Hypertonic and isotonic potassium solutions have different effects on vessel contractility resulting in differences in optimal resting tension in rat aorta1
Introduction
High K+-induced contraction is often used to examine the smooth muscle contraction function, to standardize the receptor-mediated contraction, and to determine the optimal resting tension in isolated blood vessel study[1–10]. There are 2 commonly used techniques to produce high K+: hyperosmolar addition of KCl (hypertonic K+) and iso-osmolar substitution of NaCl with KCl (isotonic K+)[2]. It has been reported that Ca2+ channel blockers may have differential relaxation effects on hypertonic and isotonic K+-induced contractions[7]. Moreover, direct observations suggest different contractions induced by hypertonic and isotonic K+ in rat aortas[2] and middle cerebral arteries[3]. However, it is unknown the differences between contractions induced by hypertonic and isotonic K+ at different resting tensions, and whether the differences may lead to different values of optimal resting tension measured by hypertonic and isotonic K+. Therefore, the current study compared the contractions and optimal resting tensions determined by 2 different high K+ solutions at increasing resting tensions. Two different kinds of aortic preparations, in which perivascular adventitial fat was either removed or left intact[5] from 3 different ages of rats (15, 25, and 62 weeks) were used for the com-parisons.
Materials and methods
Animals Male Sprague-Dawley rats were purchased from the Sino-British SIPPR/BK Lab Animal Ltd (Shanghai, China) at the age of 6 weeks and brought up in our animal house until the age for experiments (15, 25, and 62 weeks, respec-tively). They were housed in controlled temperature (23–25 °C) and lighting (8:00–20:00), and with free access to tap water and rat chow. All experimental procedures were performed in accordance with institutional guidelines for animal care.
Solutions Krebs-Henseleit solution was made using the following composition (in mmol/L): NaCl 118.4, KCl 4.7, CaCl2 2.5, MgSO4 1.2, KH2PO4 1.2, NaHCO3 25.0, glucose 11.1, and CaNa2-EDTA 0.026. Isotonic solution of high K+ (30 mmol/L) was made by iso-osmolar substitution of NaCl with KCl for Krebs-Henseleit solution and therefore it contained the following composition (in mmol/L): NaCl 93.1, KCl 30.0, CaCl2 2.5, MgSO4 1.2, KH2PO4 1.2, NaHCO3 25.0, glucose 11.1, and CaNa2-EDTA 0.026.
Aortic preparations The rat was anesthetized with sodium pentobarbital (60 mg/kg, ip). After opening the thoracic cavity, the descending thoracic aorta was immediately removed into cold Krebs-Henseleit solution aerated with 95% O2 and 5% CO2, and dissected into 5 mm-wide rings using a ruler. Four aortic rings were obtained from each rat to make 2 kinds of aortic preparations in which adventitial fat was either removed or left intact.
Determination of high K+-induced contractions at increasing resting tensions The aortic rings were suspended in conventional organ baths filled with 20 mL Krebs-Henseleit solution maintained at 37 °C and continuously aerated with 95% O2 and 5% CO2. Changes in isometric tension were recorded with IT1-25 transducers and IOX computerized system (EMKA Technologies, Paris, France)[6]. Each aortic ring was allowed to equilibrate for 60 min at a resting tension of 1.0 g and was then exposed to high K+ (30 mmol/L). When the high K+-induced contraction reached a plateau, the rings were rinsed with Krebs-Henseleit solution and allowed to re-equilibrate for 30 min at an increased resting tension of 1.5 g. Thereafter, the addition of high K+ (30 mmol/L), the recording of isometric contraction, and the washing with Krebs-Henseleit solution were repeated as described earlier. Increasingly, the resting tension was adjusted to 2.0, 2.5, 3.0, or 3.5 g. At each resting tension, the maximal contraction induced by high K+ (30 mmol/L) was measured (Figure 1). The optimal resting tension was obtained from the resting tension-contraction curve for each aorta and defined as the resting tension at which the aorta showed a maximal contraction[4].
