Inhibitory effects of cariporide on LPC-induced expression of ICAM-1 and adhesion of monocytes to smooth muscle cells in vitro1
Introduction
It has been demonstrated that the Na+/H+ exchanger (NHE) is a ubiquitous protein present in mammalian cells and regulates cell volume and intracellular pH by extruding protons in exchange for sodium influx in an electroneutral 1:1 stoichiometric relationship. At least 8 NHE isoforms thus far have been identified and designated as NHE-1−8. The subtype 1 of NHE (NHE-1) is the only significant plasma membrane isoform present in the cardiovascular system to mediate myocardial ischemia and reperfusion injury as well as injury produced by cytokine including LPC[1,2]. Numerous inhibitors of NHE-1 have been developed to attempt to treat ischemia and reperfusion injury induced by myocardial infarct. In animal models, excellent success has been obtained in this regard[3,4]. Our previous studies have demonstrated that cariporide, a new selective NHE-1 inhibitor, suppressed serum-stimulated proliferation of SMC in vitro[5] and protected against dyfunction of vascular endothelium caused by hyperlipidemia and high glucose solution, as well as alleviated formation of atherosclerosis plaques induced by high cholesterol food in rabbits[6,7]. However, the mechanisms of cariporide against atherosclerosis were undefined.
Atherosclerosis is a multifactorial disease characterized by endothelial dysfunction, smooth muscle cell proliferation and migration, inflammation, lipid and matrix accumulation and thrombus formation. Migration and proliferation of SMC from the media into the intima and the adhesion of circula-ting monocytes to endothelial cells and SMC are thought to be early events in the development of atherosclerosis[8]. It was known that the circulating oxidative low density lipoprotein (ox-LDL) plays a critical role in hyperlipidemia inducing endothelial dysfunction. The ox-LDL-mediated leukocyte adhesion to endothelial cells or SMC and this adhesion are secondary to the expression of adhesion molecules on the luminal surfaces of endothelial cells in blood vessels. The ox-LDL signal transduction pathways to promote development and progression of atherosclerosis involve [Ca2+]i and the activation of NHE-1. LPC is a primary constituent of ox-LDL and many of the effects of ox-LDL can be mimicked by LPC. LPC also has the ability to promote monocytes adhering to the endothelial surface and SMC[9–11]. We presume that the effects of cariporide against the deleterious effects of high lipidemia on endothelium-mediated vasodilatation may be related to inhibiting lipids and ox-LDL stimulated leukocyte-endothelial cell and leukocyte-SMC interaction.
The aim of this study is to explore the mechanisms of cariporide against atherosclerosis by examining whether cariporide can inhibit monocytes adhering to SMC and the expression of ICAM-1 on the surface of SMC stimulated by LPC, and whether the inhibitory effects of cariporide on the adhesion of monocytes and expression of ICAM-1 is related to the change of intracellular pH stimulated by LPC.
Materials and methods
Drugs and reagents Cariporide was obtained by Hoechst Company (Frankfurt, Germany). Monoclonal antibodies of ICAM-1, trypsin, Ficoll-Hypaque, 2,7-bis-(2-carboxy-ethyl)-5,6-carboxyfluorescein tri-acetoxy-methylester (BCECF-AM), LPC and nigericin were purchased from Sigma Chemical Co (Saint Louis, Mo, USA). Horseradish peroxidase-conjugated anti-mouse IgG and bovine serum albumin (BSA, fraction V, very low endotoxin) were purchased from Boster Bioengineering Co (Wuhan China). Dulbecco’s modified Eagle’s medium (DMEM) was purchased from GIBCO (USA). Fetal bovine serum (FBS), phosphate buffer saline (PBS), penicillin and streptomycin were purchased from Si Ji Qing Co (Hangzhou, China).
