biochemical and functional changes in the early stages in animals resemble that in humans. Other studies from long-term observations which reported the physiological and histological changes at late stages (d 120) in rats were very different from human disease[18], so that extrapolation of the data from the animal model to humans needs to be taken with caution. However some similarities exist between the bleomycin animal model and human lung fibrosis, and this model is informative for antifibrosis agent evaluation and potential mechanism research.
The inflammatory response to bleomycin is orchestrated partially by endogenous and migrating leukocytes, which is also well demonstrated in our present study. These activated leukocytes can synthesize and secrete various cytokines, chemokines, reactive oxygen species, and proteases that sustain the injury/repair processes. Neutrophils isolated from the bronchoalveolar lavage had a greater capacity to produce superoxide anion than those from the blood, and resulted in lung damage[19]. Moreover, these leukocytes together with lung epithelial and endothelial cells produced a feedback circle where stimuli from injury responses could activate alveolar and interstitial macrophages[20]. LLDT-8 ameliorated exudation in lung tissue and reduced the leukocytes number, which would result in a decrease in source of free radicals.
Substantial data prove the cellular redox state and the oxidant–antioxidant balance play a critical role in the pathogenesis of lung fibrosis in animal models and possibly in humans[21,22]. In addition to the inflammation mediator, the high level of oxidants may increase TGF-β release[23], activate protease, and enhance the fibrotic response; some antioxidants including N-acetylcysteine, and SOD can decrease collagen deposition and protect the lungs in a variety of animal models or even in clinical trials[24]. LLDT-8 served as a free-radical scavenger, enhanced SOD ability, and inhibited lipid peroxidation, which helped to ameliorate inflammatory reaction, meanwhile possibly contributing to decreased TGF-β production and alleviating fibrosis change.
Cytokines are involved in the fibrosis process. TNF-α induces adhesion molecule expression by vascular endothelial cells and intensifies the recruitment of inflammatory cells into the lungs. Moreover, TNF-α is relevant to the induction of fibrosis by augmenting synthesis of fibronectin, prostaglandin, and TGF-β. Administration of the anti-TNF-α antibody, soluble TNF-α is demonstrated to be beneficial in suppressing bleomycin-induced lung injury[25,26], and now a phase II clinical trial of soluble TNF-α receptor (Etanercept) is under way[1,27]. In our study, LLDT-8 markedly inhibited TNF-α production, providing 1 possible mechanism of its protective effect against lung injury.
The role of Th1 and Th2 cytokines in lung fibrosis remains controversial[28–32]. Recent studies disclosed that IL-4 might play a selective anti-inflammatory role during initial lung injury stages by limiting the early accumulation of T cells, but IL-4 promoted fibroblast proliferation and collagen deposition during the later stages of fibrosis[33–36]. The IL-4 level in lung tissues was detected on d 7 and 14; the IL-4 increase on d 7 was not evident, and the inhibitory effect of LLDT-8 was not significant (data not shown). However, LLDT-8 suppressed IL-4 production on d 14 after bleomycin injection, which possibly contributed to the blockade of lung injury.
Moreover, we detected IFN-γ and IL-10 levels in lung homogenates on d 7 and 14 after bleomycin injection. LLDT-8 did not affect these 2 cytokine production (data not shown). However, LLDT-8 decreased IFN-γ from concanavalin A or Sac-stimulated murine spleen cells in vitro[11]. The possible reason for this needs further investigation.
TGF-β is a pivotal mediator in lung fibrosis and has a broad spectrum of activities in pulmonary inflammation, tissue repair, and fibrosis. TGF-β can serve as a chemoattractant for fibroblasts and monocytes/macrophages and stimulate these cells to synthesize a number of pro-inflammatory and fibrogenic cytokines such as TNF-α, IL-1β, and TGF-β itself. At the same time, TGF-β is one of the most potent inducers of extracellular matrix production. TGF-β reduces the breakdown of collagen and other matrix proteins by inhibiting the generation of plasminogen activators, matrix metallopro-teinase, and elastase, as well as by enhancing the expression of tissue inhibitors of metalloproteinases, plasminogen activator inhibitor-1,2[1,37]. In our study, TGF-β production in lung homogenates elevated gradually after bleomycin treatment, while LLDT-8 potently suppressed TGF-β production and retained it at basal level as that in the normal control. Then, the reduced hydroxyproline production and lung injury by LLDT-8 was at least partially attributed to its inhibition of TGF-β production.