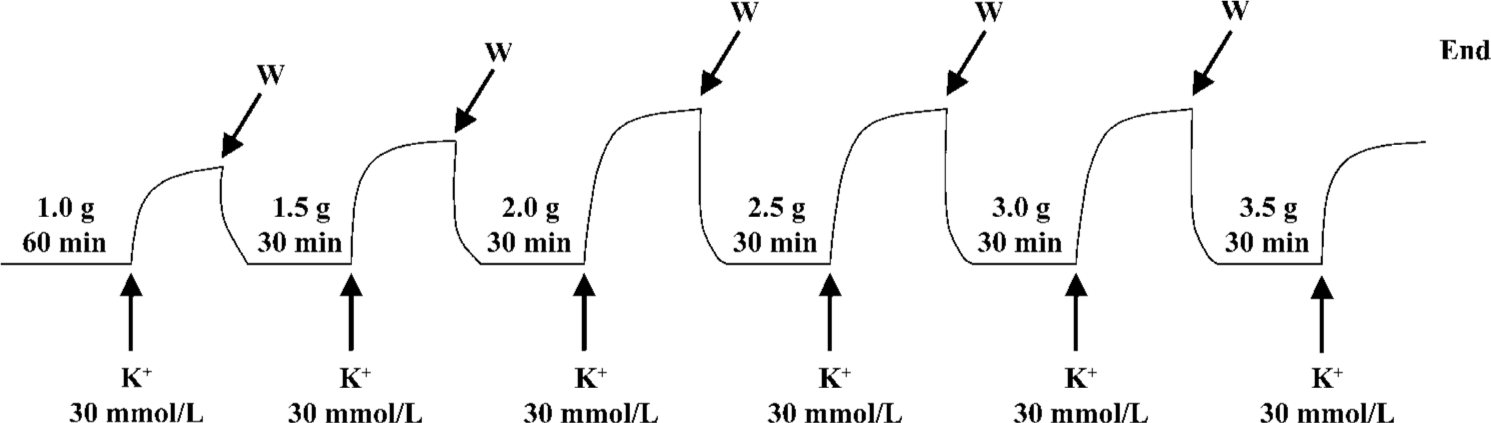
In the present study, high K+ (30 mmol/L)-induced contraction was produced by 2 commonly used techniques in the separate experiments: (i) hypertonic K+ (30 mmol/L)-induced contraction was produced by adding KCl to organ bath containing Krebs-Henseleit solution, the total concentration of KCl in the bath was 30 mmol/L; and (ii) isotonic K+ (30 mmol/L)-induced contraction was produced by replacing Krebs-Henseleit solution in organ bath with isotonic solution of high K+.
To examine a possible mechanical perturbation caused by the replacement of solution, we compared the tensions obtained before and after the change of Krebs-Henseleit solution using the same time course at the different resting tensions mentioned earlier. It was found that the change of solution did not affect the tensions (data not shown).
Statistical analysis Data are reported as means±SEM. Statistical analysis was performed with ANOVA followed by two-tailed Student’s unpaired t-test. The threshold for statistical significance was P<0.05.
Results
Rats at 3 different ages (15, 25, and 62 weeks) were used for the present study; body weight increased with age (Table 1).
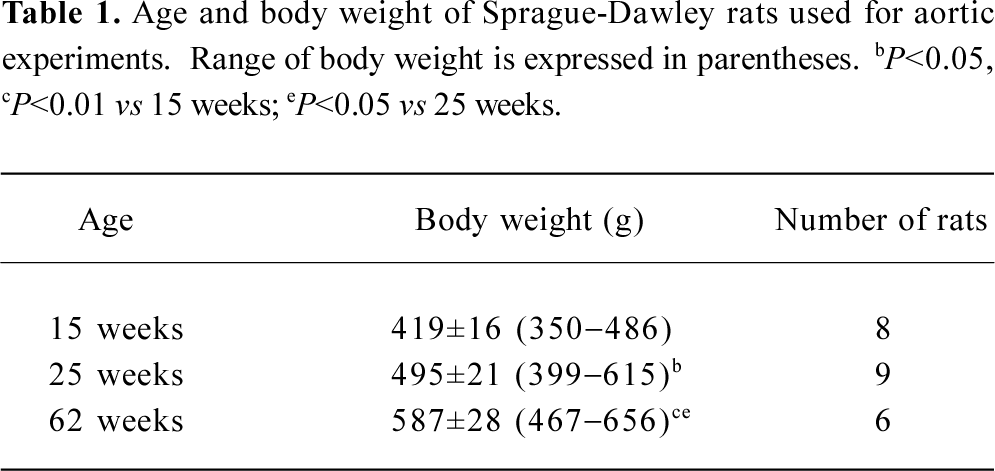
Full table
The aortic contractions induced by 2 kinds of high K+ were significantly different in all of the age groups examined (Figure 2). The different responses to hypertonic and isotonic K+ were age related. At the age of 15 weeks, isotonic K+-induced contractions were either greater or tended to be greater at almost all resting tensions when compared with hypertonic K+-induced contractions. The resting tension-contraction curve was up for isotonic K+, but down for hypertonic K+ (Figure 2A). However, at the age of 62 weeks, isotonic K+-induced contractions either were smaller or tended to be smaller at almost all resting tensions when compared with hypertonic K+-induced contractions. The resting tension-contraction curve was down for isotonic K+, but up for hypertonic K+ (Figure 2C). The results in the 25-week-old groups were between the 15- and 62-week-old groups; isotonic K+-induced contractions either were smaller or tended to be smaller at lower resting tensions from 1.0 to 2.0 g, but were either greater or tended to be greater at higher resting tensions from 2.5 to 3.5 g when compared with hypertonic K+-induced contractions. Two resting tension-contraction curves for isotonic and hypertonic K+ were crossed (Figure 2B).
