Sphingosylphosphorylcholine stimulates human monocyte-derived dendritic cell chemotaxis1
Introduction
Dendritic cells (DC) play a key role in the regulation of immune response. DC uptake pathogens or cancer cells, process them and then present processed peptides to the surface-bound major histocompatibility complex molecule, which is recognized by T cells[1,2]. DC migration is an important factor for the regulation of proper immune response against invading antigens. In terms of immature DC (iDC) functioning, trafficking is important for the recognition of antigens and their efficient uptake. After antigen uptake and processing in the peripheral tissues, DC undergo a maturation process and migrate to secondary lymphoid organs via the activation of some chemotactic receptors[3,4]. It has been reported that some extracellular stimuli, including a series of chemokines, stimulate DC chemotaxis[3–5]. Although some chemokines have been reported to regulate DC chemotaxis[3–5], further factors including bioactive lipid mediators involved in DC chemotaxis should be considered.
Sphingosylphosphorylcholine (SPC) is a bioactive lipid mediator which is a component of membrane lipids. Previous studies have demonstrated that SPC induces human neutrophils to generate cellular superoxide and calcium mobilization[6,7]. Two cell surface G protein-coupled SPC receptors have been suggested, namely, ovarian cancer G protein-coupled receptor 1 (OGR1) and G protein-coupled receptor 4 (GPR4)[8,9]. Moreover, as members of the lysopho-spholipid family play an important role in innate immune response, SPC has proven useful in the study of phagocyte activation mechanisms. However, the effect of SPC on immune response by DC has not been elucidated. In the present study, we investigated the effects of SPC on DC trafficking in iDC and mDC, and further investigated the target receptors and signaling pathways involved in the regulation of SPC-induced DC chemotaxis.
Materials and methods
Reagents RPMI-1640 medium was bought from Invitrogen Corp (Carlsbad, CA, USA). Dialyzed fetal bovine serum was purchased from Hyclone Lab Inc (Logan, UT, USA). SPC and VPC23019 were purchased from Avanti Polar Lipids Inc (Alabaster, AL, USA). Lipopolysaccharide (LPS, derived from Escherichia coli strain 055:B5) was obtained from Sigma (St Louis, MO, USA). 2'-Amino-3'-methoxyflavone (PD98059), 4-(4-fluorophenyl)-2-(4-methylsulfinylphenyl)-5-(4-pyridyl)1H-imidazole (SB203580) and 1,2-bis (amino-phenoxy) ethane-N,N,N’,N’-tetraacetoxymethyl ester (BAPTA/AM) were from Calbiochem (San Diego, CA, USA). 2-(4-Morpholinyl)-8-phenyl-4H-1-benzopyran-4-one (LY294002) was from BIOMOL Research Laboratories. (Plymouth Meeting, PA, USA). Rabbit anti-human antibodies to extracellular signal regulated protein kinase (ERK), phospho-ERK (pERK), phospho-p38 (pp38), Akt, and phospho-Akt (pAkt) were purchased from Cell Signaling Technology, Inc (Beverly, MA, USA), and horseradish peroxidase-conjugated antibodies to rabbit IgG were purchased from Kirkegaard & Perry, Inc (Gaithersburg, MD, USA).
Generation of human DC Peripheral blood was collected from healthy donors; peripheral blood mononuclear cells were isolated by separation on a Histopaque-1077 gradient, as previously described[10]. After washing twice with Hanks’ buffered saline solution (without Ca2+ and Mg2+), the peripheral blood mononuclear cells were suspended in RPMI-1640 medium containing 10% FBS and incubated for 60 min at 37 oC to allow monocytes to attach to the culture dish. Attached monocytes were then collected as described previously[10]. Peripheral blood monocytes were differentiated to DC by culture in 6-well plates in 2 mL of complete medium (RPMI-1640 medium supplemented with 10% fetal bovine serum), supplemented with recombinant human granulocyte macrophage-colony stimulating factor (10 ng/mL; Pierce Endogen, Rockford, IL, USA) and recombinant human IL-4 (10 ng/mL; Pierce Endogen, Rockford, IL, USA)[11]. All cultures were incubated at 37 oC in 5% humidified CO2. After 7 d of culture, the DC were matured by stimulation with LPS (100 ng/mL) for 48 h[11]. The generation of iDC and mDC from peripheral blood monocytes was confirmed by FACS analysis using antibodies against several CD markers, as earlier described[11,12]. The addition of granulcotye-colony stimulating factor (10 ng/mL) and IL-4 (10 ng/mL) induced monocyte differentiation of human monocytes into DC. CD14 was down-regulated in the DC, but CD1a, CD40 and HLA-DR were significantly upregulated in the DC compared with the monocytes[12]. LPS (100 ng/mL) treatment dramatically enhanced CD86 and HLA-DR expression, as reported earlier[11].