In conclusion, LLDT-8 demonstrated protective effects against bleomycin-induced murine pulmonary fibrosis. The beneficial effect of LLDT-8 might be closely associated with its activities of anti-inflammation, antioxidant, and cytokine inhibition.
Acknowledgement
We thank the research group of Dr Jin REN (Shanghai Institute of Materia Medica) for providing valuable technical assistance with histology.
References
- Gharaee-Kermani M, Phan SH. Molecular mechanisms of and possible treatment strategies for idiopathic pulmonary fibrosis. Curr Pharm Des 2005;11:3943-71.
- Khalil N, O’Connor R. Idiopathic pulmonary fibrosis: current understanding of the pathogenesis and the status of treatment. Can Med Assoc J 2004;171:153-60.
- Gross TJ, Hunninghake GW. Idiopathic pulmonary fibrosis. N Engl J Med 2001;345:517-25.
- Ozyurt H, Sogut S, Yildirim Z, Kart L, Iraz M, Armutcu F, et al. Inhibitory effect of caffeic acid phenethyl ester on bleomycin-induced lung fibrosis in rats. Clin Chim Acta 2004;339:65-75.
- Asano K, Matsuishi J, Yu Y, Kasahara T, Hisamitsu T. Suppressive effects of Tripterygium wilfordii Hook f., a traditional Chinese medicine, on collagen arthritis in mice. Immunopharma-cology 1998;39:117-26.
- Gu WZ, Banerjee S, Rauch J, Brandwein SR. Suppression of renal disease and arthritis, and prolongation of survival in MRL-lpr mice treated with an extract of Tripterygium wilfordii Hook f. Arthritis Rheum 1992;35:1381-6.
- Gu WZ, Brandwein SR. Inhibition of type II collagen-induced arthritis in rats by triptolide. Int J Immunopharmacol 1998;20:389-400.
- Tao X, Davis LS, Hashimoto K, Lipsky PE. The Chinese herbal remedy, T2, inhibits mitogen-induced cytokine gene transcription by T cells, but not initial signal transduction. J Pharmacol Exp Ther 1996;276:316-25.
- Chen BJ. Triptolide, a novel immunosuppressive and anti-inflammatory agent purified from a Chinese herb Tripterygium wilfordii Hook F. Leuk Lymphoma 2001;42:253-65.
- Huynh PN, Hikim AP, Wang C, Stefonovic K, Lue YH, Leung A, et al. Long-term effects of triptolide on spermatogenesis, epididymal sperm function, and fertility in male rats. J Androl 2000;21:689-99.
- Zhou R, Zhang F, He PL, Zhou WL, Wu QL, Xu JY, et al. (5R)-5-hydroxytriptolide (LLDT-8), a novel triptolide analog mediates immunosuppressive effects in vitro and in vivo. Int Immuno-pharmacol 2005;5:1895-903.
- Zhou R, Tang W, Ren YX, He PL, Zhang F, Shi LP, et al. (5R)-5-hydroxytriptolide attenuated collagen-induced arthritis in DBA/1 mice via suppressing interferon-gamma production and its related signaling. J Pharmacol Exp Ther 2006;318:35-44. b.
- Tang W, Yang Y, Zhang F, Li YC, Zhou R, Wang JX, et al. Prevention of graft-versus-host disease by a novel immuno-suppressant, (5R)-5-hydroxytriptolide (LLDT-8), through expansion of regulatory T cells. Int Immunopharmacol 2005;5:1904-13.
- Tang W, Zhou R, Yang Y, Li YC, Yang YF, Zuo JP. Suppression of (5R)-5-hydroxytriptolide (LLDT-8) on allograft rejection in full MHC-mismatched mouse cardiac transplantation. Transplantation 2006;81:927-33.
- Zhou R, Tang W, Ren YX, He PL, Yang YF, Li YC, et al. Preventive effects of (5R)-5-hydroxytriptolide on concanavalin A-induced hepatitis. Eur J Pharmacol 2006;537:181-9. a.
- Kremer S, Brever R, Lossos IS. Effect of immunomodulators on bleomycin-induced lung injury. Respiration 1999;66:455-62.
- Li XF, Takiuchi H, Zou JP, Katagiri T, Yamamoto N, Nagata T, et al. Transforming growth factor-β (TGF-β)-mediated immunosuppression in the tumor-bearing state: enhanced production of TGF-β and a progressive increase in TGF-β susceptibility of anti-tumor CD4+ T cell function. Jpn J Cancer Res 1993;84:315-25.