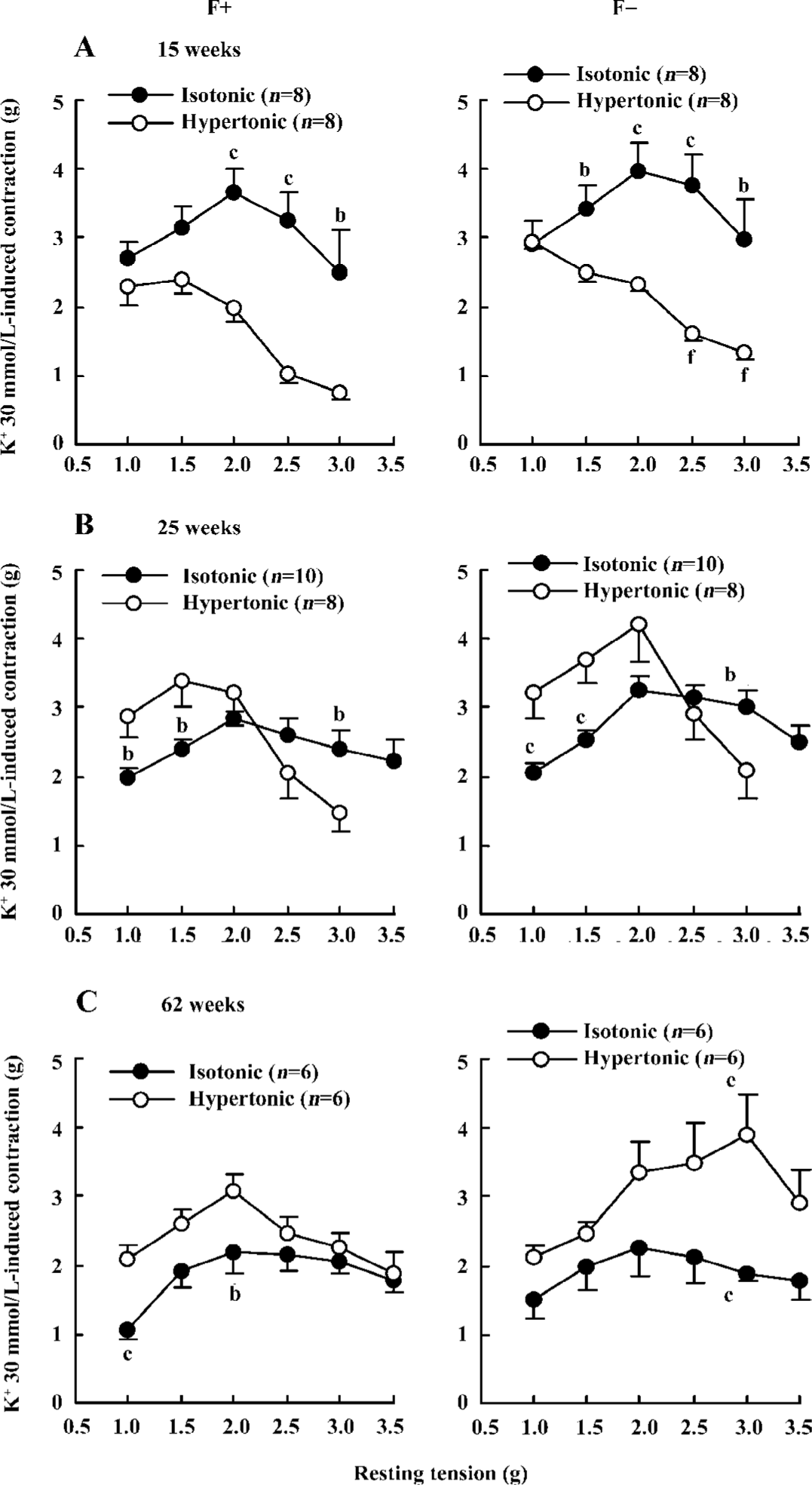
The optimal resting tensions determined by 2 kinds of high K+ were inconsistent (Figure 2; Table 2). Optimal resting tensions measured by isotonic K+ in different kinds of aortas from various age rats were almost identical; they were a constant of 2 g. However, optimal resting tensions measured by hypertonic K+ in different kinds of aortas from various age rats were changeable; they were a variable.