Cell culture The bovine aortic smooth muscle cells (BASMC) were cultured according to procedures reported in previously published literature[5]. BASMC were obtained from a media layer of thoracic aorta in freshly killed cattle (1–7 d old) and cultured in DMEM medium supplemented with 10% (v/v) FBS and supplemented with 100 U/mL penicillin and 100 µg/mL streptomycin at 37 °C in a humidified atmosphere with 5% CO2. SMC were identified by their typical ‘hill and valley’ growth pattern and by positive fluorescence with antibodies against smooth muscle actin. Cells from passages 4 to 10 were used in the experiments.
Isolation of monocytes The monocytes were isolated by a modified method as described by Recalde[12]. In brief, human leukocyte-rich buffy coats of healthy blood donors obtained from the blood supply center of the Xiang-ya Hospital (Changsha, China) were mixed with dextran and remnant red blood cells were allowed to sediment. The monocytes were separated from lymphocytes using Ficoll-Hypaque by centrifugation at 600×g for 15 min. The monocytes collected from the interface were elutriated twice with ice-cold PBS. Purified cells were resuspended in DMEM containing 1% FCS and the cell concentration was adjusted to 5×105 cells/mL. The isolated populations were >85% pure examined with Wright-Giemsa stain; the purified cells were more than 90% viable and were identified with 0.4% Trypan blue.
Assay for monocyte adhesion For the adhesion studies, SMC were seeded in 24-well dishes and allowed to proliferate to confluence (approximately 48 h). Then the SMC were continuously incubated in DMEM medium supplemented with 1% (v/v) FBS without additives for 12 h before experi-mentation. The SMC were incubated in culture medium containing LPC and/or cariporide for 4 h. The assay of the adhesion rate of monocytes to SMC was conducted as previously described by Territo et al[13]. Briefly, the SMC were treated with different factors; they were eluted 3 times by PBS and 1 mL of the monocyte (5×105 cells) suspension of DMEM containing 5% FCS was added to each of the wells of the 24 well tissue culture plates. An additional 1 mL of the monocyte suspension was also saved for protein assay. Culture plates were incubated at 37 °C for 1 h and mixed gently every 15 min to ensure even dispersal of non-adherent monocytes. After incubation, the wells were rinsed twice with PBS containing Ca2+ and Mg2+ at 37 °C to remove the non-adherent monocytes. The SMC and adherent monocytes were then solubilized in 1 mL of 0.2 mol/L NaOH for subsequent protein analysis using the method described by Bradford[14]. The percentage of monocyte adhesion was then calculated by the micrograms of protein in the wells containing SMC and monocytes, minus the micrograms of protein in the wells containing SMC alone, divided by the micrograms of protein in 1 mL of the initial monocyte suspension.
Expression of adhesion molecules The expression of ICAM-1 in SMC was evaluated with a cell ELISA technique as described by Pigott et al[15]. The experimental protocol assay of monocyte adhesion to SMC was similar to that described earlier. The SMC were cultured in 96-well micro-plates and the confluence monolayer of SMC were subjected to treatment with or without LPC (5 µg/mL) in the presence or absence of cariporide for 4 h. The SMC were then rinsed twice with warm PBS containing 1% BSA and fixed with 0.15% glutaraldehyde for 15 min. The Supernatants were discarded and 100 µL of monoclonal antibody of ICAM-1 (5 µg/mL) was added to each well and incubated for 2 h at 37 °C. After being washed twice, the plates were added with 100 µL/well of peroxidase-conjugated goat antimouse IgG (1:2000) dilution in PBS containing 1% BSA and incubated at 37 °C for 1 h. The plates were washed 5 times with PBS containing 1% BSA, and 100 µL of O-phenylenediamine-H2O2 substrate was added to each well and incubated in a dark place for 15 min. H2SO4 2 mol/L was added at 50 µL/well to stop the reaction. The absorbance at 492 nm was measured using a microplate reader (Model 309 EL, Bio-Rad, california, USA) The value for ICAM-1 expression on normal SMC (without any additives) was normalized to 100%, and the value of ICAM-1 expression of SMC induced by additive agents was presented as increasing percentage plus 100%.
