Pretreatment with insulin enhances anticancer functions of 5-fluorouracil in human esophageal and colonic cancer cells
Introduction
For more than 40 years, 5-fluorouracil (5-FU) has been widely used as the first-line drug in many clinical anticancer therapies[1–3]. However, nowadays 5-FU resistance during the course of treatment has become common, which is an important cause of failure for cancer therapy[4]. Several studies have postponed the proper model for the resistance effect of 5-FU, including transport mechanisms, metabolism, drug-detoxifying mechanisms, cell cycle kinetics, and protection from apoptosis[5,6]. According to these mechanisms, various methods were adopted to improve the effects of 5-FU; for example, increasing the uptake of drugs in tumor cells to improve therapeutic sensitivities[7]. The transport of 5-FU across the cell membrane is believed to be through passive or facilitated diffusion[8]. Theoretically, increasing the intracellular uptake of 5-FU or reducing its efflux may enhance the anticancer effects of 5-FU. 5-FU is a pyrimidine antagonist, which is converted intracellularly to several active metabolites: fluorodeoxyuridine monophosphate (FdUMP), fluorodeoxyuridine triphosphate (FdUTP), and fluorouridine triphosphate (FUTP). FdUMP binds to the nucleotide-binding site of thymidylate synthase (TS), thereby blocking binding of the normal substrate deoxyuridine monophosphate (dUMP) and inhibiting deoxythymidine monophosphate (dTMP) synthesis which is necessary for DNA replication and repair. FdUTP can be misincorporated into DNA and can lead to DNA strand breaks and cell death. FUTP is extensively incorporated into RNA, disrupting many aspects of normal RNA processing and function[6]. 5-FU has the main cytotoxic action to tumor cells in the DNA synthesis phase (S phase), but it is not S phase-dependent for the function of FUTP[9]. It is hypothesized that the anticancer effects of 5-FU would be enhanced by increasing the S phase population of tumor cells while decreasing the cell number in the G0/G1 phase[6]. Furthermore, the ability to undergo apoptosis in response to drug treatment plays an important role in the sensitivity of tumor cells to chemotherapy. The apoptosis pathway is inactive in tumors with chemotherapeutic resistance[10]. Accumulating evidence indicates that enhancing apoptosis of tumor cells in response to 5-FU may improve chemotherapeutic sensitivity[11].
In this study, we investigated the effects of insulin on enhancing 5-FU anticancer functions in human esophageal and colonic cancer cells. Insulin is known as the most potent physiological anabolic agent. It has profound effects on both carbohydrate and lipid metabolism. It also has significant influences on protein and mineral metabolism[12]. Insulin stimulates DNA synthesis and modulates transcription, altering the cell content of numerous mRNA[12]. We examined whether tumor cells were more sensitive to 5-FU after insulin enhanced cellular metabolism. In addition, insulin promotes the uptake of many nutrition materials by facilitated diffusion; for example, insulin facilitates the entry of glucose into many issues, mainly by enriching the concentration of Glut4 proteins at the plasma membrane, and stimulates the uptake of amino acids by regulating amino acid transporters[13–15]. Insulin also increases the permeability of many cells to potassium, magnesium, and phosphate ions via activating sodium-potassium ATPases[16,17]. However, its effect on the permeability of 5-FU has not been investigated.
In order to investigate the effects of insulin on chemotherapeutic sensitivity of tumor cells to 5-FU and the possible mechanisms, we performed our study in aspects of cell proliferation, cell cycle, drug uptake, inducement of apoptosis, and TS level in human esophageal and colonic tumor cells.
Materials and methods
Cell culture The human esophageal carcinoma cell line (Eca 109) and human colonic cancer cell line (Ls-174-t) were cultured in RPMI-1640 medium (Life Technologies, USA), supplemented with 10% FBS (Sijiqing Biological Engineering Co, Hangzhou, China), 100 U/mL penicillin, and 100 µg/mL streptomycin in a humidified atmosphere (5% CO2) at 37 ºC.
Cytotoxicity assay MTT is widely used to assess viability and metabolic state of cells. As described previously[18], logarithmic phase cells were plated into 96-well plates at a density of 7×103 cells per well for Eca 109 cells and 9×103 cells per well for Ls-174-t cells. After being pre-exposed to insulin (Huminsulin Lilly, Fegersheim, France), the cells were treated with 5-FU (Xudong Haipu Pharmaceutical Co, Shanghai, China) for 48 h. Then 10 µL MTT (Sigma, USA) was added to the culture medium to a final concentration of 0.5 mg/mL and incubated at 37 ºC for 4 h. After that, the formazan crystals were solubilized with 100 µL DMSO (Sigma, USA) for 15 min. The absorbance was measured at a wavelength of 570 nm with a microplate reader (model 550, Bio-Rad, Hercules, CA, USA). All assays were carried out 3 times.
Cell cycle analysis After insulin or 5-FU treatment, Eca 109 and Ls-174-t cells were washed twice with cold phosphate buffered saline (PBS) and fixed with 70% ethanol at 4 ºC for 8 h. After the ethanol was washed out, the fixed cells were treated with 50 µg/mL RNase A at 37 ºC for 1 h, and then incubated with 100 µg/mL PI (Sigma, USA) at 4 ºC for 30 min. Cell cycle was analyzed by flow cytometry (Coulter Elite ESP, Coulter, Miami, FL, USA).
DNA fragmentation assay A total of 107 cells were collected after 5-FU treatment for 48 h or pretreatment with 1 U/L insulin for 8 h, followed by 5-FU exposure for 48 h. Untreated Eca 109 and Ls-174-t cells were regarded as control groups. The cells were washed with PBS twice and the genome DNA was extracted according to the protocol of the genomic DNA extraction kit (Biology Engineering Company, Shanghai, China). Briefly, 20 µL DNA samples were sampled for electrophoresis on 1% agarose gel containing 0.1% ethidium bromide in TAE buffer (40 mmol/L Tris, 20 mmol/L sodium acetate, and 1 mmol/L EDTA, pH 8.0) and visualized by ultraviolet illumination.
Terminal transferase dUTP nick end labeling assay (TUNEL assay) After drug treatment, the cells were washed 3 times with PBS and fixed with formaldehyde. After being permeabilized with 0.1% Triton X-100 in 0.1% sodium citrate, the cells were washed twice with PBS. The DNA nick-labeling reaction was performed using 50 µL TUNEL reaction mixture, including 45 µL enzyme solution and 5 µL nucleotide mix at 37 ºC for 60 min. The cells were then washed 3 times with PBS and analyzed under a fluorescence microscope (Nikon, Tokyo, Japan).