Chemotaxis assay Chemotaxis assays were performed using multi well chambers (Neuroprobe Inc, Gaithersburg, MD, USA)[13]. Briefly, prepared DC were suspended in RPMI-1640 medium at 1×106 cells/mL, and 25 μL of this suspension was placed into the upper well of a chamber separated by an 8 μm polyhydrocarbon filter from the lipid-containing lower well. After incubation for 90 min at 37 oC, non-migrated cells were removed by scraping, and cells that had migrated across the filter were dehydrated, fixed and stained with hematoxylin (Sigma; St Louis, MO, USA). Stained cells from a particular well were then counted in 3 randomly chosen high power fields (×400)[14].
Stimulation of DC with SPC for Western blot analysis
DC (2×106) were stimulated with SPC at the indicated concentrations for predetermined times. After stimulation, the cells were washed with serum-free RPMI-1640 medium and lysed in lysis buffer (20 mmol/L Hepes, pH 7.2, 10% glycerol, 150 mmol/L NaCl, 1% Triton X-100, 50 mmol/L NaF, 1 mmol/L Na3VO4, 10 µg/mL leupeptin, 10 µg/mL aprotinin and 1 mmol/L phenylmethylsulfonyl fluoride). Detergent insoluble materials were pelleted by centrifugation (12 000×g, 15 min at 4 oC), and the soluble supernatant fraction was removed and stored at either -80 oC or used immediately. Protein concentrations in the lysates were determined using Bradford protein assay reagent.
Electrophoresis and immunoblot analysis Protein samples were prepared for electrophoresis and then separated using a 10% SDS-polyacrylamide gel and the buffer system described previously[15]. Following electrophoresis, they were blotted onto nitrocellulose membranes, which were then blocked by incubation in Tris-buffered saline containing Tween-20 (TBST) (25 mmol/L Tris-HCl, 150 mmol/L NaCl, and 0.05% TBST) and 5% dried non-fat milk. The membranes were then incubated with anti-phospho-ERK antibody, anti-phospho-Akt kinase antibody, anti-ERK antibody or anti-Akt antibody and washed with TBST. Antigen-antibody complexes were visualized using an enhanced chemiluminescence detection system by incubating membranes with 1:5000 diluted goat anti-rabbit IgG or goat anti-mouse IgG antibody, coupled with a horseradish peroxidase.
Reverse transcription polymerase chain reaction (RT-PCR) analysis mRNA was isolated by using a QIAshredder and an RNeasy kit (Qiagen, Hilden, Germany). mRNA, M-MLV reverse transcriptase, and pd (N) 6 primers (Imvitrogen corp; Carlsbad, CA, USA) were used to obtain cDNA. The primers used for the RT-PCR analysis were reported previously[16]. The sequences of the primers used were as follows. Human OGR1: forward, 5'-TTCCTGCCCTA-CCACGTGTTGC-3'; reverse, 5'-CTTCCAGACCCCTAACT-CGCCA-3'; human GPR4: forward, 5'-ACCTCTATCGGGTG-TTCGTG-3'; reverse, 5'-CCACTCACCTCCAAGAGGAA-3'; and human GAPDH: forward, 5'-GATGACATCAAGAAGG-TGGTGAA-3', reverse, 5'-GTCTTACTCCTTGGAGGCCA-TGT-3'. Amplification was performed over 30 cycles (94 oC/1 min [denaturation], 62 oC/1 min [annealing], and 72 oC/1 min [extension]). PCR products were electrophoresed in 2% agarose gel and visualized by ethidium bromide staining.