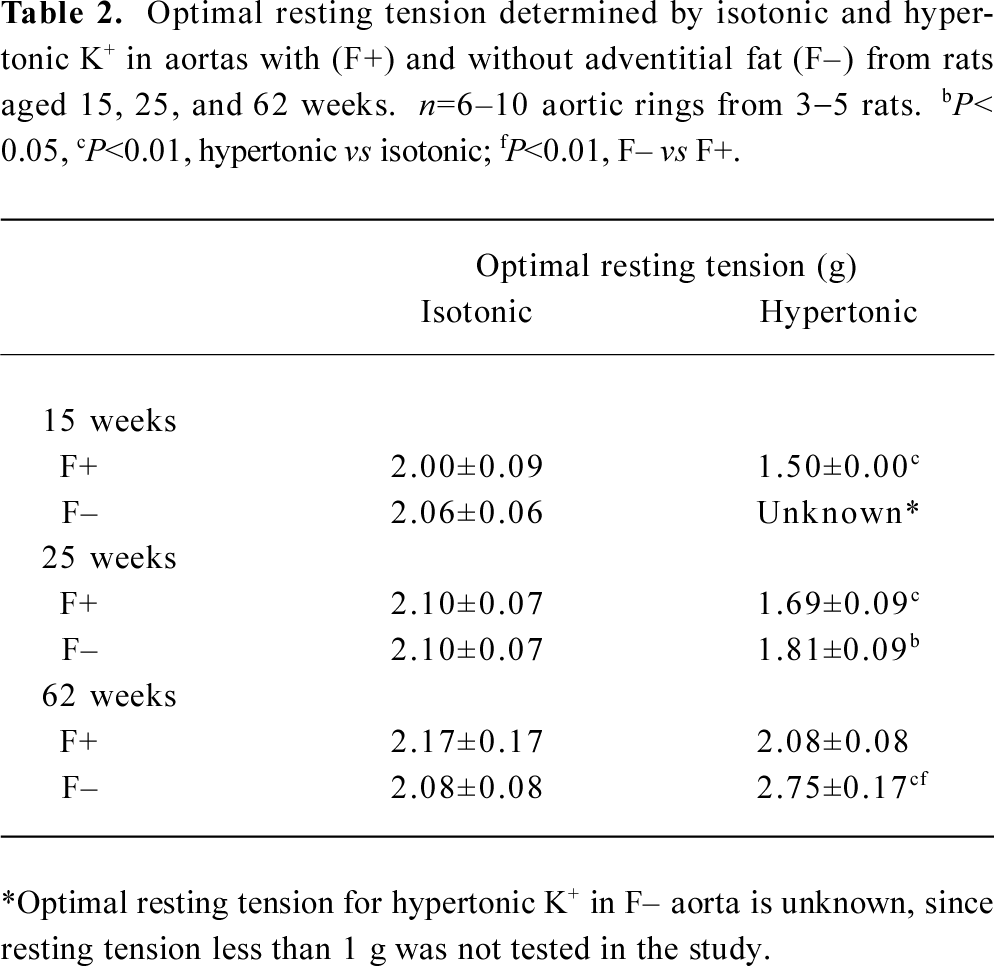
Full table
The effects of adventitial fat on high K+-induced contractions were evaluated by comparisons between aortas with and without adventitial fat. For isotonic K+-induced contractions, there were no significant differences between aortas with and without adventitial fat (Figure 2). For hypertonic K+-induced contractions, only a few points in the 15- and 62-week-old groups showed a difference between aortas with and without adventitial fat, but most points did not show the differences (Figure 2). Although the adventitial fat had little effect on the maximal contractions induced by high K+ as described earlier and demonstrated in Figure 2, the adventitial fat always delayed the development of high