Western blot analysis The cells were exposed to insulin (1 U/L) for 8 h, 5-FU (25 µg/mL for Eca 109 cells and 37 µg/mL for Ls-174-t cells, respectively) for 24 h, or insulin pretreatment for 8 h followed by 5-FU treatment for 24 h. After treatment, cell extracts were prepared by incubating cells with cold cell lysis buffer with 1 g/L leupeptin and 1 mmol/L phenylmethanesulfonyl fluoride (PMSF). The cell lysates were loaded in SDS-sample buffer [50 mmol/L Tris-HCl (pH 6.8), 2% (w/v) SDS, 10% glycerol, 100 mmol/L DL-Dithio-threitol, and 0.01% (w/v) bromophenol blue] and separated on a 10% SDS-PAGE. After being transferred to a nitrocellulose transfer membrane (Amersham Pharmacia Biotech, UK), the blots were incubated in blocking buffer (containing 5% skim milk powder and 0.1% Tween 20) and probed with diluted caspase-3 antibodies (1:400, CST, Danvers, MA, USA) or an antithymidylate synthase antibody (1:200 TS 106, ABCAM, Cambridge, UK) for 1 h. After washing, the membrane was incubated with horseradish peroxidase-conjugated secondary antibody (1:50, Sino-American Biotechnology, Louyang, China). The immunoblot was visualized using 3,3’-Diaminobenzidine (DAB, Sino-American Biotechnology, Louyang, China). The relative density of the bands was analyzed with TotalLab Software (ImageMaster VDS Video Documentation System, Amersham Pharmacia Biotech, UK). The image was captured using a high performance CCD (change couple device) camera and the system calculated the pixel density of the manually selected band of the immunoblot.
HPLC analysis After tumor cells with the same number were exposed to 5-FU or insulin/5-FU for 6 h, 200 µL cell culture medium of each group was collected and mixed with 3 mL ethyl acetate. The mixture was then centrifuged at 2000×g for 10 min. The organic layer was removed and evaporated to dryness. Remains were resuspended in the mobile phase.
The HPLC system (Waters, Milford, MA, USA) was utilized to determine the concentration of 5-FU in the culture medium. The mobile phase (water/methanol, 85:15) was delivered by the Waters M600E system (USA) and chromatographic patterns were recorded with Waters PDA996 photodiode array detector (USA). A Luna C18 column (ϕ4.6×250 mm, 5 µm) was used for sample separation, and the sample (10 µL) was injected onto the column at a flow rate of 0.9 mL/min at room temperature. Data were analyzed by the Millennium Chromatographic Manager (Waters, Milford, MA, USA).
Statistical analysis Data were expressed as mean±SD and analyzed with the statistical program SPSS 13.0. Q test, ANOVA, and chi-square test were used. P<0.05 was considered statistically significant.
Results
Insulin enhances the inhibitory function of 5-FU on the proliferation of Eca 109 and Ls-174-t cells After pretreatment with insulin for 2, 4, 8, 12, 24 h and followed by 5-FU treatment, the inhibition rates of 5-FU in Eca 109 and Ls-174-t cells enhanced significantly compared with 5-FU treatment, except for the 2 h group (Figure 1A, 1B). The growth inhibition of 5-FU in both cancer cell lines increased gradually when the cells were pretreated with insulin from 0 to 12 h. However, if the exposure time of insulin was longer than 12 h, the inhibition rate of 5-FU on the proliferation of the tumor cells did not enhance any more. By statistical analysis, we found that there was no significant difference among the 8, 12, and 24 h groups, which means that the growth inhibition of 5-FU can be enhanced maximally after insulin pretreatment for 8–24 h.
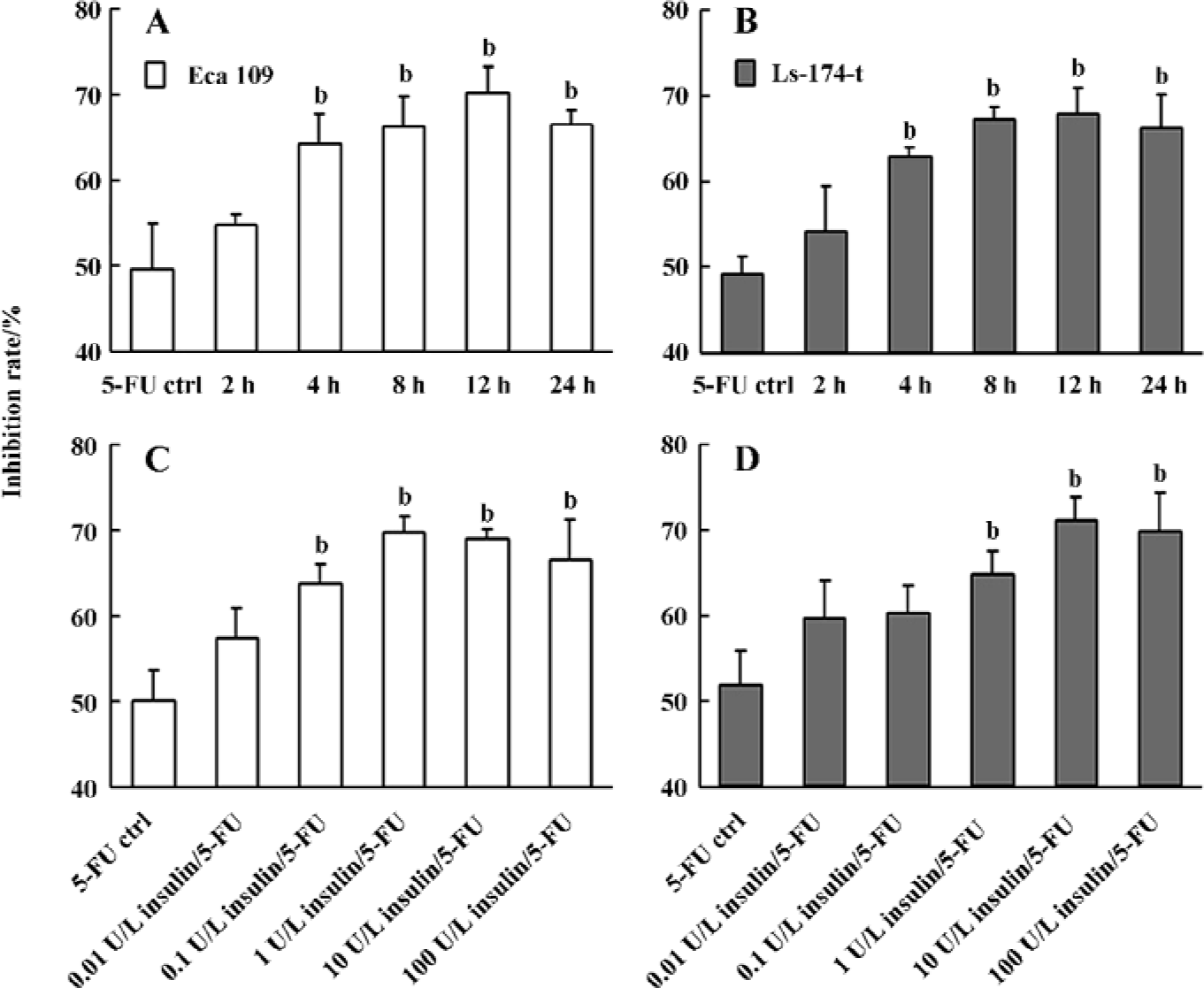
To investigate whether the upregulation of 5-FU chemotherapeutic effects by insulin is dose-dependent, we set up the dose scales of insulin from 0.01 to 100 U/L. After treatment with insulin for 8 h, the tumor cells were exposed to 5-FU for 48 h before the MTT assay. The results showed that when Eca 109 and Ls-174-t cells were pretreated with insulin in a low dose range, the inhibition rate of 5-FU increased in a dose-dependent manner. When the concentration of insulin was less than 1 U/L for Eca 109 cells or less than 10 U/L for Ls-174-t cells, the growth inhibition was dose-dependent (Figure 1C, 1D). However, we observed that the growth inhibition rate of 5-FU was similar in the high-dose insulin groups in both cancer cell lines. We proposed that this was because of the saturation of the insulin receptors on the cell membranes.