Statistics The results are expressed as Mean±SD of the number of determinations indicated. Statistical significance of differences was determined by ANOVA. Significance was accepted when P<0.05.
Results
SPC induces chemotaxis of iDC and mDC In the present study, we examined the effect of SPC on the migration of iDC. Various concentrations of SPC caused iDC migration showing a concentration dependency. SPC 100 nmol/L elicited maximal migration in iDC (Figure 1A). To investigate whether maturation of DC affects responsiveness to SPC, the DC were matured in the presence of LPS and chemotactic migration by SPC was examined. mDC also migrated to SPC (Figure 1B). In mDC, SPC-induced chemotactic migration was maximal at 1 μmol/L (Figure 1B). To distinguish between SPC-induced chemotaxis and chemokinesis, we performed migration assays in the absence or presence of SPC in the upper wells of the chambers as described previously[17]. As shown in Figure 1, the addition of SPC (10 μmol/L) in the upper chamber reduced the SPC-induced migrations of iDC and mDC to the lower well, which demonstrates that SPC induces DC chemotaxis.
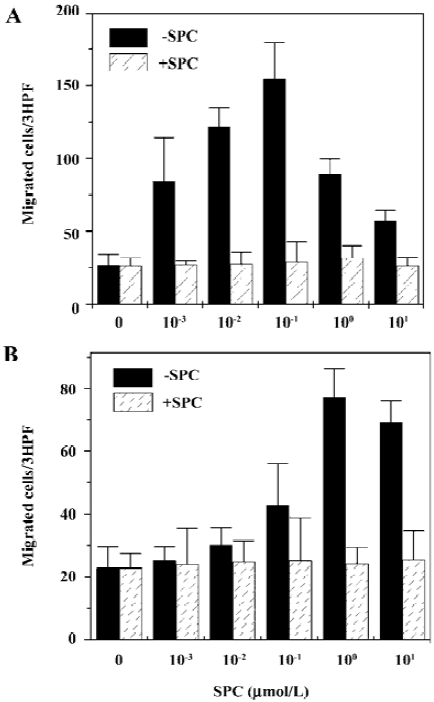
SPC induces DC chemotaxis via pertussis toxin-sensitive G proteins Many previous reports have demonstrated that pertussis toxin (PTX)-sensitive G protein-mediated signaling is critically involved in DC chemotaxis[18,19]. We also examined the effect of PTX on SPC-induced DC chemotaxis. When iDC were preincubated with 100 ng/mL of PTX prior to the chemotaxis assay, the number of cells migrating toward SPC was reduced by >95% versus cells not treated with PTX (Figure 2A). Preincubation of mDC with 100 ng/mL of PTX prior to the chemotaxis assay also almost completely inhibited SPC-induced chemotactic migration in mDC (Figure 2B). These results strongly suggest the involvement of PTX-sensitive G proteins in SPC-induced chemotaxis in iDC and mDC.