Measurement of intracellular pH value The measurement of [pH]i in SMC contained pH indicator BCECF and was performed according to the modified method described by Rink et al[16,17]. SMC were seeded on coverslips resting in a tissue culture dish. Confluent cells on coverslips were made quiescent by feeding DMEM medium with 1% (v/v) FBS for 24 h and treated with different factors for 4 h. The cells remaining adherent to the coverslips were incubated in HEPES buffer (NaCl 153.0 mmol/L, KCl 5.0 mmol/L, glucose 5.0 mmol/L, HEPES 10 mmol/L, 0.1% BSA; pH 7.4) and supplemented with 2 µmol/L of BCECF-AM at 37 °C for 30 min. The cells were then washed twice with HEPES buffer to remove unincorporated dye for fluorescent measurement and the coverslip was placed in a customized holder and inserted into a quartz resting in a water-jacketed cuvette holder (at 37 °C). Fluorescent intensity was monitored by a spectrofluorimeter (RF-5000, Shimadzu Co, Tokyo prefecture, Japan). The excitation wavelength was 505 nm (bandwidth 5 nm) and 440 nm (bandwidth 5 nm) and the emission wavelength was 530 nm (bandwidth 10 nm). The ratio of the 505 to 440 nm fluorescence values was used to estimate [pH]i. Over the pH range 6.4–7.8, the regression line relating to this fluorescence ratio to pH is linear. Known values for [pH]i of the SMC were obtained using buffers of various pH containing high levels of potassium HEPES solution (NaCl 20.0 mmol/L, KCl 120.0 mmol/L, glucose 10.0 mmol/L, HEPES 10 mmol/L, 0.1% BSA; pH 7.4) and 2 µg/mL of nigericin.
Experimental protocols In order to examine the effects of cariporide against monocytes adhering to SMC and the expression of ICAM-1 in the surface of SMC stimulated by LPC, the experiment was divided into 4 groups: the normal control group, in which SMC were incubated in normal culture medium; the LPC-treated control group, in which SMC were incubated in contained LPC (5 µg/mL) medium; the cariporide-alone control group, in which SMC were incubated in contained cariporide (20 µmol/L) culture medium; and the cariporide-treated group, in which SMC were incubated in a medium containing a series of concentrations of cariporide (2.5, 5, 10, 20 µmol/L) and LPC (5 µg/mL).
Statistical analysis All data were expressed as mean±SD and analyzed by one-way ANOVA and Newman-Keuls method. P<0.05 was considered significant difference, P<0.01 was considered as a very significant difference.
Results
Effects of LPC on monocytes adhering to SMC The adhesion ratio of monocytes with SMC was 15%±1.3% with the absence of LPC in the cultured medium. Treatment of SMC with LPC (1, 2.5, 5, and 10 µg/mL) for 1 h in the same conditions induced a dose-dependent increase of the adhesion ratio of monocytes, but when LPC was at 10 µg/mL, the adhesion ratio of monocytes to SMC was at its peak (Figure 1). The time-efficacy relationship of an increasing monocyte adhesion induced by LPC was also assessed. The adhesion ratio of monocytes to SMC increased with time delays of SMC exposure to LPC. A peak of monocyte adhesion ratio was apparent (44%±6%, P<0.01 vs absence of LPC) after 4 h of exposure to LPC, and the adherance ratio decreased when SMC were exposed to LPC for 6 h (Figure 2). Therefore, present experiments selected LPC at concentration of 5 µg/mL with an incubation time of 4 h as a stimulating factor of SMCs for an examination of effects of cariporide against adhesion.