Impact of insulin on cell cycle progression The impact of insulin on cell cycle was analyzed by flow cytometry. The tumor cells were treated with insulin of different concentrations (0.01–100 U/L) for 8 h. There was a reduction in the G1 phase cells and an increase in the S phase cells in Eca 109 and Ls-174-t cells (Figure 2). The percentage of the G2/M phase cells remained much the same among all groups. It demonstrated that insulin recruited G1 phase cells to the S phase.
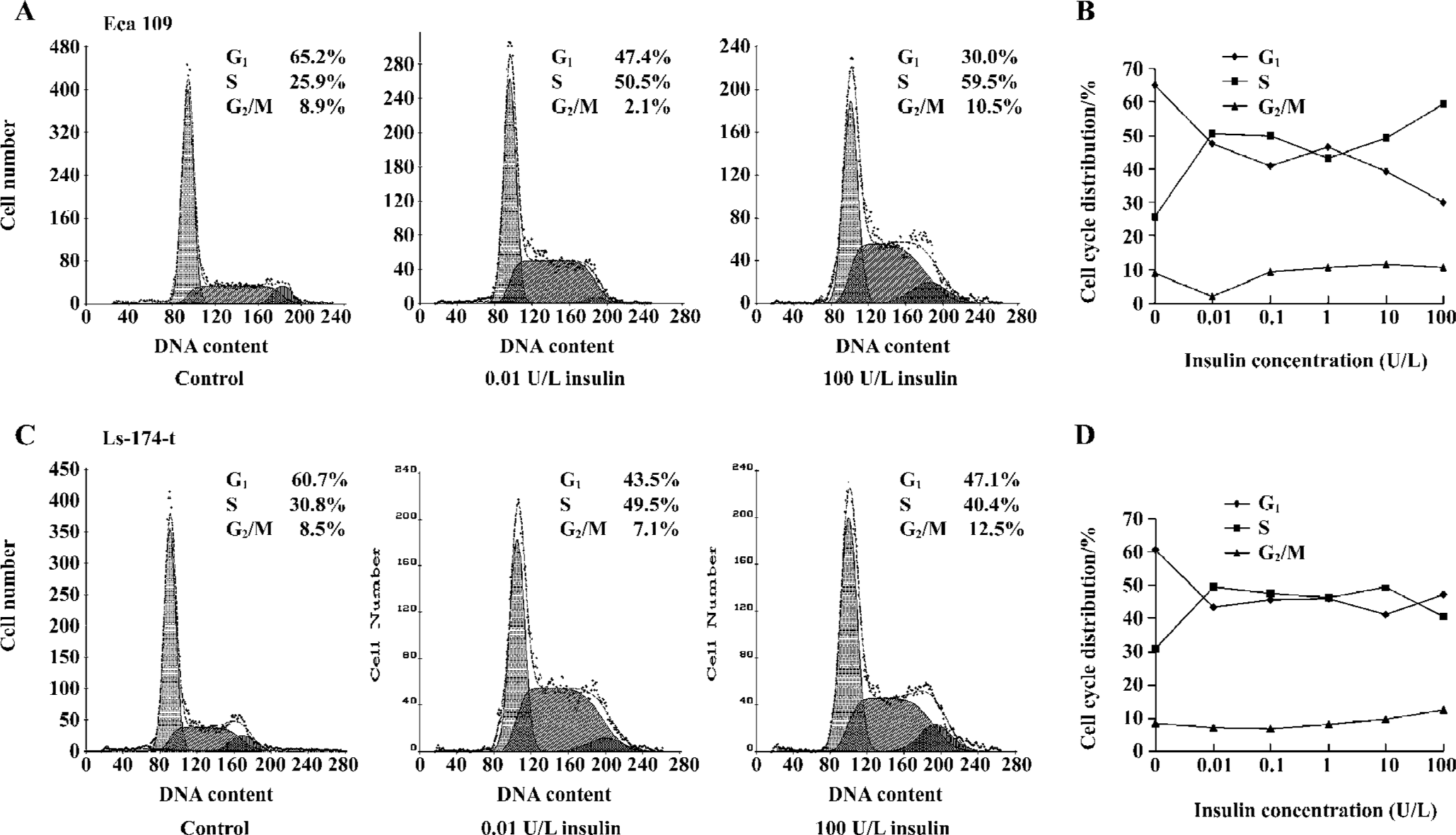
The tumor cells were exposed to insulin (1 U/L) for 2, 4, 8, 12, or 24 h, and then cell cycle distribution was examined. The percentage of the S phase cells increased significantly, accompanied by the decrease of the G1 and G2/M phase cells (Figure 3). The effect of insulin was time dependent in the first 4 h of exposure for Eca 109 cells or in the first 8 h of exposure for Ls-174-t cells, but there was no significant difference with the longer insulin treatment. Interestingly, the cell population of the G2/M phase gradually decreased as the treatment time increased.