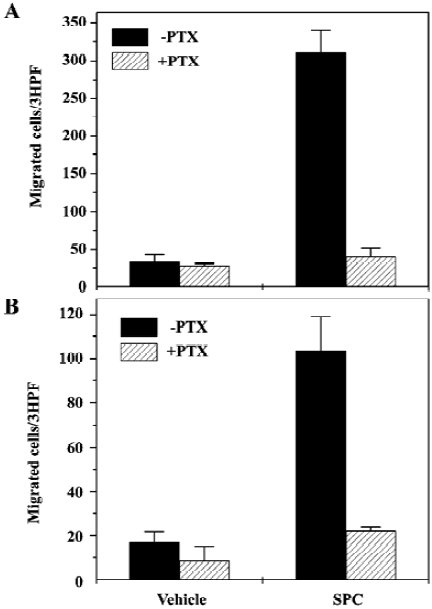
SPC stimulates mitogen-activated protein kinases (MAPK) and Akt activity in DC MAPK has been reported to mediate extracellular signals to the nucleus in a variety of cell types[20]. In this study, we attempted to determine whether SPC stimulated MAPK activity by Western blotting with anti-phospho-specific antibodies for the enzyme. When iDC were stimulated with SPC (1 μmol/L) for several lengths of time, ERK phosphorylation levels increased transiently and exhibited maximal activity after 5 min of stimulation (Figure 3A), which returned to baseline after 30 min of stimulation (Figure 3A). SPC also stimulated p38 kinase phosphorylation in a time-dependent manner, showing maximal activity at 2–5 min after stimulation (Figure 3A). The effect of SPC on Akt phosphorylation in iDC was also checked. SPC caused Akt phosphorylation at 2–30 min of stimulation with SPC in iDC (Figure 3B).
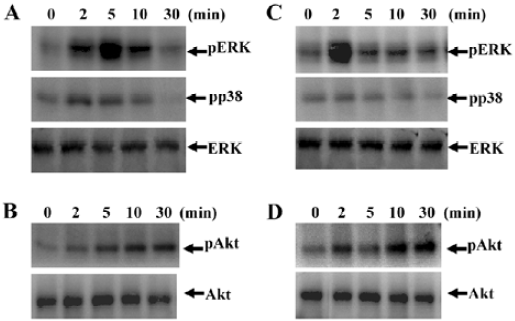
We then examined the effect of SPC on MAPK phosphorylation in mDC. Treating mDC with 1 μmol/L of SPC caused both ERK and p38 kinase phosphorylation at 2 min of stimulation, which returned to baseline after 30 min of stimulation (Figure 3C). SPC also caused Akt phosphorylation at 2–30 min of stimulation with SPC in mDC (Figure 3D).
Regulation of SPC-induced DC chemotaxis We observed that SPC dramatically induced DC chemotaxis (Figure 1). In order to elucidate the intracellular signaling pathways involved in the induction of DC chemotaxis by SPC, we performed chemotaxis assay using cells treated with an ERK pathway inhibitor (PD98059), which prevents the activation of MAPK kinase 1/2, an upstream activator of ERK 1/2. PD98059 treatment inhibited the SPC-induced iDC chemotaxis (Figure 4A). This finding suggests that the PD98059-inhibitable MAPK pathway may be involved in SPC-induced iDC chemotaxis. We also examined the effects of the p38 kinase, PI3K and Ca2+ pathways on the SPC-induced iDC chemotaxis. As shown in Figure 4A, pretreatment of DC with SB203580 (20 µmol/L) or LY294002 (50 µmol/L) for 15 min, before treatment with SPC, blocked the chemotactic migration by SPC. However, BAPTA/AM, a Ca2+ chelator did not affect the SPC-induced chemotactic migration in iDC (Figure 4A), ruling out the role of Ca2+ in the process. Taken together, these results suggest that the ERK, p38 kinase and PI3K pathways exert a positive effect on SPC-induced iDC migration.
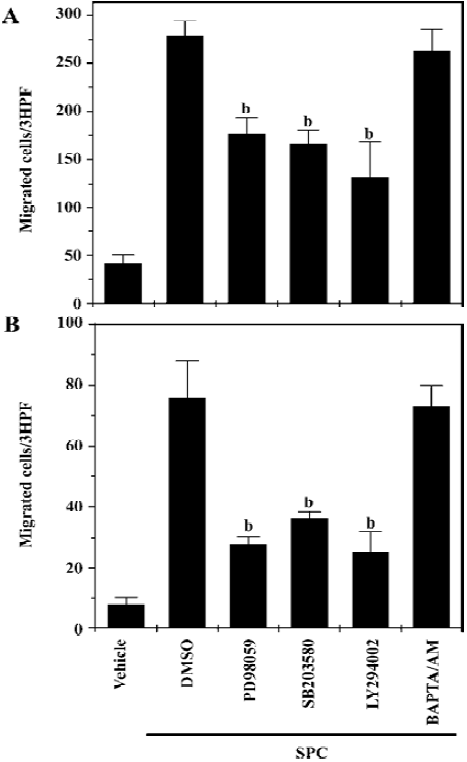
We also investigated the signaling pathways involved in SPC-induced chemotactic migration in mDC. SPC-induced mDC chemotaxis was also strongly inhibited by PD98059, SB203580 and LY294002, but not by BAPTA/AM (Figure 4B). The results also indicate that SPC induces mDC chemotaxis via ERK, p38 kinase and PI3K-mediated pathways.