K+-induced contractions. The time at which high K+ induced half-maximal contractions is summarized in Table 3; it was significantly longer in aortas with adventitial fat than in aortas without adventitial fat for both hypertonic and isotonic K+ solutions at different resting tensions in all age groups. The representative original recordings are shown in Figure 3.
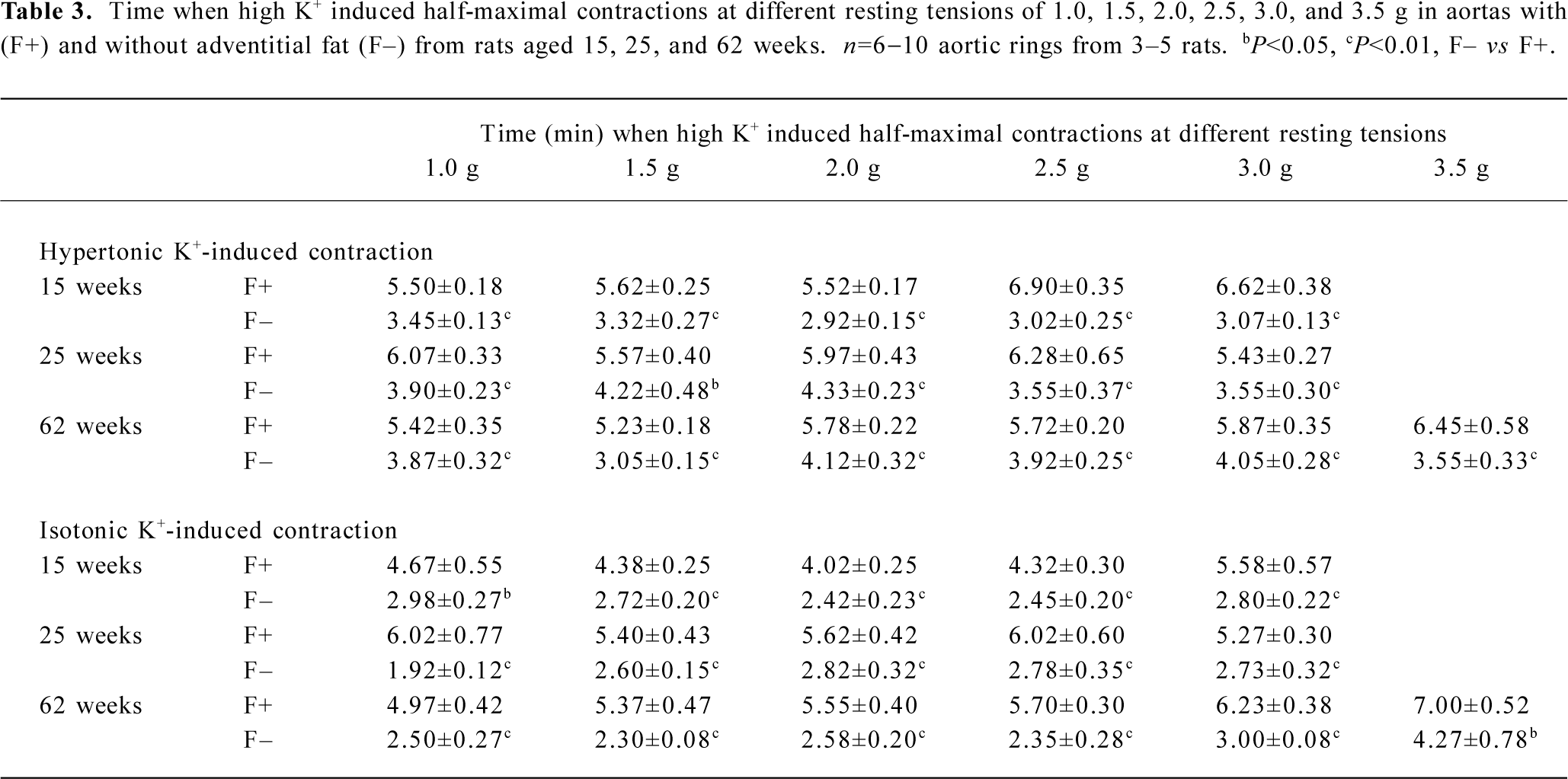
Full table
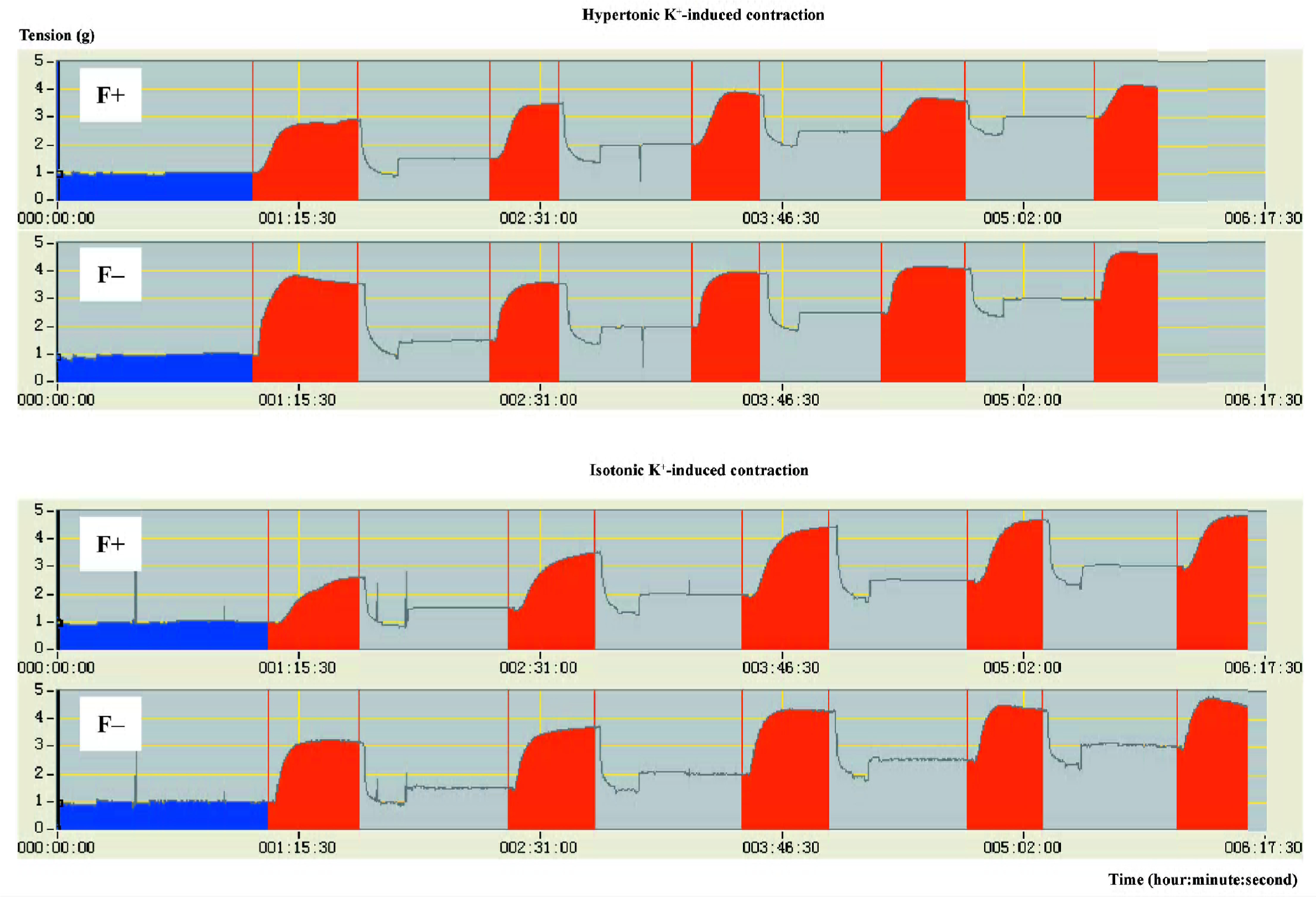
Discussion
The main finding of the present study is that there exists big differences between aortic contractions induced by hypertonic and isotonic K+ at different resting tensions, and these differences make the optimal resting tension values inconsistent when measured by the 2 kinds of high K+.
