Translocation of classical PKC and cortical granule exocytosis of human oocyte in germinal vesicle and metaphase II stage1
Introduction
PKC is initially described as a family of serine/threonine kinases that can be activated by Ca2+, phospholipid and diacylglycerol (DAG)[1]. Until now, at least 12 isoforms have been identified and grouped in three subclasses. They are c protein kinase C (cPKC) including α, β1, βII, γ; novel PKCs (nPKC) including δ, ε, η, and θ; and atypical PKCs (aPKC) including ζ, λ, and µ. Most PKC research is focused on cPKC.
PKC is a major intracellular kinase that has been implicated in a large number of signal transduction processes that mediate extracellular signals into intracellular events. In the process of mammalian oocyte fertilization, PKC plays a role in three major events: (1) a periodic increase in intracellular Ca2+ (Ca2+ oscillations); (2) the release of CG, which blocks polyspermy; and (3) release from meiotic arrest.
Sousa et al[2] reported that fertilization-induced Ca2+ oscillations showed a periphery-to-centre propagation of Ca2+ waves and, at the same time, PKC were accumulated in the cell periphery in human preimplantation embryos. However, the isoforms of PKC were not addressed in the Sousa et al report. Until now, there has been a lack of information regarding which members of the PKC family are present in human oocytes. To better understand the role of PKC, it is necessary to clarify this issue prior to tracking PKC activity in maturation, fertilization and subsequent embryonic development[3].
The spare oocytes were donated and collected for research over the past 2 years. Due to limited specimens and ethical reasons, the major objective of this study is to establish the relationship between cPKC and CG exocytosis. We performed experiments to determine the translocation of cPKCs in human oocytes and tested whether cPKCs were involved in the signal process of CG exocytosis.
Materials and methods
Collection of oocytes Oocytes used in this study were collected from the Reproduction and Genetic Center of Peking University First Hospital. These specimens were clinically unsuitable or redundant after manipulations of intracytoplasmic sperm injection (ICSI). Written consent was obtained from both sides of the couples. This study was approved by the Ethics Review Committee of Peking University.
Immature oocytes at GV or metaphase I (MI) stage were retrieved using transvaginal retrieval method 36 h after control oocyte hyperstimulation under the guidance of ultrasonography. The oocytes of GV-stage were stained with immunofluorescence. The oocytes of MI-stage were cultured in Hepes medium (Invitrocare, San Diego, CA, USA) containing 10% serum substitute supplement (Irvine Scientific, Santa Ana, CA, USA) at 37 °C, 5% CO2 to form MII oocytes. The MII oocytes were used for immunofluorescence or treated with drugs.
Drug treatment of oocytes To study the relationship between exocytosis of CG and activation of classical PKC α, β1 and γ, phorbol 12-myristate 13-acetate (PMA, Alexis, San Diego, CA, USA), staurosporine (Alexis) and 4α-phorbol 12,13-didecanoate (4α-PDD; Alexis) were used to treat oocytes. The chemicals were dissolved in dimethylsulfoxide (Sigma, St Louis, MO, USA), stored at –20 °C, and diluted with Hepes buffer medium prior to use.
It has been reported that 1.62 µmol PMA could induce the translocation of cPKC isoforms and exocytosis of CG[4]. However, in this experiment, we had to use 3.2 µmol PMA to see translocation. For evaluation of translocation of cPKC and exocytosis of CG, MII oocytes were treated with 3.2 µmol PMA for 15 min followed by LCSM. As control, oocytes were treated with 3.2 µmol 4α-PDD, a biologically inactive phorbol ester. To evaluate exocytosis of CG and distribution of PKC α, β1 and γ, MII oocytes were treated sequentially with 3.2 µmol PMA for 15 min, 2 µmol staurosporine for 15 min followed by 3.2 µmol PMA plus 2 µmol staurosporine for 15 min.