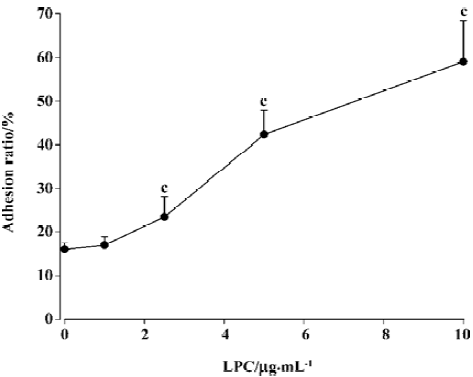
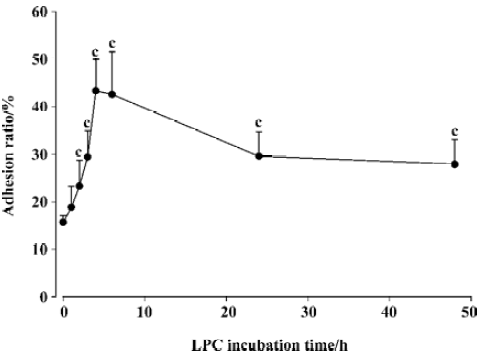
Inhibitory effects of cariporide on LPC-induced adhesion of monocytes to SMC The incubation of SMC with LPC alone (5 µg/mL) for 4 h resulted in an increase of 2.7 times in the percentage of monocytes adhering to SMC (P<0.01 vs normal control). Preincubation of SMC with cariporide (2.5 µmol/ L to 10 µmol/L) in the medium with the presence of LPC (5 µg/mL) for 4 h resulted in a dose-dependent inhibition on LPC-stimulated monocytes adhering to SMC. It was found that the threshold concentration of cariporide against LPC-stimulated adhesion of monocytes to SMC was 5 µmol/L, however, the inhibiting effect of cariporide at 10 µmol/L was achieved (22%±3%, P<0.01 vs normal control) (Figure 3). The incubation of SMC with cariporide alone had no effect on monocytes adhering to SMC.
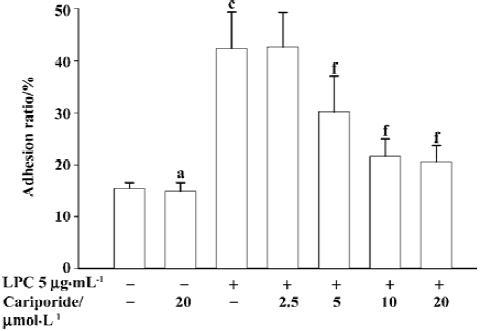
Effects of cariporide on LPC-induced expression of ICAM-1 As shown in Figure 4, after the exposure of SMC to LPC at 5 µg/mL for 4 h, the expression of ICAM-1 on the surface of the SMC significantly increased (216%±40%, P<0.01 vs normal group). The co-incubation of SMC with cariporide (5, 10, 20 µmol/L) and LPC (5 µg/L) resulted in a significant inhibition of LPC-stimulated expression of ICAM-1 on the surface of the SMC in a concentration-dependent manner of cariporide (Figure 4). The percentage of ICAM-1 expression in the groups where cariporide was present was 168%±22%, 134%±14% and 132%±15%, respectively (P<0.01 vs LPC-alone group). The expression of ICAM-1 in SMC was not affected by treatment with cariporide alone.
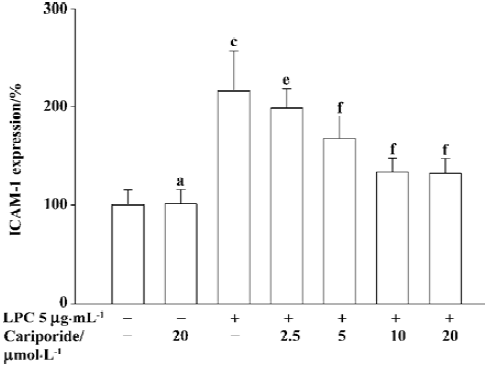
Effects of cariporide on [pH]i of SMC The exposure of SMC to LPC (5 µg/mL) for 4 h resulted in a significant increase of [pH]i in SMC (7.31±0.04 vs 7.19±0.01 of control baseline [pH]i, P<0.01). In contrast, after SMC were incubated with cariporide alone at 20 µmol/L for 4 h, [pH]i significantly decreased (7.094±0.012 vs normal baseline, P<0.01). After the SMC were co-incubated with different concentrations of cariporide and LPC(5 µg/mL) for 4 h, the [pH]i also decreased. The value of [pH]i in SMC with the presence of cariporide at concentration of 5, 10, and 20 µmol/L was 7.15±0.03, 7.113±0.017 and 7.102±0.018, respectively (P<0.01 vs LPC-alone group) (Figure 5).