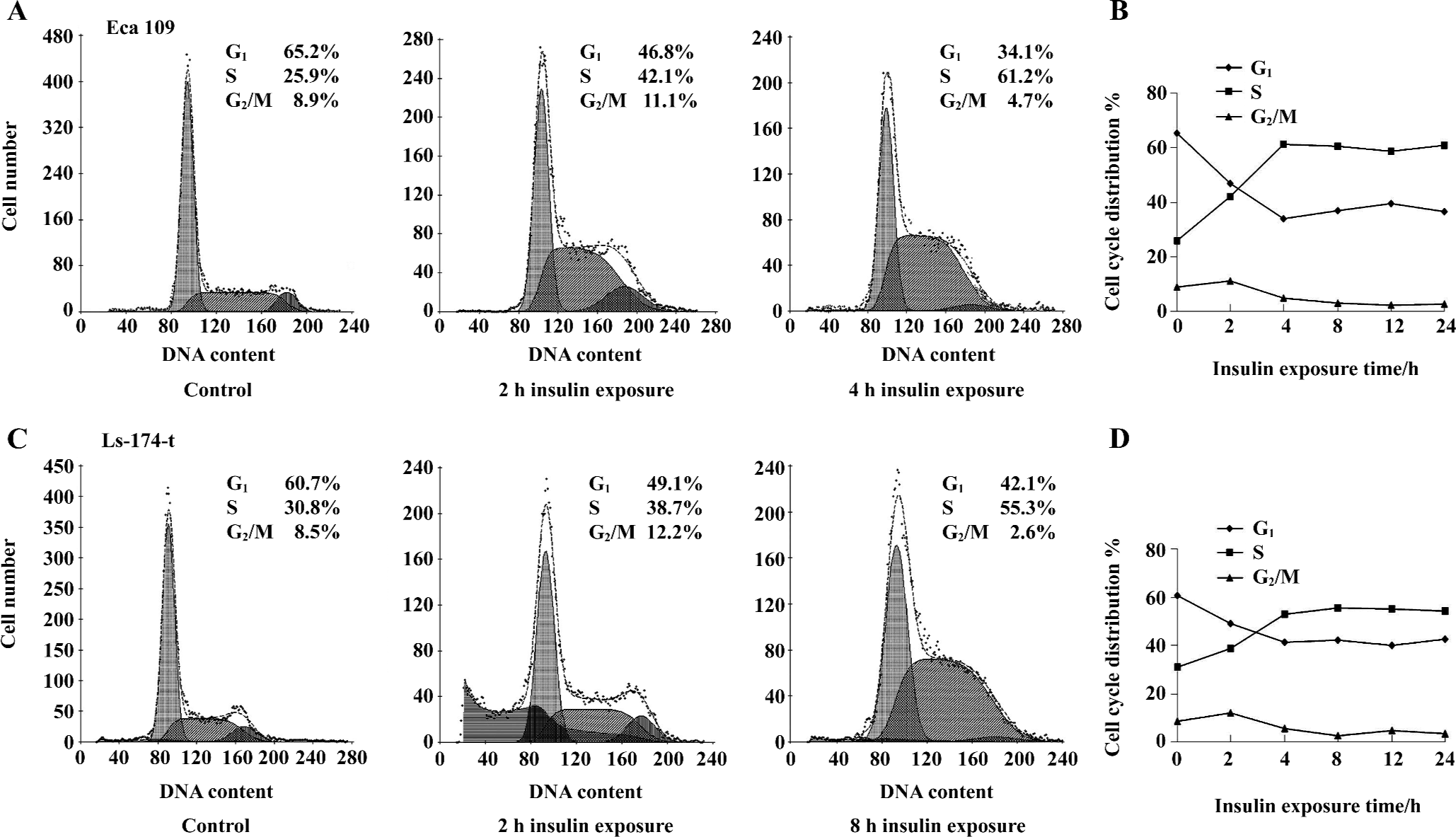
Insulin enhances the uptake of 5-FU in tumor cells In an effort to evaluate potential mechanisms, we performed HPLC assay and TS ternary complex detection to examine the uptake of 5-FU in the 2 tumor cell lines with or without insulin treatment. Examining the concentration of 5-FU in culture medium can indirectly analyze the total consumption of 5-FU by tumor cells and avoid the effect of drug metabolism and drug efflux via drug pumps at the cell membranes. In this study, the tumor cells were divided into 2 groups with the same cell number cultured in equal volumes of medium. One group was treated with 5-FU and the other was pretreated with insulin (4 h) followed by 5-FU exposure. The concentrations of 5-FU used for the Eca 109 and Ls-174-t cells were 25 µg/mL and 37 µg/mL, respectively. Then 200 µL culture medium was collected for HPLC analysis after 5-FU treatment for 6 h. In the Eca 109 cells, the 5-FU concentration of the insulin/5-FU group was 12.12±1.314 µg/mL, and in the 5-FU group it was 19.48±0.548 µg/mL. In the Ls-174-t cells, the 5-FU concentration in the insulin/5-FU group was 21.24±2.147 µg/mL, and in the 5-FU group it was 33.59±1.871 µg/mL (Figure 4).