DC express receptors for SPC Previous studies have demonstrated that SPC acted on at least 2 different cell surface receptors, ie, OGR1 and GPR4[8,9]. Since we observed that SPC induced chemotactic migration in iDC and mDC, we examined the expression pattern of SPC receptors in DC using RT-PCR analysis. As shown in Figure 5, human monocytes express OGR1, but not GPR4. iDC were not found to express both OGR1 and GPR4 (Figure 5). Like monocytes, mDC express OGR1, but not GPR4 (Figure 5). The results indicate that the expression patterns of SPC receptors change during the differentiation and maturation process of human monocyte-derived DC.
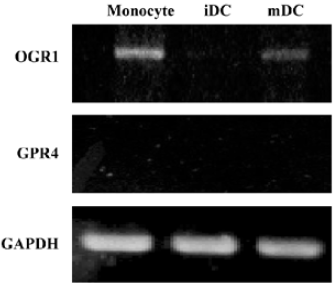
Role of VPC23019 on SPC-induced DC chemotaxis In order to determine whether SPC shows DC chemotaxis via S1P receptors, we utilized the S1P receptor-selective antagonist, VPC23019. As shown in Figure 6, S1P-induced DC chemotaxis was completely inhibited by preincubating DC with 10 µmol/L of VPC23019. However, SPC-induced DC chemotaxis was unaffected by VPC23019 (10 µmol/L; Figure 6). These results strongly indicate that SPC acts as a unique cell surface receptor, which is different from S1P receptors, resulting in DC chemotaxis.
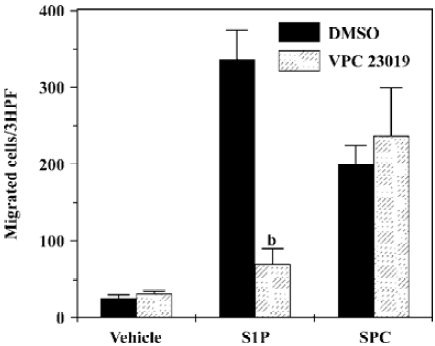
Discussion
In this study, we investigated the effects of SPC on DC chemotaxis. SPC caused chemotactic migration of iDC and mDC, suggesting that SPC act as a chemoattractant for DC throughout maturation (Figure 1). We also found the expression of SPC receptors in iDC and mDC, and the signaling pathways led to chemotactic migration.
Since DC are key players for the induction of immune responses, their migration is a very important factor for the regulation of immune responses against invading pathogens. Several previously published reports have demonstrated that some lipid mediators, such as lysophosphatidic acid and S1P, could induce DC migration into distinct anatomical regions[21,22]. In this study, we showed that another important lipid mediator, SPC, regulated DC chemotaxis in mDC as well as in iDC. Since S1P, a similar sphingolipid, also acts as a DC chemoattractant, we examined the utilization of S1P receptors by SPC. As there was no effect of the S1P receptor antagonist, VPC23019, on SPC-induced DC chemotaxis, we can rule out the involvement of S1P receptors on SPC-induced DC chemotaxis.
