Phenotypic and molecular characterization of 5 novel CTX-M enzymes carried by Klebsiella pneumoniae and Escherichia coli1
Introduction
Resistance to the expanded-spectrum cephalosporins can occur in Escherichia coli (E. coli) and Klebsiella species via the production of expanded-spectrum β-lactamases (ESBL) that are capable of hydrolyzing oxyimino-cephalosporins and monobactams[1,2]. The CTX-M-β-lactamases are a group of molecular class A ESBL that exhibit an overall preference for hydrolysis of cefotaxime (hence the CTX-M name) and ceftriaxone and a higher susceptibility to tazobactam than to clavulanic acid. CTX-M-β-lactamases have been recognized and reported in the literature with increasing frequency[2,3]. This resistance mechanism is widespread throughout the world, with reports of clinical isolates producing these β-lactamases from Europe, Africa, Asia, South America, and most recently in North America[3,4].
The blaCTX-M genes are often carried on transferable plasmids [5]. Two of them (blaCTX-M-2 and blaCTX-M-9) were found to be associated with complex class 1 integrons related to In6 and In7, although they are not found on typical gene cassettes[5]. CTX-M-β-lactamases are not closely related to Temoniera (TEM) or Sulphydryl variable (SHV) ESBL but share high amino acid identity with chromosomal β-lactamases from Kluyvera georgiana, Kluyvera cryocrescens, and Kluyvera ascorbata[6−9]. According to a recent review and new data within GenBank, CTX-M-β-lactamases can be divided into 5 groups based on their amino acid sequence identities[3]. Group I includes CTX-M-1, -3, -10 to -12, -15 (UOE-1), -22, -23, -28, -29, and -30. Group II includes CTX-M-2, -4 to -7, and -20 and Toho-1. Group III includes CTX-M-8. Group IV includes CTX-M-9, -13, -14, -16 to -19, -21, and -27 and Toho-2. Finally, group V includes CTX-M-25 and -26 (http://www.lahey.org/studies/). The members of these groups exhibit >94% amino acid identity within the group and members of different lineages differ at 10%?30% of the amino acid residues[3].
In our previous report, 5 strains of Klebsiella pneumoniae (K. pneumoniae) and 3 strains of E. coli produced 5 novel CTX-M enzymes were identified in our area[10]. These novel enzymes came from CTX-M-14 with 1−3 amino acid substitution by sequencing and the BLAST program (http://www.ncbi.nlm.nih.gov/BLAST/). We suspected that clonal outbreak had occurred among those isolates, but did not have adequate evidence to confirm this hypothesis. Thus, in the present study, we sought to further characterize the novel CTX-M enzymes involved and determine the molecular epidemiology of these strains.
Materials and methods
Bacterial strains Five K. pneumoniae isolates and 3 E coli isolates were collected from 3 hospitals; 1 hospital was a tertiary-care teaching hospital with over 1000 beds, and the other two were tertiary-care hospitals with more than 500 beds in Hefei, Anhui province, between September 1999 and September 2000. Eight strains were from the hospitalized patients, including 5 from sputum and 3 from urine samples (Table 1). The isolates were identified by using the Microscan Walkaway-40 System (Dade Behring, West Sacramento, California, USA). All of the strains were confirmed non-repeated and clinical significance isolates. E. coli C600, E. coli DH5a, and E. coli JM109 were stored by the Anhui Center for Surveillance of Bacterial Resistance (Hefei, China).
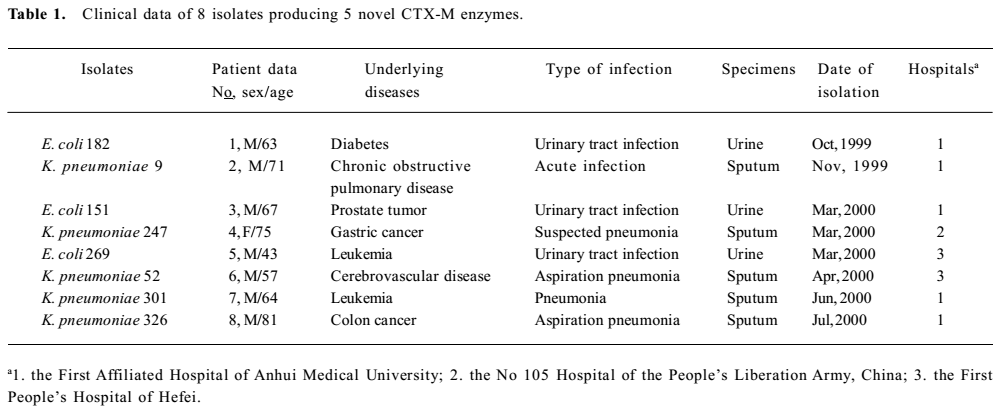
Full table
Conjugation experiment To determine whether resistance was transferable, conjugations were performed with a streptomycin-resistant recipient, E. coli C600 (lac-) as the recipient. Donor strains in the logarithmic phase of growth were mixed with recipients in the early stationary phase in a 1:10 ratio in Muller-Hinton broth (Oxiod, Basingstoke, Hampshire, UK), and the mixture was incubated at 37 ℃ for 14 h[11]. Conjugation mixtures were plated on MacConkey agar (Tianhe, Hangzhou, China) containing streptomycin (500 µg/mL) and cefotaxime (2 µg/mL) and then incubated for approximately 20 h at 37 ℃.