Immunofluorescence Oocytes were briefly treated with acidic Tyrode's solution (pH 2.1−2.5; Sigma, St Louis, MO, USA) to remove zona pellucida [4]. Zona-free cells were fixed for 15 min in 4% paraformaldehyde (2% paraformaldehyde for MII oocytes). Double staining of PKC isoforms and CG was performed as described in a previous study[5]. Briefly, oocytes were permeabilized with incubation buffer (0.5% Triton-100 in 20 mmol/L Hepes, pH 7.4, 3 mmol/L MgCl2, 50 mmol/L NaCl, 300 mmol/L sucrose and 0.02% NaN3) for 30 min at 37 °C in a incubation chamber, followed by blocking in 1% BSA for 1 h and incubation overnight with Lens Culinaris Agglutinin-biotin (LCA-biotin; Vector Labs, Burlingame, CA, USA) for CG staining or the specific antibodies (mouse anti-human) for PKC α, β1 and γ (1:80; Αlexis) at 4 °C. After extensive washes, oocytes were incubated with Fluorescein isothiocynate (FITC)-labeled streptavidin for CG stain or rhodamine-labeled rabbit-anti-mouse IgG antibody (KPL, Gaithersburg, MD, USA) for PKCα, β1 or γ (Southern Biotech, Birmingham, AL, USA) for 1 h. Finally, cells were stained with 0.02 g/L Hoechst 33258 (Molecular Probes, Eugene, OR, USA) 10 min for displaying chromatin, mounted on slides using GVA-mount (Zymed, San Francisco, CA, USA) and examined by using LCSM (MRC-2100, Bio-Rad, Richmond, USA). Nonspecific staining was determined by incubation with isotype control antibodies.
Data analysis The SPSS version 11.0 for Windows (SPSS Inc., Chicago, IL, USA) software was used for the statistical analysis including the Student-Neumann-Keul's test and the paired t-test. The values were presented as the mean±SEM. P<0.05 was considered to be statistically significant. Oocytes displaying signs of degeneration as indicated by dark cytoplasm or fragmented cytoplasm were excluded from analysis.
Results