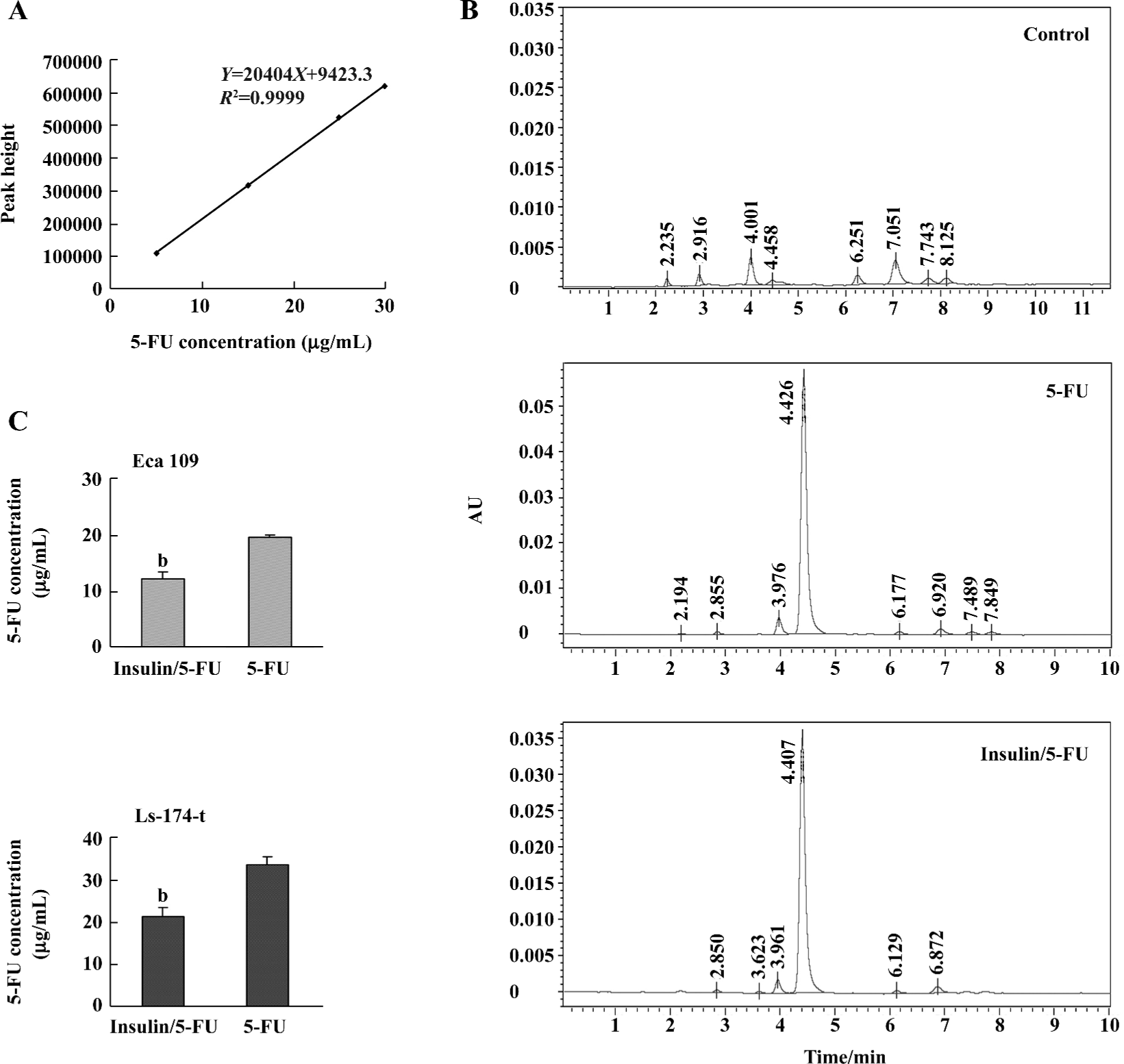
It is difficult to measure intracellular 5-FU concentration accurately because of the intracellular metabolizability itself. FdUMP, the metabolite of 5-FU, binds to TS and the folate cofactor 5-10-methylene tetrahydrofolate to form a complex in the tumor cells[6]. The increase of the TS ternary complexes reflects the intracellular augment of FdUMP. The uptake of 5-FU can be analyzed via the amount of FdUMP. In this study, the amount of the TS ternary complex increased in both tumor cell lines after insulin/5-FU treatment compared with 5-FU-alone treatment, which indicated the enhancement of 5-FU uptake (Figure 5).
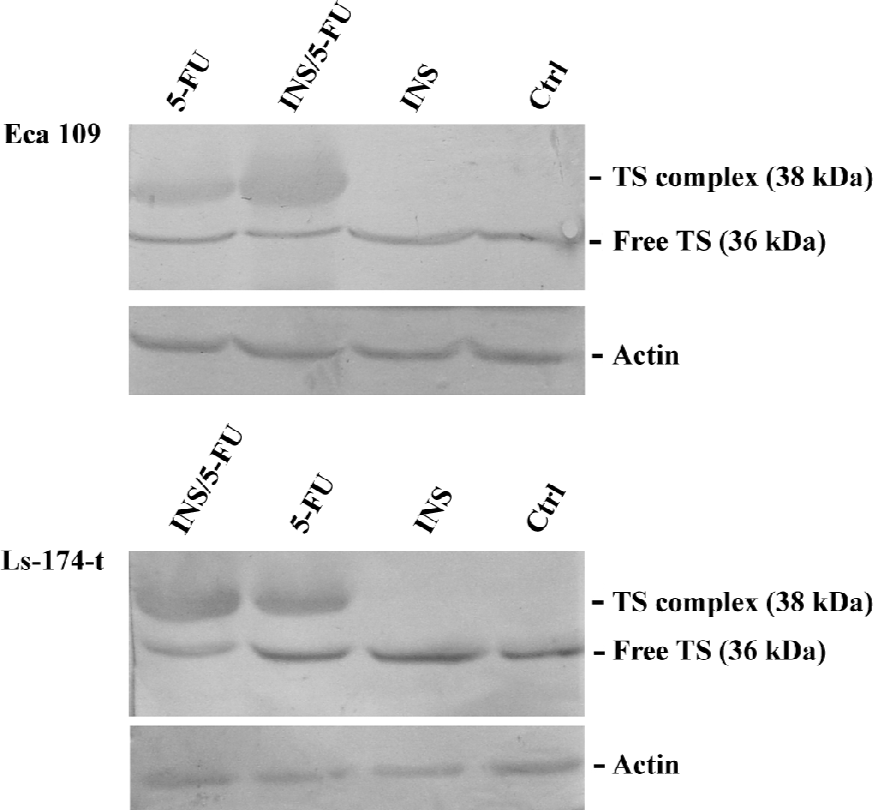
Effect of insulin/5-FU treatment on TS expression The expression of TS and the formation of the TS ternary complex was investigated by Western blotting. TS was basally expressed in both cell lines (Figure 5). 5-FU exposure induced the appearance of an additional band of 38 kDa (the TS ternary complex) and the increase of the total TS. Insulin treatment increased the TS expression slightly. For the Ls-174-t cells, densitometric analysis showed that, compared with 5-FU treatment, insulin/5-FU treatment induced the expression of the TS ternary complex with a 1.7-fold increase and decreased the free TS expression to 47%. The Eca 109 cells with insulin/5-FU treatment showed a 2.1-fold increase in the expression of the TS ternary complex compared with 5-FU treatment, while the level of free TS did not show obvious changes.
Insulin enhances 5-FU-induced apoptosis The combination of insulin and 5-FU produces a sequence-dependent apoptosis synergy. 5-FU is an S phase-specific anticancer drug. After 5-FU treatment for 48 h, only a few apoptotic cells were observed by flow cytometry analysis in both tumor cell lines. When the tumor cells were pretreated with insulin (0.01–100 U/L) for 4, 8, or 12 h followed by 5-FU treatment, the apoptotic population increased significantly compared with 5-FU treatment alone (Table 1). The apoptotic population increased in a dose-dependent manner when the concentration of insulin was below 1 U/L. However, when insulin and 5-FU were added at the same time, the statistical difference was not observed in all insulin concentration groups compared with the 5-FU control.