The mechanisms for the different responses to hypertonic and isotonic K+ seem complex. It is well established that in most smooth muscle, high K+ evokes contraction via an elevation of cytosolic Ca2+ through the dihydropyridine-sensitive Ca2+ channel (L-type Ca2+ channel)[2]. Recent studies show that high K+ can also cause Ca2+ sensitization involving the translocation and activation of the RhoA kinase[8]. In contrast to isotonic K+, hypertonic K+ causes contraction not only via the L-type Ca2+ channel, but also by hyperosmotic action as well as membrane enzyme activation. For osmotic action, it is well known that alterations in osmolarity may affect muscle cell volume and membrane potential[11,12]. Both hyperosmotic and hyposmotic solutions can change vascular tone. For example, hyperosmotic solution induced contractions in rat and guinea-pig aortas[2] and dilation in rat middle cerebral arteries[3] and skeletal muscle arteries[13]. Hyposmotic solution induced contractions in rat tail arteries[14]. For enzymatic action, one study demonstrated that the dihydropyridine-insensitive component of hypertonic K+-induced contraction in Ca2+-free medium was sensitive to protein kinase C inhibitors, H7 and calphostin C[2]. The results suggested the activation of Ca2+-independent isozyme of protein kinase C was involved in the hypertonic K+-induced contraction[2,15]. In addition, it should be noted whether the factor of low Na+ in the isotonic K+ solution may influence its effect. Previous studies showed that in rat aortas without endothelium, increasing Na+ by the addition of 1–30 mmol/L NaCl to Krebs’ solution did not affect the resting tone, but it changed the phenylephrine-induced contraction. Increasing Na+ by the addition of 1, 3, and 6 mmol/L NaCl increased the phenylephrine-induced contraction, whereas further increasing Na+ by the addition of 10, 20, and 30 mmol/L NaCl inhibited the phenylephrine-induced contraction[16]. Simultaneous Mg2+ withdrawal and Na+ reduction (to 84 mmol/L) by replacement of NaCl with isosmolar amounts of sucrose in normal Krebs-Ringer bicarbonate induced significant increases of basal tone of denuded rat aortic rings[17]. The reduction of both Na+ and Cl– caused hyperpolarization of hamster aortic endothelial cells, indicating the essential role of endothelial cells in mediating vascular functions[18]. According to our own results in the present study, we can not make any conclusions for the effect of low Na+ on the isotonic K+-induced contractility. Further experiments need to be performed in the future.
The 2 kinds of high K+ examined in the present study are commonly used in vascular studies[1–10,19,20]. Some laboratories usually use hypertonic K+, which is very easy to produce and manipulate, and others use isotonic K+, a relatively complicated technique. It should be kept in mind when one reads the literature reports that hypertonic and isotonic K+ may induce different results. The previous data and the present study indicate that isotonic K+ is superior to hypertonic K+ for most of vascular studies, unless hypertonic K+ is absolutely necessary for the experiments; for instance, both high K+ and high osmolarity are needed to mimic the pathological conditions after traumatic brain injury[3]. The viewpoint that isotonic K+ is superior to hypertonic K+ for most vascular studies is based on the following 3 reasons. First, isotonic/iso-osmotic solution tallies with the actual physiological situation. Second, the mechanisms for isotonic K+-induced contraction are relatively simple compared with those for hypertonic K+-induced contraction. Third, the optimal resting tensions measured by isotonic K+ are almost identical. They are a constant of 2 g in different kinds of aortic preparations from various age rats. This point is very important for the comparisons under the same condition of resting tension in vascular study. In contrast, the variable values of optimal resting tensions measured by hypertonic K+ mean that different resting tensions should be used for various rat aortas, possibly causing inappropriate results.