Changes of cPKCs in the maturation processes of oocytes from GV to MII stage In this study, human oocytes at GV and MI stage retrieved from patients were the redundant cells after artificial fertilization manipulations, and those at MII stage were obtained by in vitro culture of the MI oocytes. Immunofluorescence using antibodies against PKC α, β1 and γ showed that in GV oocytes, PKC α, β1 and γ were localized to the germinal vesicles, with a weak expression in the ooplasm (PKCα=100%, n=9; PKCβ1=88.89%, n=9; PKCγ=83.33%, n=12). In MII oocytes, PKC α, β1 and γ were distributed evenly in ooplasm (PKCα=88.89%, n=9; PKCβ1=100%, n=10; PKCγ=90%, n=10), and the expression of PKCα in the membrane was stronger than in the ooplasm. However, the expression of PKC γ in MII oocytes was weaker than that in GV (P<0.05). No staining was seen in the isotype control (Figure 1).
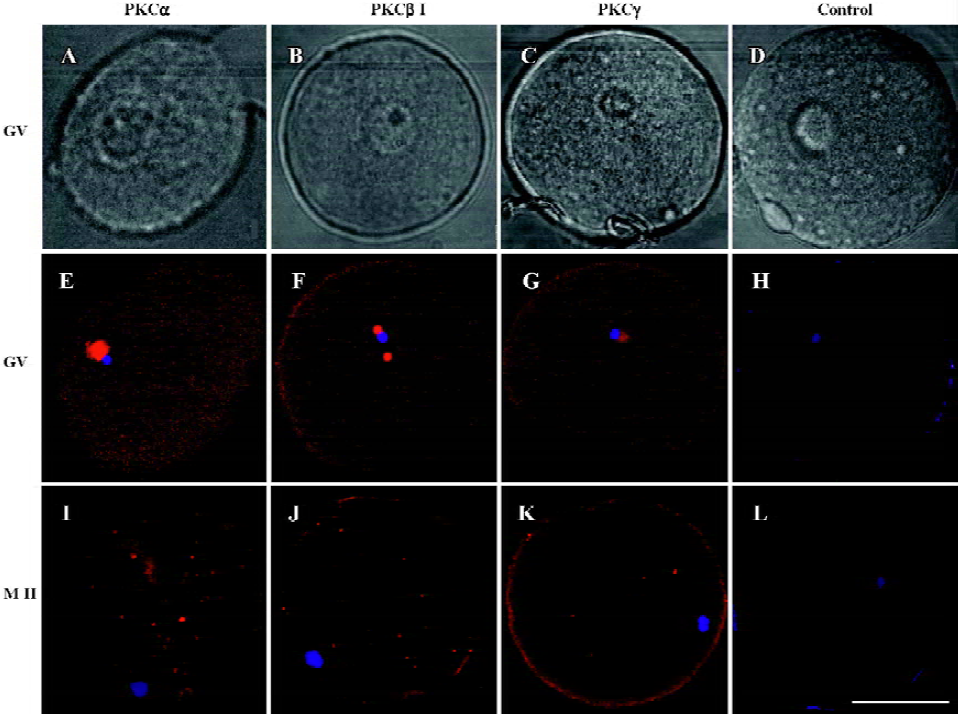
Changes of classical PKCs in oocytes treated with PKC activator, PKC inhibitor or 4α-PDD After exposure of MII oocytes to PKC activator PMA for 15 min, cPKC were transferred to the peripheral cytoplasm and cell membrane, with a marginal expression in ooplasm (PKCα=75%, n=8; PKCβ1=87.5%, n=8; PKCγ=60%, n=10) (Figure 2A–2C). Incubation with the PKC inhibitor staurosporine followed by PMA plus staurosporine blocked translocation of cPKC induced by PMA. The three PKCs (ie, α, β1 and γ) were evenly distributed in ooplasm under this condition (PKCα=100%, n=8; PKCβ1=100%, n=9; PKCγ=85.71%, n=7) (Figure 2D–2F). The biologically inactive phorbol ester, 4α-PDD could not induce translocation of PKC (PKCα=90%, n=10; PKCβ1=100%, n=10; PKCγ=85.71%, n=7) (Figure 2G–I). The difference was significant (P<0.05).
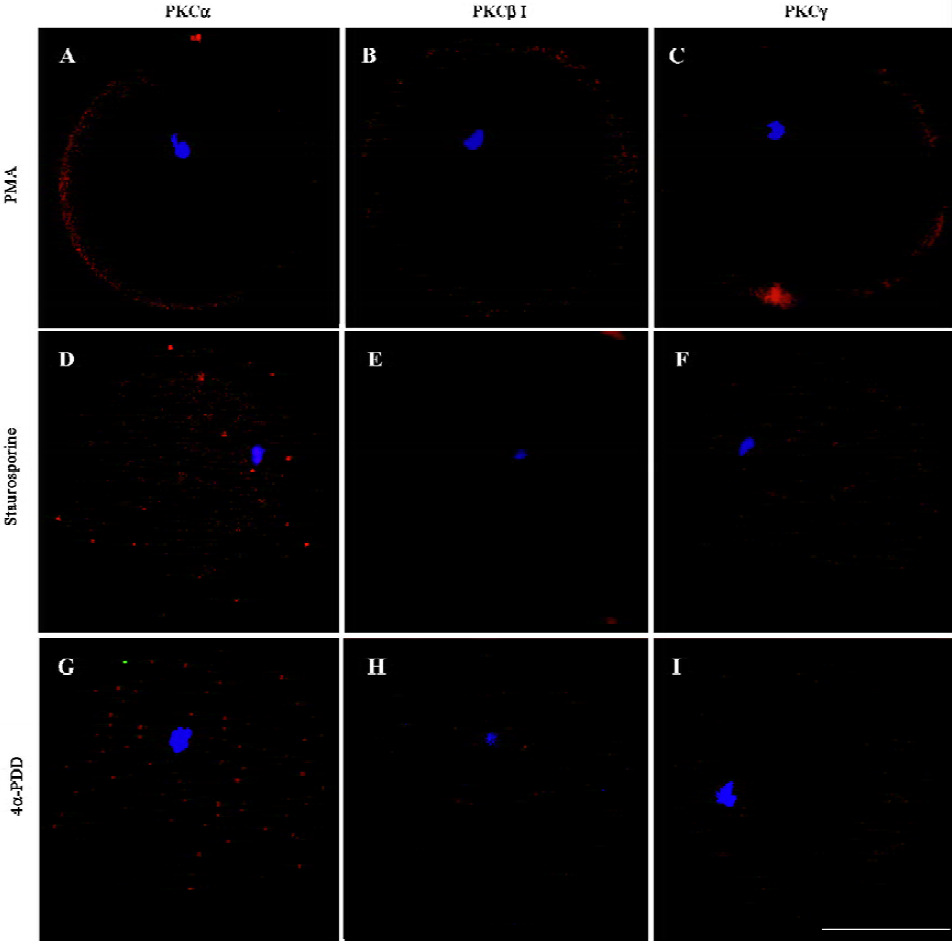
Exocytosis of CG in oocytes Exocytosis of CG was not found in mature oocytes (0%, n=29) (Figure 3A). Treatment with PKC activator PMA resulted in considerable exocytosis of CG in MII oocytes (80.77%, n=26) (Figure 3B). No significant exocytosis of CG was induced by treatment with PKC inhibitor and 4α-PDD (3.92%, n=51) (Figure 2D−I). The difference was significant (P<0.05).