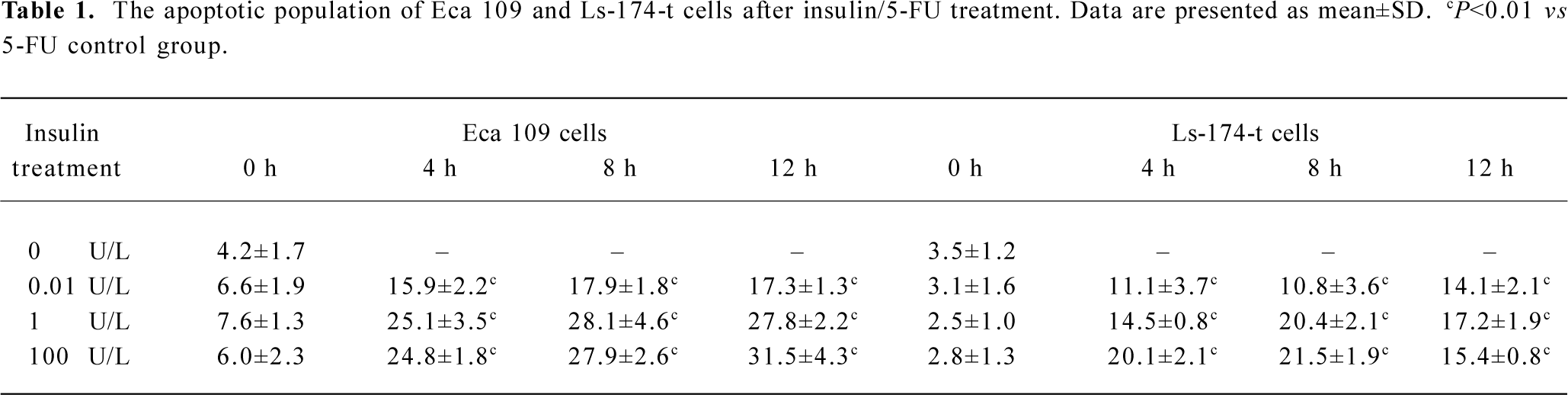
Full table
We also determined the apoptosis by TUNEL assay and DNA fragmentation. 1×107 cells were collected after 5-FU treatment or insulin/5-FU treatment. The result of the TUNEL assay showed that the number of apoptotic cells increased after insulin pretreatment in both cell lines (Figure 6A). Additionally, DNA fragments were observed in the Eca 109 cells, but not in the Ls-174-t cells (Figure 6B). In the Eca 109 cells, there was no obvious DNA fragment, but a dispersing strip was observed in the 5-FU treatment group. When the cells were pretreated with insulin before 5-FU, DNA fragments appeared.
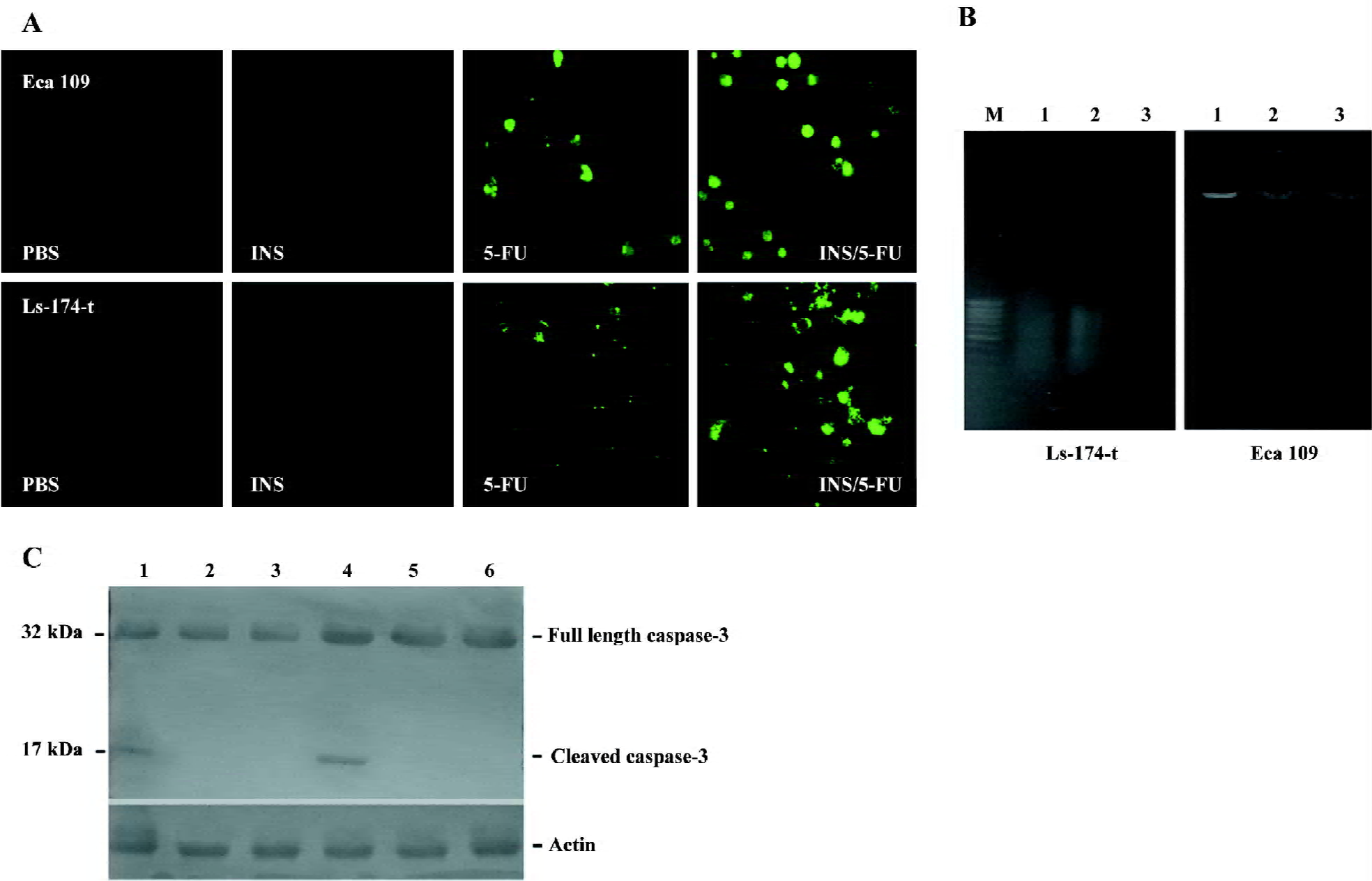
Furthermore, the expression of caspase-3 and its cleavage in Eca 109 and Ls-174-t cells were analyzed by Western blotting. The results showed that 5-FU treatment after insulin exposure for 8 h would increase the expression of cleaved caspase-3 compared with the 5-FU control (Figure 6C). The insulin/5-FU treatment resulted in an apoptotic response, indicating that the enhancement of the apoptotic response may be contributed to this synergistic interaction.