- Borzone G, Moreno R, Urrea R, Meneses M, Oyarzun M. Bleomycin-induced chronic lung damage does not resemble human idiopathic pulmonary fibrosis. Am J Respir Crit Care Med 2001;163:1648-53.
- Tarnell EB, Oliver BL, Johnson GM, Watts FL, Thrall RS. Superoxide anion production by rat neutrophils at various stages of bleomycin-induced lung injury. Lung 1992;170:41-50.
- Oury TD, Thakker K, Menache M, Chang LY, Crapo JD, Day BJ. Attenuation of bleomycin-induced pulmonary fibrosis by a catalytic antioxidant metalloporphyrin. Am J Respir Crit Care Med 2001;164:164-9.
- Kinnula VL, Fattman CL, Tan RJ, Oury TD. Oxidative stress in pulmonary fibrosis. Am J Respir Crit Care Med 2005;172:417-22.
- Kuwanl K, Nakashima N, Inoshima I, Hagimoto N, Fujita M, Yoshimi M, et al. Oxidative stress in lung epithelial cells from patients with idiopathic interstitial pneumonias. Lab Invest 2002;82:1695-706.
- Bellocq A, Azoulay C, Marullo S, Flahault A, Fouqueray B, Philippe C, et al. Reactive oxygen and nitrogen intermediates increase transforming growth factor-beta 1 release from human epithelial alveolar cells through two different mechanisms. Am J Respir Cell Mol Biol 1999;21:128-36.
- Salvemini D, Riley DP, Cuzzocrea S. SOD mimetics are coming of age. Nat Rev Drug Discov 2002;1:367-74.
- Piguet PF, Ribaux C, Karpuz V, Grau GE, Kapanci Y. Expression and localization of tumor necrosis factor-alpha and its mRNA in idiopathic pulmonary fibrosis. Am J Pathol 1993;143:651-5.
- Yara S, Kawakami K, Kudeken N, Tohyama M, Teruya K, Chinen T, et al. FTS reduced bleomycin-induced cytokine and chemokine production and inhibits pulmonary fibrosis in mice. Clin Exp Immunol 2001;124:77-85.
- Walter N, Collard HR, King TE Jr. Current perspectives on the treatment of idiopathic pulmonar fibrosis. Proc Am Thorac Soc 2006;3:330-8.
- Izbicki G, Segel MJ, Christensen TG, Conner MW, Breuer R. Time course of bleomycin-induced lung fibrosis. Int J Exp Path 2002;83:111-9.
- Gharaee-Kermani M, Phan SH. Role of cytokines and cytokine therapy in wound healing and fibrotic diseases. Curr Pharm Des 2001;11:1083-103.
- Lossos IS, Or R, Goldstein RH, Conner MW, Breuer R. Amelioration of bleomycin-induced pulmonary injury by cyclosporin A. Exp Lung Res 1996;22:337-49.
- Zhu J, Cohen DA, Goud SN, Kaplan AM. Contribution of T lymphocytes to the development of bleomycin-induced pulmonary fibrosis. Ann NY Acad Sci 1996;796:194-202.
- Sharma SK, MacLean JA, Pinto C, Kradin RL. The effect of an anti-CD3 monoclonal antibody on bleomycin-induced lymphokine production and lung injury. Am J Respir Crit Care Med 1996;154:193-200.
- Huaux F, Liu T, McGarry B, Ullenbruch M, Phan SH. Dual roles of IL-4 in lung injury and fibrosis. J Immunol 2003;170:2083-92.
- Arai T, Abe K, Matsuoka H, Yoshida M, Mori M, Goya S, et al. Introduction of the interleukin-10 gene into mice inhibited bleomycin-induced lung injury in vivo. Am J Physiol Lung Cell Mol Physiol 2000;278:L914-22.
- Sakamoto H, Zhao LH, Jain F, Kradin R. IL-12p40(-/-) mice treated with intratracheal bleomycin exhibit decreased pulmonary inflammation and increased fibrosis. Exp Mol Pathol 2002;72:1-9.
- Gharaee-Kermani M, Nozaki Y, Hatano K, Phan SH. Lung interleukin-4 gene expression in a murine model of bleomycin-induced pulmonary fibrosis. Cytokine 2001;15:138-47.
- Krishna G, Liu K, Shigemitsu H, Gao M, Raffin TA, Rosen GD. PG490-88, a derivative of triptolide, blocks bleomycin-induced lung fibrosis. Am J Pathol 2001;158:997-1004.