SPC is a component of membrane lipids and occurs naturally in blood plasma and in high density lipoprotein particles[23]. It has been reported that high amounts of SPC are found in the brain of patients with type A Niemann-Pick disease, resulting from a deficiency of sphingomyelinase activity[24]. SPC is a potent mitogen that increases intracellular free calcium and free arachidonate, resulting in the activation of AP-1, which may contribute to the pathophysiology of Niemann-Pick disease[24]. High levels of SPC have also been reported in the epidermis of atopic dermatitis patients who express abnormally high levels of sphingomyelin deacylase[25]. It has been suggested that SPC has a pathological effect in skin disease by modulating inflammatory processes of the epidermis via upregulation of intercellular adhesion molecule-1 and tumor necrosis factor-α[25,26]. The levels of SPC have been reported to be higher in malignant ascites of patient with ovarian cancer, suggesting that SPC may be involved in ovarian cancer development[27]. SPC has been reported to induce chemotactic migration of IL-2 activated natural killer cells[28] and vascular endothelial cells[29]. From these reports, SPC has been proposed to be involved in inflammation, angiogenesis and cancer. In this study, we demonstrated that SPC induces DC chemotaxis. Taken together, SPC may play important roles in the modulation of several pathological responses, including inflammation and cancer via inducing DC chemotaxis.
Because some reports have demonstrated that SPC binds to its specific G protein-coupled receptors, including OGR1 and GPR4[8,9], we also checked the expression pattern of SPC receptors in monocytes, iDC, and mDC. During the differentiation of human monocytes into iDC, OGR1 was found to be down-regulated, and iDC were found not to express OGR1 (Figure 5). The functional roles of OGR1 on DC chemotaxis had not been previously determined. Here, we confirmed that mDC, but not iDC, express OGR1 (Figure 5), suggesting a potential role of OGR1 in mDC chemotaxis. Taken together, we suggest that SPC induces iDC chemotaxis via a unique receptor which is different from OGR1 or GPR4. Since SPC-induced iDC chemotaxis were PTX-sensitive, unknown SPC receptors were coupled with the PTX-sensitive G protein. According to our result, even though OGR1 is not expressed in iDC, it is expressed in mDC (Figure 5). Furthermore, SPC induced chemotactic migration in mDC (Figure 1), although it is unclear whether this was done via OGR1.
With the downstream signaling of the SPC receptor in iDC and mDC, the results were perplexing. Although SPC stimulated chemotactic migration of DC in a PTX-sensitive manner, SPC did not induce calcium release. Until now, SPC receptors, OGR1 and GPR4 have been reported to stimulate calcium release and calcium release via PTX-sensitive G proteins. Keeping in mind previous reports and the results of the present study, it is reasonable to assume that SPC also acts on another receptor which is different from OGR1 and GPR4.
A variety of chemoattractants induce chemotaxis of leukocytes via their own receptors. Chemoattractant-induced signaling and chemotaxis in DC are PTX-sensitive, indicating the involvement of PTX-sensitive G protein-coupled receptors[19,30]. In the present study, SPC-induced DC chemotaxis was also found to be PTX-sensitive (Figure 2). Other important components of the chemoattractant-mediated signaling for DC chemotaxis are PI3K and MAPK[31,32]. SPC also activates multiple types of protein kinases, including ERK, p38 kinase, and PI3K activities downstream of the receptor activation. DC chemotaxis by SPC shows a LY294002, PD98059 and SB203580-sensitivity, indicating a PI3K, ERK, and p38 kinase-dependency (Figure 4). Several signaling molecules involved in the regulation of DC chemotaxis have been reported[32,33]. They include phospholipase C, Ca2+, PI3K, MAPK, Rho, and pyk2[33]. SPC-induced mDC chemotaxis was more largely inhibited by PD98059, SB203580 and LY294002 than that of iDC chemotaxis. It suggests that other signaling molecules (except PI3K, ERK and p38 kinase) will play a role in SPC-induced iDC chemotaxis.
In conclusion, our findings indicate that SPC modulates chemotactic migration in iDC and mDC. Our findings also suggest a new perspective on the roles of OGR1 or SPC receptors in the regulation of immune responses via their chemotactic activity for DC. Furthermore, these findings suggest that SPC receptors should be regarded as important chemotherapeutic targets with respect to the modulation of DC migration.
References
- Banchereau J, Steinman RM. Dendritic cells and the control of immunity. Nature 1998;392:245-52.