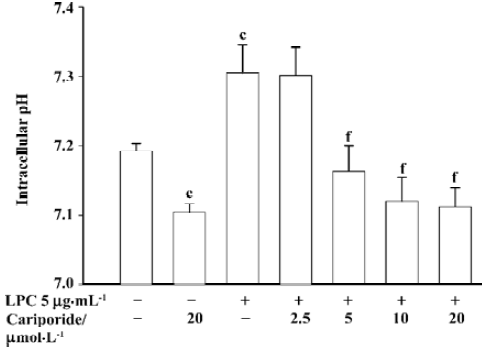
Discussion
Cariporide inhibits the interchange of sodium ions and protons in myocardial cells during unstable angina, myocardial infarction (MI), or ischemic damage due to angioplasty and reperfusion following thrombolysis. It has currently been evaluated in phase II/III clinical trials as a protective drug against MI[4,18]. However, there is little literature regarding the anti-atherosclerosis effects of sodium-hydrogen ion exchange inhibitors.
Our previous studies have demonstrated that cariporide markedly protects against the dysfunction of the endothelium-dependent of rabbit aortic artery injured by hyperchole-sterolemia, high glucose and relieved formation of atheroma plaques in high cholesterol-fed rabbits[6,7]. We also observed that cariporide had an inhibitory effect on the serum-stimulated proliferation of bovine aortic SMC in vitro[5]. These results show that cariporide has an anti-atherogenesis effect, but the mechanisms of anti-atherogenesis of cariporide has not properly been understood as yet.
It has been shown that both native and ox-LDL are potent atherogenic factors. The recruitment of monocyte-derived macrophages under endothelium hyperplasia and the migration of arterial SMC contribute to inflammation and the development of intimal hyperplasia during athero-sclerosis. In addition to endothelial cells, vascular SMC also play an important role in the development and progress of atherosclerosis[9,19,20]. It is known that the damage of endothelium-dependent relaxation of blood vessels and the formation of the atherosclerotic plaque caused by hypercholesterolemia is related to circulating ox-LDL. The ox-LDL promotes expression of adherent molecules on the surface of endothelium or smooth cells and stimulates monocytes adhering to the vascular wall. LPC is a major phospholipid component of ox-LDL, which is formed by hydrolysis of phosphatidylcholine (PC) in low-density lipoprotein cholesterol (LDL-C). Several experiments have proven that vascular SMC have the ability to express ICAM-1. LPC is able to mimic the effects of ox-LDL in vivo or in vitro, which can increase the expression of ICAM-1 on the surface of SMC and induce leukocyte rolling and adherence in the mesenteric rat microvasculature in vivo and stimulate monocytes binding to SMC in vitro[10]. Therefore ox-LDL and LPC are important factors in the pathogenesis of atherosclerosis and their signal transduction pathways may involve [Ca2+]i and the activation of the Na+/H+ exchanger[9,21].
In order to further explore the mechanisms of anti-atherogenesis of cariporide, we observed the effects of cariporide on human monocytes adhering to SMC and on the expression of ICAM-1 on the surface of cultured bovine aortic SMC stimulated by LPC.
The major findings in the present study are as follows: first, LPC significantly stimulated monocytes binding to SMC and enhanced expression of ICAM-1 on the surface of SMC, which was associated with an increase of [pH]i. Second, cariporid markedly prevented an increase of monocytes adhering to SMC and decreased expression of ICAM-1 on the surface of SMC stimulated by LPC. Third, cariporide simultaneously also blocked the elevation of [pH]i induced by LPC.