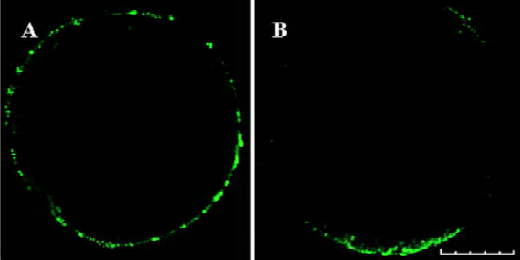
Discussion
The expression of PKC α, β1 and γ has been reported in animals but not in humans[6-9]. In our study, cPKC isoforms were found to be concentrated in germinal vesicles at the GV stage and dispersed in MII oocytes. In mouse GV oocytes, PKCα and PKCβII uniformly distribute in the cytoplasm, while PKCβI localizes in the cytoplasm and plasma membrane. In mouse MII eggs, although distribution of PKCα and PKCβI is the same as GV stage, PKCβII can distribute in the cytoplasm and plasma membrane[8]. In pig eggs, the three PKC isoforms (PKC α, β1 and γ) concentrate in the GV but evenly distribute in the cytoplasm of MII eggs[4]. The different location of cPKC in different species might correlate with their function. The functions of PKC in GV are unknown at present, but their compartmentalization during oocyte development may be physiologically important. Germinal vesicle breakdown (GVBD) was one of the most important steps during the maturation of oocytes. PKC elicits GVBD by activating maturation-promoting factor[10]. When activated by FSH and PKC, mitogen-activated protein kinases (MAPK) may stimulate the synthesis of specific proteins in cumulus cells followed by the secretion of an unknown positive factor that is capable of inducing GVBD in oocytes[11]. Direct activation of PKC in oocyte suppresses maturation, while stimulation in cumulus cells generates a positive trigger that leads to meiotic resumption[12-14]. PKC activity could disrupt the MI-to-MII transition, leading to a precocious exit from meiosis too[15].
There was further evidence suggesting that an increase in PKC activity occurred at fertilization as the activation of PKC was often associated with a translocation to the plasma membrane from cytoplasm. This is similar to our observations. Treatment with PMA, a PKC activator, PKC α, β1 and γ moved to the periphery and cell membrane, and CG exocytosis was induced at the same time. These effects were blocked in the presence of staurosporine, a potent inhibitor of PKC, suggesting that the activation of PKC is necessary for PKC translocation and CG exocytosis. As a control, 4α-PDD, a biologically inactive phorbol ester, could not induce translocation and CG exocytosis. Such a transition has also been observed in hamster oocytes after treatment with Ca2+[16,17] and spermatozoa[18].