We measured high K+-induced contraction at different resting tensions in aortic rings from 15- to 62-week-old rats. The resting tension-contraction curve for isotonic K+ was downshifted with age, while the pattern of the curves was similar and the value of the measured optimal resting tensions was almost the same in the different age groups. These results indicate that the ability of the contraction was easily affected by the factor of age, while the optimal resting tension, a property of muscle cell, was relatively constant, at least in the examined age range from 15 to 62 weeks.
The present study provides detailed data on high K+-induced contraction and optimal resting tension not only in a wide range of rat age from 15 to 62 weeks, but also in 2 different kinds of aortic preparations in which adventitial fat is either removed or left intact. This is because in most previous isolated artery studies, the adventitial fat is removed routinely and this manipulation neglects the potential role of adventitial fat. However, since 2002, several studies have reported the role of adventitial fat in the regulation of arterial tone in rat aortas[5,21,22] and mesenteric arteries[23] as well as in human internal thoracic arteries[24]. The effects of adventitial fat on high K+-induced contraction should be evaluated in detail. Our results showed that the adventitial fat could delay the development of high K+-induced contractions at different resting tensions, but had little effect on the maximal contractions in response to high K+.
Over the last 25 years, the importance of endothelium in the regulation of vascular tone has become apparent[25–27]. It is well known that the intimal endothelial cells can produce and release a variety of vasoactive substances to modulate medial smooth muscle contraction and relaxation. The endothelium has inhibitory effect on high K+-induced contraction. Basal release of endothelial factor(s), such as NO from rat aortic endothelium, may have a relaxing effect on smooth muscle, thus reducing the agonist-induced contraction[6]. It is also reported that vascular smooth muscle can influence endothelium through myo-endothelial junctions (gap junctions)[26]. Increases in Ca2+ in the vascular smooth (which occur with extracellular K+ concentrations above 20−30 mmol/L) can be transmitted to the endothelium via the myo-endothelial junctions. Increased Ca2+ in the endothelium may stimulate the release of NO.
The potential role of adventitial fat in the regulation of arterial tone was explored recently. Regarding the interaction between adventitial fat and smooth muscle, some studies including our unpublished experiments demonstrated that adventitial fat could inhibit the contraction induced by several agonists, such as serotonin, phenylephrine, angiotensin II, and endothelin[5,21–24]. In addition, the bioassay experiments revealed that adventitial fat could release a relaxing factor, named as adventitium- or adipocyte-derived relaxing factor (ADRF)[5,21]. Our results that adventitial fat had little effect on the maximal contraction in response to high K+ are consistent with the previous data[5,23], indicating the inhibitory effect of adventitial fat on vascular contraction is agonist specific. The slower development of high K+-induced contraction in aortas with adventitial fat than in aortas without adventitial fat was possibly due to the concomitant secretion of ADRF from adventitial fat. The effect of ADRF is dependent on opening of K+ channels[5,23] which initially suppressed the development of high K+-induced contraction, but eventually this effect was abolished by high concentration of extracellular K+[1].
In summary, our experiments provide new evidence about differential effects of hypertonic and isotonic K+ on aortic contraction. The optimal resting tensions in different kinds of aortic preparations from various age rats are almost a constant determined by isotonic K+, but a variable determined by hypertonic K+. To our knowledge, this is the first study to determine the optimal resting tensions in aortas with adventitial fat, which is meaningful for the future work on the complex interactions between vascular layers, especially the study of the paracrine effects of adventitial fat on vascular tone[28,29].
References
- Gollasch M, Bychkov R, Ried C, Behrendt F, Scholze S, Luft FC, et al. Pinacidil relaxes porcine and human coronary arteries by activating ATP-dependent potassium channels in smooth muscle cells. J Pharmacol Exp Ther 1995;275:681-92.
- Low AM, Loke JCP, Kwan CY, Daniel EE. Sensitivity to protein kinase C inhibitors of nicardipine-insensitive component of high K+ contracture in rat and guinea-pig aorta. Br J Pharmacol 1994;112:604-10.
- Golding EM, Steenberg ML, Johnson TD, Bryan RM Jr. The effects of potassium on the rat middle cerebral artery. Brain Res 2000;880:159-66.
- Gonzalez MC, Arribas SM, Molero F, Fernandez-Alfonso MS. Effect of removal of adventitia on vascular smooth muscle contraction and relaxation. Am J Physiol Heart Circ Physiol 2001;280:H2876-81.
- Lohn M, Dubrovska G, Lauterbach B, Luft FC, Gollasch M, Sharma AM. Periadventitial fat releases a vascular relaxing factor. FASEB J 2002;16:1057-63.
- Miao CY, Villeneuve N, Brunel-Jacquemin C, Petit C, Guillaumin JP, Gransagne D, et al. Chronic mild hyperhomocysteinemia induces aortic endothelial dysfunction but does not elevate arterial pressure in rats. J Vasc Res 2005;42:148-56.