The results of the present study are also supported by other published studies. Jung et al[22] reported that acute and chronic treatment with cariporide significantly reduced the infarct size of myocardium induced by coronary artery occlusion in rabbits fed an atherogenic or normal diet, and chronic cariporide treatment also prevented dysfunction of endothelium-dependent relaxation induced by an atherogenic diet[23]. Kaloyianni et al[24] demonstrated that high glucose solution caused an activation of NHE-1 in human monocytes and in turn, stimulated the increase of the expression of CD36 receptors on the surface of monocytes from normal and obese individuals; it positively influenced monocyte migration and adhesion to laminin, the sodium/hydrogen ion exchange inhibitor. Cariporide or ethylisopropyl amiloride counteracted these effects caused by high glucose solution[23,24]. Based on these results, we presume that the activation of NHE-1 plays a crucial role in those effects induced by LPC. LPC may initially induce cytoplasm acidification, which in turn results in the activation of NHE-1 in the plasma membrane, extruding intracellular H+ in exchange for extracellular Na+, followed by an increase of [pH]i. It then provokes an intracellular Na+ concentration elevation, which in turn activates reverse-mode Na+/Ca2+ exchange, leading to an influx of Ca2+ into cells and intracellular Ca2+ overload[2,3]. A rise in intracellular calcium may act as a second messenger to induce expression of adherent molecules and the activation of SMC. Cariporide-inhibited activation of NHE-1 may attenuate an LPC-induced intracellular calcium overload, sequentially alleviating the expression of adherent molecules and adhesion of monocytes. Our study did not directly measure changes of intracellular calcium concentration, but it was shown that LPC induced an elevation of [pH]i in SMC. This assumption is also supported by the following cases: LPC dose-dependently induced a sustained increase in intracellular calcium in cultured SMC[22,25].
The present study shows that stimulative effects of LPC on the expression of ICAM-1 and monocytes adhering to SMC are related to the activation of NHE-1 in SMC. Cariporide is able to inhibit LPC-induced expression of ICAM-1 in cultured SMC and monocytes adhering to SMC. The atherosclerosis is a complex disease involving multiple factors. We believe that the activation of NHE-1 may participate in the progress of atherosclerosis; the selective NHE-1 inhibitors may serve as new drugs for the treatment of atherosclerosis. Our results provide some basis for anti-atherosclerotic effects of cariporide. Further studies may prove this possibility.
References
- Gan XT, Chakrabarti S, Karmazyn M. Modulation of Na+/H+ exchange isoform 1 mRNA expression in isolated rat hearts. Am J Physiol 1999;277:H993-8.
- Linz WJ, Busch AE. NHE-1 inhibition: from protection during acute ischaemia/reperfusion to prevention/reversal of myocardial remodeling. Naunyn Schmiedebergs Arch Pharmacol 2003;368:239-46.
- Karmazyn M, Sawyer M, Fliegel L. The Na(+)/H(+) exchanger: a target for cardiac therapeutic intervention. Curr Drug Targets Cardiovasc Haematol Disord 2005;5:323-35.
- Karmazyn M. Pharmacology and clinical assessment of caripo-ride for the treatment of coronary artery diseases. Expert Opin Investig Drugs 2000;9:1099-108.
- Tu JH, Liu LY. Selective sodium/hydrogen exchanger inhibitor HOE642 inhibited proliferation of vascular smooth muscle cells. Chin J Pharmacol Toxicol 2001;15:260-4.
- Wang SX, Xiong XM, Song T, Liu LY. Protective effects of cariporide on endothelial dysfunction induced by high glucose. Acta Pharmacol Sin 2005;26:329-33.
- Tu JH, Liu LY, Zhang XH. Protective effect of Na(+)-H+ exchanger inhibitor cariporide on the injury of vascular endothelial function induced by hypercholesterolemia. J Hu-nan Med Univ 2002;27:13-6. (in Chinese).
- Ross R. The pathogenesis of atherosclerosis: A perspective for the 1990s. Nature 1993;362:801-9.