Following the fusion of sperm and oocyte, the contents of CG release into the perivitelline space (cortical reaction), causing the zona pellucida to become refractory to sperm binding and penetration (zona reaction). The sperm-oocyte fusion, mediated by GTP-binding protein (G-protein), might elicit the generation of two second messengers, inositol 1,4,5 triphosphate (IP3)[19] and DAG. The former induce Ca2+ exocytosis from intracellular stores and the latter activate PKC, leading to CG exocytosis. The CG exocytosis cause zona sperm receptor modification and zona hardening, and thus blocks polyspermic penetration. Oolemma modification after sperm-oocyte fusion and formation of CG envelope following cortical reaction may also contribute to polyspermy block[20−22].
Pharmacological agents including phorbol esters and synthetic diacylglycerols have been used to imitate the events of egg activation, such as CG exocytosis and the second polar body initiation[23−25]. However, contradictory results have been obtained from the pharmacological studies using PKC activators or inhibitors on mammalian eggs. It has been found that phorbol esters induce the initiation of second polar body-like structures in hamster eggs; while in mouse eggs such structures have not been detected[26]. In mouse denuded oocytes, meiotic resumption was inhibited by phorbol esters[13].
Because a parthenogenetic agent (phorbol esters) does not fully mimic the egg activation, PKC activation following the sperm-induced egg activation should be assessed before drawing conclusions. Ducibella and LeFevre[27] addressed this issue and demonstrated the failure of PKC inhibitors to block fertilization-induced CG exocytosis. However, their results did not exclude the possibility of PKC involvement in exocytosis as either a very small amount of PKC was sufficient for cortical reaction or that other active pathways could compensate for PKC inhibition.
A key phenomena associated with PKC function in vivo is likely to be subcellular distribution of both the enzyme and substrate[28]. PKC isoforms have been found to be distributed differentially among different cell types together with a number of targeting proteins[29]. An aim in future study would be to identify the specific roles of each of these isoforms in human oocyte fertilization.
In conclusion, we revealed for the first time that PKC α, β1 and γ existed in human oocytes. Their subcellular distribution is differently regulated during oocyte maturation. cPKC isoforms participate in CG exocytosis in human oocytes and the activation of these subunits can induce CG exocytosis. However, the function of each of the isoforms needs to be investigated.
Acknowledgements
Thanks to Prof Lin LIU from Marine University and Prof Qing-yuan SUN from the Institute of Zoology of the Chinese Academy of Sciences who helped with the immunofluorescence.
References
- Nishizuka Y. Intracellular signaling by hydrolysis of phospholipids and activation of protein kinase C. Science 1992;258:607-14.
- Sousa M, Barros A, Tesarik J. Developmental changes in calcium dynamics, protein kinase C distribution and endoplasmic reticulum organization in human preimplantation embryos. Mol Hum Reprod 1996;2:967-77.
- Jones KT. Protein kinase C action at fertilization: overstated or undervalued? Rev Reprod 1998;3:7-12.
- Fan HY, Tong C, Li MY, Lian L, Chen DY, Schatten H, et al. Translocation of the classic protein kinase C isoforms in porcine oocytes: implications of protein kinase C involvement in the regulation of nuclear activity and cortical granule exocytosis. Exp Cell Res 2002;277:183-91.
- Luria A, Tennenbaum T, Sun QY, Rubinstein S, Breitbart H. Differential localization of conventional protein kinase C isoforms during mouse oocyte development. Biol Reprod 2002;62:1564-70.
- Eckberg WR, Szuts EZ. Diacylglycerol content of Chaetopterus oocytes during maturation and fertilization. Dev Biol 1993;159:732-35.
- Lu Z, Xia G, Zhang J. Protein kinase C, rather than protein kinase A is involved in follicle-stimulating hormone-mediated meiotic resumption of mouse cumulus cell-enclosed oocytes in hypoxanthine-supplemented medium. Mol Cell Endocrinol 2001;182:225-32.
- Luria A, Tennenbaum T, Sun QY, Rubinstein S, Breitbart H. Differential localization of conventional protein kinase C isoforms during mouse oocyte development. Biol Reprod 2000;6:1564-70.
- Avazeri N, Courtot AM, Lefevre B. Regulation of spontaneous meiosis resumption in mouse oocytes by various conventional PKC isozymes depends on cellular compartmentalization. J Cell Sci 2004;117:4969-78.