Discussion
Insulin is a polypeptide hormone with multiple roles[12]. As a drug, insulin is usually used in diabetes and some studies have found that it can also be used in cardiopathy treatment[19,20]. The effects of insulin on tumor therapy have not been fully investigated, and there are only few papers that mention the topic. Lasalvia-Prisco et al[21] reported that methotrexate and insulin produced a significant antitumoral response that was not seen with either methotrexate or insulin used separately in 30 patients with multidrug-resistant metastatic breast cancer. Jiao et al[22] demonstrated that insulin enhanced the chemotherapeutic sensitivity of cisplatin, etoposide, and 5-FU in the human esophageal cell line (NEC) and lung cancer cell line (GLC) by MTT assay and thought that the combination of insulin and 5-FU increased G2/M arrest. In this study, we were interested in the effects of insulin on the functions of 5-FU and the possible mechanism. We demonstrated that pretreatment with insulin can enhance the function of 5-FU on Eca 109 and Ls-174-t tumor cells. However, enhancement of G2/M arrest was not observed in Eca 109 and Ls-174-t cells after exposure to insulin/5-FU. In order to investigate the mechanisms in more depth, we examined the changes of cell cycle, intracellular uptake of 5-FU, cell apoptosis, and TS expression after treatment with insulin and insulin/5-FU, which, to our knowledge, have not been mentioned in previous studies.
Aspects of the cell cycle provide the rationale for and are of major importance in the successful application of antineoplastic chemotherapy[23]. In an effort to understand the mechanism that insulin enhances the antitumor effect of 5-FU, we assessed the cell cycle distribution after the tumor cells were treated with insulin or insulin/5-FU. Insulin markedly increased the cell population of the S phase while it decreased the cell number of the G0/G1 phase in Eca 109 and Ls-174-t cells. 5-FU is an S phase-specific agent, which exerts its lethal effects exclusively or primarily during the S phase[24]. Thus, after insulin pretreatment with the increasing cell number of the S phase, the anticancer effect of 5-FU is enhanced. Moreover, it is also important for insulin to reduce cell number of the G0/G1 phase during which dormant tumor cells can escape the effects of drug therapy[25]. Insulin treatment induces a reduction in ratio of cell of the G0/G1 phase and recruits cells into the S phase, which is beneficial to the cytotoxic effects of 5-FU. We conclude that insulin can enhance the function of 5-FU via increasing its target cells.
Insulin stimulates Na+, K+-ATPase activity, and translocation to the plasma membrane via phosphorylation of the alpha-subunits by the extracellular signal-regulated kinase (ERK) 1/2 mitogen-activated protein kinase[16,17]. Previous studies demonstrate that the uptake of 5-FU is apparently Na+-dependent[26]. In addition to Na+, the carrier-mediated transport of 5-FU may require some other factors that are yet to be identified[26]. We hypothesize that insulin may indirectly enhance the uptake of 5-FU in tumor cells via stimulating Na+, K+-ATPase activity. By HPLC analysis and TS ternary complex detection, we confirmed that the uptake of 5-FU was increased in Eca 109 and Ls-174-t cells after insulin exposure.
Resistance to apoptosis plays an important role in cancer pathogenesis[10,27]. It allows tumor cells to survive beyond their normally intended life spans and provide opportunities for selection of progressively aggressive clones. Inducement of apoptosis in tumor cells is one of the methods in improving chemotherapeutic effects[11,28]. 5-FU can trigger the intrinsic pathway to induce apoptosis[29]. Apoptosis obviously occurs after tumor cells are exposed to higher concentration of 5-FU for a long time. However, in our study, the results showed that with insulin pretreatment, 5-FU induced more tumor cells to apoptosis compared with 5-FU-alone treatment. Moreover, caspase-3 assay demonstrated that insulin/5-FU treatment upregulated the activation of caspase-3 in the 2 tumor cell lines. We conclude that insulin enhances the apoptotic effect of 5-FU by increasing the number of sensitive cells and the intracellular concentration of 5-FU.
TS, which converts dUMP to dTMP, is the only de novo source of intracellular thymidylate for DNA synthesis[30]. Inhibition of the enzyme results in chromosome breaks and cell death[31]. TS is an important target for 5-FU. Cells metabolize 5-FU to FdUMP and FUTP. FdUMP and the folate cofactor 5-10-methylene tetrahydrofolate bind to TS to form a covalently-bound ternary complex that inhibits TS[32]. The expression level of TS among tumors varies considerably, and cellular sensitivity to 5-FU is related to alterations in the TS level[33]. In our study, insulin/5-FU treatment increased the level of the TS ternary complex and decreased the free TS expression compared with 5-FU treatment in the Ls-174-t cells. For the Eca 109 cells, we just observed the enhancement of the TS ternary complex. It indicated that the responses to the insulin/5-FU treatment existed differently between Eca 109 and Ls-174-t cells. In clinics, patients with low tumor TS expression have a significantly better prognosis than those with high TS expression[34]. We consider that the decrease of the free TS level after insulin/5-FU treatment has clinical significance to some extent. Moreover, the increase of the TS ternary complex also is beneficial for tumor therapy.
In summary, our data suggest that insulin enhances the chemotherapeutic effect of 5-FU in Eca 109 and Ls-174-t cells. Insulin increases the cell number of the S phase and the uptake of 5-FU. Insulin/5-FU treatment enhances apoptosis of tumor cells and changes TS and the TS ternary complex levels compared with 5-FU treatment. A more detailed understanding of mechanisms by which insulin improves the chemosensitivity of 5-FU may lead to new strategies for the clinical chemotherapy of tumors.
Acknowledgements
We thank Prof Shi-xin LU (Cancer Institute Chinese Academy of Medical Sciences, Beijing, China) for thoughtful discussions during the course of this study, Zhu TAN (Institute of Biochemistry and Cell Biology, Chinese Academy of Sciences, Shanghai, China) for assistance with flow cytometry, and Xu XU (Institute of Organic Chemistry, Chinese Academy of Sciences, Shanghai, China) for expert help with HPLC.
References
- Paradiso A, Xu J, Mangia A, Chiriatti A, Simone G, Zito A, et al. Topoisomerase-I, thymidylate synthase primary tumour expression and clinical efficacy of 5-FU/CPT-11 chemotherapy in advanced colorectal cancer patients. Int J Cancer 2004;111:252-8.
- Fryar EB, Das JR, Davis JH, Desoto JA, Laniyan I, Southerland WM, et al. Raloxifene attenuation of 5-FU/methotrexate cytotoxicity in human breast cancer cells: the importance of sequence in combination chemotherapy. Anticancer Res 2006;26:1861-7.
- Prevost A, Merol JC, Aime P, Moutel K, Roger-Liautaud F, Nasca S, et al. A randomized trial between two neoadjuvant chemotherapy protocols: CDDP+5-FU versus CDDP+VP16 in advanced cancer of the head and neck. Oncol Rep 2005;14:771-6.