- Nielsen-Kudsk JE, Nielsen CB, Mellemkjaer S. Influence of osmolarity of solutions used for K+ contraction on relaxant responses to pinacidil, verapamil, theophylline and terbutaline in isolated airway smooth muscle. Pharmacol Toxicol 1992;70:46-9.
- Ratz PH, Berg KM, Urban NH, Miner AS. Regulation of smooth muscle calcium sensitivity: KCl as a calcium-sensitizing stimulus. Am J Physiol Cell Physiol 2005;288:C769-83.
- Wu XL, Wang YY, Cheng J, Zhao YY. Calcium channel blocking activity of calycosin, a major active component of Astragali Radix, on rat aorta. Acta Pharmacol Sin 2006;27:1007-12.
- Lefebvre B, Caron F, Bessard G, Stanke-Labesque F. Effect of 5-lipoxygenase blockade on blood pressure and acetylcholine-evoked endothelium-dependent contraction in aorta from spontaneously hypertensive rats. J Hypertens 2006;24:85-93.
- Sasaki N, Takano M, Mitsuiye T, Noma A. Changes in cell volume induced by ion channel flux in guinea-pig cardiac myocytes. Clin Exp Pharmacol Physiol 1999;26:698-706.
- Ferenczi EA, Fraser JA, Chawla S, Skepper JN, Schwiening CJ, Huang CL. Membrane potential stabilization in amphibian skeletal muscle fibres in hypertonic solutions. J Physiol 2004;555:423-38.
- de Clerck I, Guyssens B, Pannier JL, Van de Voorde J. Hyperosmo-larity causes BK Ca-dependent vasodilatations in rat skeletal muscle arteries. Med Sci Sports Exerc 2005;37:1697-703.
- Wijetunge S, Hughes AD. Mechanism of contraction of rat isolated tail arteries by hyposmotic solutions. J Vasc Res 2005;42:93-100.
- Collins EM, Walsh MP, Morgan KG. Contraction of single vascular smooth muscle cells by phenylephrine at constant [Ca2+]i. Am J Physiol 1992;262:H754-62.
- Barron LA, Green GM, Khalil RA. Gender differences in vascular smooth muscle reactivity to increases in extracellular sodium salt. Hypertension 2002;39:425-32.
- Zhang AM, Altura BT, Altura BM. Endothelial-dependent sexual dimorphism in vascular smooth muscle: role of Mg2+ and Na+. Br J Pharmacol 1992;105:305-10.
- Miao K, Wondergem R, Hossler FE, Joyner WL. Contributions of K+, Na+, and Cl- to the membrane potential of intact hamster vascular endothelial cells. J Cell Physiol 1993;156:550-9.
- Faure S, Javellaud J, Achard JM, Oudart N. Vasoconstrictive effect of angiotensin IV in isolated rat basilar artery independent of AT1 and AT2 receptors. J Vasc Res 2006;43:19-26.
- Oniki H, Fujii K, Kansui Y, Goto K, Iida M. Effects of angiotensin II receptor antagonist on impaired endothelium-dependent and endothelium-independent relaxations in type II diabetic rats. J Hypertens 2006;24:331-8.
- Dubrovska G, Verlohren S, Luft FC, Gollasch M. Mechanisms of ADRF release from rat aortic adventitial adipose tissue. Am J Physiol Heart Circ Physiol 2004;286:H1107-13.
- Gao YJ, Holloway AC, Zeng ZH, Lim GE, Petrik JJ, Foster WG, et al. Prenatal exposure to nicotine causes postnatal obesity and altered perivascular adipose tissue function. Obes Res 2005;13:687-92.
- Verlohren S, Dubrovska G, Tsang SY, Essin K, Luft FC, Huang Y, et al. Visceral periadventitial adipose tissue regulates arterial tone of mesenteric arteries. Hypertension 2004;44:271-6.
- Gao YJ, Zeng ZH, Teoh K, Sharma AM, Abouzahr L, Cybulsky I, et al. Perivascular adipose tissue modulates vascular function in the human internal thoracic artery. J Thorac Cardiovasc Surg 2005;130:1130-6.
- Furchgott RF, Zawadzki JV. The obligatory role of endothelial cells in the relaxation of arterial smooth muscle by acetylcholine. Nature 1980;288:373-6.
- Dora KA, Doyle MP, Duling BR. Elevation of intracellular calcium in smooth muscle causes endothelial cell generation of NO in arterioles. Proc Natl Acad Sci USA 1997;94:6529-34.
- Burnstock G. Vessel tone and remodeling. Nat Med 2006;12:16-7.
- Fernandez-Alfonso MS. Regulation of vascular tone: the fat connection. Hypertension 2004;44:255-6.
- Gollasch M, Dubrovska G. Paracrine role for periadventitial adipose tissue in the regulation of arterial tone. Trends Pharmacol Sci 2004;25:647-53.