- Eckberg WR, Palazzo RE. Regulation of M-phase progression in Chaetopterus oocytes by protein kinase C. Dev Biol 1992;149:395-405.
- Fan HY, Huo LJ, Chen DY, Schatten H, Sun QY. Protein kinase C and mitogen-activated protein kinase cascade in mouse cumulus cells: cross talk and effect on meiotic resumption of oocyte. Biol Reprod 2004;70:1178-87.
- Downs SM, Cottom J, Hunzicker-Dunn M. Protein kinase C and meiotic regulation in isolated mouse oocytes. Mol Reprod Dev 2001;58:101-15.
- Lefevre B, Pesty A, Koziak K, Testart J. Protein kinase C modulators influence meiosis kinetics but not fertilizability of mouse oocytes. J Exp Zool 1992;264:206-13.
- Lu Q, Smith GD, Chen DY, Yang Z, Han ZM, Schatten H, et al. Phosphorylation of mitogen-activated protein kinase is regulated by protein kinase C, cyclic 3',5'-adenosine monophosphate, and protein phosphatase modulators during meiosis resumption in rat oocytes. Biol Reprod 2001;64:1444-50.
- Viveiros MM, Hirao Y, Eppig JJ. Evidence that protein kinase C (PKC) participates in the meiosis I to meiosis II transition in mouse oocytes. Dev Biol 2001;235:330-42.
- Gallicano GI, McGaughey RW, Capco DG. Protein kinase M, the cytosolic counterpart of protein kinase C, remodels the internal cytoskeleton of the mammalian egg during activation. Dev Biol 1995;167:482-501.
- Gallicano GI, Yousef MC, Capco DG. PKC-a pivotal regulator of early development. Bioessays 1997;19:29-36.
- Gallicano GI, McGaughey RW, Capco DG. Activation of protein kinase C after fertilization is required for remodeling the mouse egg into the zygote. Mol Reprod Dev 1997;46:587-601.
- Xu Z, Kopf GS, Schultz RM. Involvement of inositol 1,4,5-trisphosphate-mediated Ca2+ release in early and late events of mouse egg activation. Development 1994;7:1851-9.
- Sun QY. Cellular and molecular mechanisms leading to cortical reaction and polyspermy block in mammalian eggs. Microsc Res Tech 2003;61:342-8.
- Sun QY, Wang WH, Hosoe M, Taniguchi T, Chen DY, Shioya Y. Activation of protein kinase C induces cortical granule exocytosis in a Ca2+-independent manner, but not the resumption of cell cycle in porcine eggs. Dev Growth Differ 1997;39:523-9.
- Markoulaki S, Matson S, Abbott AL, Ducibella T. Oscillatory CaMKII activity in mouse egg activation. Dev Biol 2003;2:464-74.
- Bement WM. Signal transduction by calcium and protein kinase C during egg activation. J Exp Zool 1992;4:382-97.
- Bement WM, Capco DG. Protein kinase C acts downstream of calcium at entry into the first mitotic interphase of Xenopus laevis. Cell Regul 1990;3:315-26.
- Ducibella T, Kurasawa S, Duffy P, Kopf GS, Schultz RM. Regulation of the polyspermy block in the mouse egg: maturation-dependent differences in cortical granule exocytosis and zona pellucida modifications induced by inositol 1,4,5-trisphosphate and an activator of protein kinase C. Biol Reprod 1993;48:1251-7.
- Moore GD, Kopf GS, Schultz RM. Complete mouse egg activation in the absence of sperm by stimulation of an exogenous G protein-coupled receptor. Dev Biol 1993;2:669-78.
- Ducibella T, LeFevre L. Study of protein kinase C antagonists on cortical granule exocytosis and cell-cycle resumption in fertilized mouse eggs. Mol Reprod Dev 1997;46:216-26.
- Mochly-Rosen D, Gordon AS. Anchoring proteins for proteins kinase C: a means for isozyme selectivity. FASEB J 1998;12:35-42.
- Dekker LV, Parker PJ. Protein kinase C-a question of specificity. Trends Biochem Sci 1994;19:73-7.