- Fukushima M, Fujioka A, Uchida J, Nakagawa F, Takechi T. Thymidylate synthase (TS) and ribonucleotide reductase (RNR) may be involved in acquired resistance to 5-fluorouracil (5-FU) in human cancer xenografts in vivo. Eur J Cancer 2001;37:1681-7.
- van Kuilenburg AB. Dihydropyrimidine dehydrogenase and the efficacy and toxicity of 5-fluorouracil. Eur J Cancer 2004;40:939-50.
- Longley DB, Harkin DP, Johnston PG. 5-fluorouracil: mechanisms of action and clinical strategies. Nat Rev Cancer 2003;3:330-8.
- Loeffler M, Kruger JA, Niethammer AG, Reisfeld RA. Targeting tumor-associated fibroblasts improves cancer chemotherapy by increasing intratumoral drug uptake. J Clin Invest 2006;116:1955-62.
- Gottesman MM. Mechanisms of cancer drug resistance. Annu Rev Med 2002;53:615-27.
- Shah MA, Schwartz GK. Cell cycle-mediated drug resistance: an emerging concept in cancer therapy. Clin Cancer Res 2001;7:2168-81.
- Pommier Y, Sordet O, Antony S, Hayward RL, Kohn KW. Apoptosis defects and chemotherapy resistance: molecular interaction maps and networks. Oncogene 2004;23:2934-49.
- Makin G, Dive C. Modulating sensitivity to drug-induced apoptosis: the future for chemotherapy? Breast Cancer Res 2001;3:150-3.
- De Meyts P. Insulin and its receptor: structure, function and evolution. Bioessays 2004;26:1351-62.
- Furtado LM, Somwar R, Sweeney G, Niu W, Klip A. Activation of the glucose transporter GLUT4 by insulin. Biochem Cell Biol 2002;80:569-78.
- Acevedo CG, Marquez JL, Rojas S, Bravo I. Insulin and nitric oxide stimulates glucose transport in human placenta. Life Sci 2005;76:2643-53.
- Gu S, Villegas CJ, Jiang JX. Differential regulation of amino acid transporter SNAT3 by insulin in hepatocytes. J Biol Chem 2005;280:26055-62.
- Al-Khalili L, Kotova O, Tsuchida H, Ehren I, Feraille E, Krook A, et al. ERK1/2 mediates insulin stimulation of Na(+),K(+)-ATPase by phosphorylation of the alpha-subunit in human skeletal muscle cells. J Biol Chem 2004;279:25211-8.
- Rosic NK, Standaert ML, Pollet RJ. The mechanism of insulin stimulation of (Na+,K+)-ATPase transport activity in muscle. J Biol Chem 1985;260:6206-12.
- Xu JM, Song ST, Tang ZM, Liu XQ, Jiang ZF, Zhou L, et al. Evaluation of in vitro chemosensitivity of antitumor drugs using the MTT assay in fresh human breast cancer. Breast Cancer Res Treat 1998;49:251-9.
- Lautamaki R, Airaksinen KE, Seppanen M, Toikka J, Harkonen R, Luotolahti M, et al. Insulin improves myocardial blood flow in patients with type 2 diabetes and coronary artery disease. Diabetes 2006;55:511-6.
- Sauer WH, Cappola AR, Berlin JA, Kimmel SE. Insulin sensitizing pharmacotherapy for prevention of myocardial infarction in patients with diabetes mellitus. Am J Cardiol 2006;97:651-4.
- Lasalvia-Prisco E, Cucchi S, Vazquez J, Lasalvia-Galante E, Golomar W, Gordon W. Insulin-induced enhancement of antitumoral response to methotrexate in breast cancer patients. Cancer Chemother Pharmacol 2004;53:220-4.
- Jiao SC, Huang J, Sun Y, Lu SX. The effect of insulin on chemotherapeutic drug sensitivity in human esophageal and lung cancer cells. Zhonghua Yi Xue Za Zhi 2003;83:195-7. Chinese..
- O’Connor PM, Kohn KW. A fundamental role for cell cycle regulation in the chemosensitivity of cancer cells? Semin Cancer Biol 1992;3:409-16.
- Boumah CE, Setterfield G, Kaplan JG. Purine and pyrimidine analogues irreversibly prevent passage of lymphocytes from the G1 to the S phase of the cell cycle. Can J Biochem Cell Biol 1984;62:280-7.
- Jedema I, Barge RM, Nijmeijer BA, Willemze R, Falkenburg JH. Recruitment of leukemic cells from G0 phase of the cell cycle by interferons results in conversion of resistance to daunorubicin. Leukemia 2003;17:2049-51.
- Yuasa H, Matsuhisa E, Watanabe J. Intestinal brush border transport mechanism of 5-fluorouracil in rats. Biol Pharm Bull 1996;19:94-9.
- Hickman JA. Apoptosis and chemotherapy resistance. Eur J Cancer 1996;32A:921-6.
- Amantana A, London CA, Iversen PL, Devi GR. X-linked inhibitor of apoptosis protein inhibition induces apoptosis and enhances chemotherapy sensitivity in human prostate cancer cells. Mol Cancer Ther 2004;3:699-707.
- Adachi Y, Taketani S, Oyaizu H, Ikebukuro K, Tokunaga R, Ikehara S. Apoptosis of colorectal adenocarcinoma induced by 5-FU and/or IFN-gamma through caspase 3 and caspase 8. Int J Oncol 1999;15:1191-6.
- Yang Z, Cloud A, Hughes D, Johnson LF. Stable inhibition of human thymidylate synthase expression following retroviral introduction of an siRNA gene. Cancer Gene Ther 2006;13:107-14.
- Curtin NJ, Harris AL, Aherne GW. Mechanism of cell death following thymidylate synthase inhibition: 2'-deoxyuridine-5'-triphosphate accumulation, DNA damage, and growth inhibition following exposure to CB3717 and dipyridamole. Cancer Res 1991;51:2346-52.
- Mandel HG. The target cell determinants of the antitumor actions of 5-FU: does FU incorporation into RNA play a role? Cancer Treat Rep 1981;65 Suppl 3:63-71.
- Ota D, Kusama M, Kaise H, Nakayama S, Misaka T, Tsuchida A, et al. Evaluation of sensitivity to 5-FU on the basis of thymidylate synthase (TS)/dihydropyrimidine dehydrogenase (DPD) activity and chromosomal analysis in micro tissue specimens of breast cancer. Breast Cancer 2004;11:356-66.
- Van Triest B, Peters GJ. Thymidylate synthase: a target for combination therapy and determinant of chemotherapeutic response in colorectal cancer. Oncology 1999;57:179-94.