- Gouni-Berthold I, Sachinidis A. Possible non-classic intracellular and molecular mechanisms of LDL cholesterol action contributing to the development and progression of atherosclerosis. Curr Vasc Pharmacol 2004;2:363-70.
- Scalia R, Murohara T, Campbell B, Kaji A, Lefer AM. Lysophos-phatidylcholine stimulates leukocyte rolling and adherence in rat mesenteric microvasculature. Am J Physiol 1997;272:H2584-90.
- Watanabe T, Pakala R, Katagiri T, Benedict CR. Lysophosphati-dylcholine is a major contributor to the synergistic effect of mildly oxidized low-density lipoprotein with endothelin-1 on vascular smooth muscle cell proliferation. J Cardiovasc Pharmacol 2002;39:449-59.
- Fogelman AM, Elahi F, Sykes K, Van Lenten BJ, Territo MC, Berliner JA. A modification of the Recalde method for the isolation of human monocytes. J Lipid Res 1988;29:1243-7.
- Territo MC, Berliner JA, Almada L, Ramirez R, Fogelman AM. β-very low density lipoprotein pretreatment of endothelial monolayers increases monocyte adhesion. Atherosclerosis 1989;9:824-8.
- Bradford MM. A rapid and sensitive method for the quantitation of microgram quantities of protein utilizing the principle of protein-dye binding. Anal Biochem 1976;72:248-54.
- Pigott R, Needham LA, Edwards RM, Walker C, Power C. Structural and functional studies of the endothelial activation antigen endothelial leukocyte adhesion molecule-1 using a panel of monoclonal antibodies. J Immunol 1991;147:130-5.
- Rink TJ, Tsien RY, Pozzan T. Cytoplasmic pH and free Mg2+ in lymphocytes. J Cell Biol 1982;95:189-96.
- Thomas JA, Buchsbaum RN, Zimniak A, Racker E. Intracellular pH measurements in Ehrlich ascites tumor cells utilizing spectroscopic probes generated in situ. Biochemistry 1979;18:2210-8.
- Chin B, Lip GY. Cariporide aventis. Curr Opin Investig Drugs 2000;1:340-6.
- Kinard F, Jaworski K, Sergent-Engelen T, Goldstein D, Van Veldhoven PP, Holvoet P, et al. Smooth muscle cells influence monocyte response to LDL as well as their adhesion and transmigration in a coculture model of the arterial wall. J Vasc Res 2001;38:479-91.
- Morelli PI, Martinsson S, Ostergren-Lunden G, Friden V, Moses J, Bondjers G, et al. IFNgamma regulates PDGF-receptor alpha expression in macrophages, THP-1 cells, and arterial smooth muscle cells. Atherosclerosis 2006;184:39-47.
- Gan XT, Chakrabarti S, Karmazyn M. Modulation of Na+/H+ exchange isoform 1 mRNA expression in isolated rat hearts. Am J Physiol 1999;277:H993-8.
- Jung O, Albus U, Lang HJ, Busch AE, Linz W. Effects of acute and chronic treatment with the sodium hydrogen exchanger 1 (NHE-1) inhibitor cariporide on myocardial infarct mass in rabbits with hypercholesterolaemia. Basic Clin Pharmacol Toxicol 2004;95:24-30.
- Koliakos G, Zolota Z, Paletas K, Kaloyianni M. High glucose concentrations stimulate human monocyte sodium/hydrogen exchanger activity and modulate atherosclerosis-related functions. Pflugers Arch 2004;449:298-306.
- Kaloyianni M, Zolota Z, Paletas K, Tsapas A, Koliakos G. Cariporide counteracts atherosclerosis-related functions in monocytes from obese and normal individuals. Obes Res 2005;13:1588-95.
- Lee KU, Lee IK, Han J, Song DK, Kim YM, Song HS, et al. Effects of recombinant adenovirus-mediated uncoupling protein 2 over expression on endothelial function and apoptosis. Circ Res 2005;96:1200-7.