- Lanzavecchia A, Sallusto F. Dynamics of T lymphocyte responses: intermediates, effectors, and memory cells. Science 2000;290:92-7.
- Sozzani S, Allavena P, Vecchi A, Mantovani A. The role of chemokines in the regulation of dendritic cell trafficking. J Leukoc Biol 1999;66:1-9.
- Zlotnik A, Yoshie O. Chemokines: a new classification system and their role in immunity. Immunity 2000;12:121-7.
- Randolph GJ, Angeli V, Swartz MA. Dendritic-cell trafficking to lymph nodes through lymphatic vessels. Nat Rev Immunol 2005;5:617-28.
- Van Koppen CJ, Meyer Z, Heringdorf D, Zhang C, Laser KT, Jakobs KH. A distinct G(i) protein-coupled receptor for sphingosylphosphorylcholine in human leukemia HL-60 cells and human neutrophils. Mol Pharmacol 1996;49:956-61.
- Okajima F, Kondo Y. Pertussis toxin inhibits phospholipase C activation and Ca mobilization by sphingosylphosphorylcholine and galactosylsphingosine in HL60 leukemia cells. Implications of GTP-binding protein-coupled receptors for lysosphingolipids. J Biol Chem 1995; 270: 26 332–40.
- Xu Y, Zhu K, Hong G, Wu W, Baudhuin LM, Xiao Y, et al. Sphingosylphosphorylcholine is a ligand for ovarian cancer G-protein-coupled receptor 1. Nat Cell Biol 2000;2:261-7.
- Zhu K, Baudhuin LM, Hong G, Williams FS, Cristina KL, Kabarowski JH, . Sphingosylphosphorylcholine and lysophosphatidylcholine are ligands for the G protein-coupled receptor GPR4. J Biol Chem 2001; 276: 41 325–35.
- Bae YS, Bae H, Kim Y, Lee TG, Suh PG, Ryu SH. Identification of novel chemoattractant peptides for human leukocytes. Blood 2001;97:2854-62.
- Kang HK, Lee HY, Kim MK, Park KS, Park YM, Kwak JY, et al. The synthetic peptide Trp-Lys-Tyr-Met-Val-D-Met inhibits human monocyte-derived dendritic cell maturation via formyl peptide receptor and formyl peptide receptor-like 2. J Immunol 2005;175:685-92.
- Kang HK, Lee HY, Lee YN, Jo EJ, Kim JI, Kim GY, et al. Up-regulation of phospholipase Cgamma1 and phospholipase D during the differentiation of human monocytes to dendritic cells. Int Immunopharmacol 2004;4:911-20.
- Bae YS, Yi HJ, Lee HY, Jo EJ, Kim JI, Lee TG, et al. Differential activation of formyl peptide receptor-like 1 by peptide ligands. J Immunol 2003;171:6807-13.
- Bae YS, Lee HY, Jo EJ, Kim JI, Kang HK, Ye RD, et al. Identification of peptides that antagonize formyl peptide receptor-like 1-mediated signaling. J Immunol 2004;173:607-14.
- Bae YS, Song JY, Kim Y, He R, Ye RD, Kwak JY, et al. Differential activation of formyl peptide receptor signaling by peptide ligands. Mol Pharmacol 2003;64:841-7.
- Yun MR, Okajima F, Im DS. The action mode of lysophosphatidyl choline in human monocytes. J Pharmacol Sci 2004;94:45-50.
- Bae YS, Kim Y, Kim Y, Kim JH, Suh PG, Ryu SH. Trp-Lys-Tyr-Met-Val-D-Met is a chemoattractant for human phagocytic cells. J Leukoc Biol 1999;66:915-22.
- Yang D, Chertov O, Bykovskaia SN, Chen Q, Buffo MJ, Shogan J, et al. Beta-defensins: linking innate and adaptive immunity through dendritic and T cell CCR6. Science 1999;286:525-8.
- Idzko M, Dichmann S, Ferrari D, Di Virgilio F, la Sala A, Girolomoni G, et al. Nucleotides induce chemotaxis and actin polymerization in immature but not mature human dendritic cells via activation of pertussis toxin-sensitive P2y receptors. Blood 2002;100:925-32.
- Johnson GL, Lapadat R. Mitogen-activated protein kinase pathways mediated by ERK, JNK, and p38 protein kinases. Science 2002;298:1911-2.
- Idzko M, Panther E, Corinti S, Morelli A, Ferrari D, Herouy Y, et al. Sphingosine 1-phosphate induces chemotaxis of immature and modulates cytokine-release in mature human dendritic cells for emergence of Th2 immune responses. FASEB J 2002;16:625-7.
- Panther E, Idzko M, Corinti S, Ferrari D, Herouy Y, Mockenhaupt M, et al. The influence of lysophosphatidic acid on the functions of human dendritic cells. J Immunol 2002;169:4129-35.
- Nofer JR, Fobker M, Hobbel G, Voss R, Wolinska I, Tepel M, et al. Activation of phosphatidylinositol-specific phospholipase C by HDL-associated lysosphingolipid. Involvement in mitogenesis but not in cholesterol efflux. Biochemistry 2000;39:15199-207.
- Berger A, Rosenthal D, Spiegel S. Sphingosylphosphocholine, a signaling molecule which accumulates in Niemann-Pick disease type A, stimulates DNA-binding activity of the transcription activator protein AP-1. Proc Natl Acad Sci USA 1995;92:5885-9.
- Murata Y, Ogata J, Higaki Y, Kawashima M, Yada Y, Higuchi K, et al. Abnormal expression of sphingomyelin acylase in atopic dermatitis: an etiologic factor for ceramide deficiency? J Invest Dermatol 1996;106:1242-9.
- Imokawa G, Takagi Y, Higuchi K, Kondo H, Yada Y. Sphingosylph osphorylcholine is a potent inducer of intercellular adhesion molecule-1 expression in human keratinocytes. J Invest Dermatol 1999;112:91-6.
- Xiao YJ, Schwartz B, Washington M, Kennedy A, Webster K, Belinson J, et al. Electrospray ionization mass spectrometry analysis of lysophospholipids in human ascitic fluids: comparison of the lysophospholipid contents in malignant vs nonmalignant ascitic fluids. Anal Biochem 2001;290:302-13.
- Jin Y, Damaj BB, Maghazachi AA. Human resting CD16–, CD16+ and IL-2-, IL-12-, IL-15- or IFN-alpha-activated natural killer cells differentially respond to sphingosylphosphorylcholine, lysophosphatidylcholine and platelet-activating factor. Eur J Immunol 2005;35:2699-708.
- Kim KS, Ren J, Jiang Y, Ebrahem Q, Tipps R, Cristina K, et al. GPR4 plays a critical role in endothelial cell function and mediates the effects of sphingosylphosphorylcholine. FASEB J 2005;19:819-21.
- Yang D, Rosenberg HF, Chen Q, Dyer KD, Kurosaka K, Oppenheim JJ. Eosinophil-derived neurotoxin (EDN), an antimicrobial protein with chemotactic activities for dendritic cells. Blood 2003;102:3396-403.
- Ardeshna KM, Pizzey AR, Walker SJ, Devereux S, Khwaja A. The upregulation of CC chemokine receptor 7 and the increased migration of maturing dendritic cells to macrophage inflammatory protein 3beta and secondary lymphoid chemokine is mediated by the p38 stress-activated protein kinase pathway. Br J Haematol 2002;119:826-9.
- Riol-Blanco L, Sanchez-Sanchez N, Torres A, Tejedor A, Narumiya S, Corbi AL, et al. The chemokine receptor CCR7 activates in dendritic cells two signaling modules that independently regulate chemotaxis and migratory speed. J Immunol 2005;174:4070-80.
- Scandella E, Men Y, Legler DF, Gillessen S, Prikler L, Ludewig B, et al. CCL19/CCL21-triggered signal transduction and migration of dendritic cells requires prostaglandin E2. Blood 2004;103:1595-601.