PCR amplification and bla gene sequencing Plasmid DNA from different K. pneumoniae and E. coli strains was used as the template in the PCR amplification. The oligonucleotides used as primers for amplification and sequencing are shown in Table 2. A search for the blaTEM, blaSHV, blaCTX-M-9, blaOXA-1, blaOXA-2, and blaOXA-10 genes in the clinical isolates was performed by PCR amplification, as described previously. The detection of the ampC gene was performed as described by Bou and Martinez-Beltran[12,13].
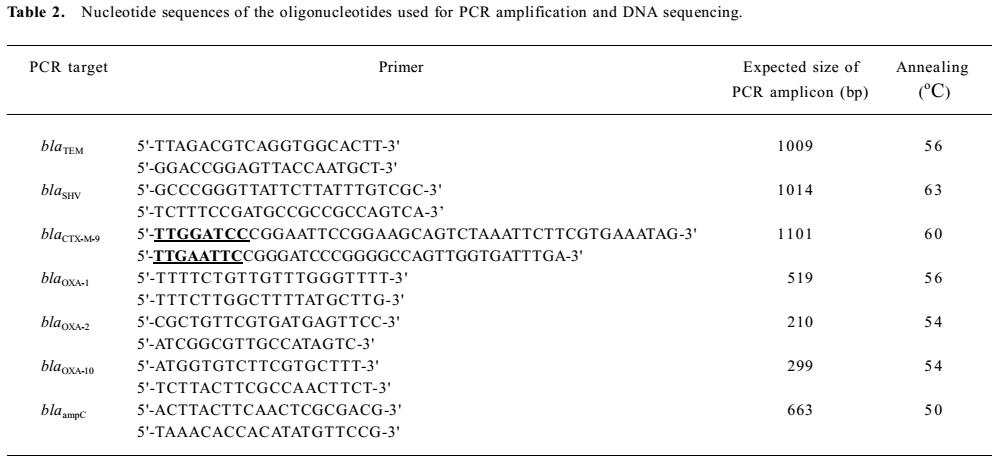
Full table
The entire CTX-M genes from the screening for the CTX-M-9 group gene positive strains were sequenced directly from PCR amplified DNA. Primers were used for the amplification of 1101 bp products containing the whole CTX-M-9 group open reading frame (ORF). Plasmid DNA extracted from 8 clinical isolates and their transconjugant by rapid alkaline lysis protocol was used as the template. PCR amplification was carried out under the conditions as previously described[10]. The purified PCR products were ligated with pGEM-Teasy vectors (Promega, Madison, Wisconsin, USA) and expressed in E. coli DH5a. All nucleotide sequences were determined by the bidirectional sequencing of PCR production with the 3730 automatic DNA sequencer (Sangon, Shanghai, China). The sequences were compared with the sequence of the CTX-M-14 gene (AF252622).
Cloning of the CTX-M gene To ascertain the resistant characteristic of the novel enzymes, the transform experiment was performed as described by Ishii et al[14]. For cloning the novel CTX-M genes, the whole ORF amplicon was linked into the vector pHSG398 (2227bp) by T4 DNA ligase (TaKaRa, Dalian, China) after cleavage by EcoRI and BamHI restriction enzymes (TaKaRa, China). Then, the recombinant plasmid was introduced into E. coli JM109 made competent by the calcium chloride method. After transformation, a few clones grew on Luria-Bertani (L-B) agar plates supplemented with cefotaxime (2 µg/mL) and chloromycetin (50 µg/mL). They harbored an identical plasmid with an insert of approximately 1101 bp. These plasmids were used as templates to determine the nucleotide sequence in the 3730 automatic DNA sequencer (Sangon, China).
Antimicrobial susceptibility tested The minimal inhibitory concentrations (MIC) of antimicrobial agents were determined by the broth dilution method according to the guidelines of the Clinical and Laboratory Standards Institute (CLSI), 2005[15]. The MIC was defined as the lowest concentration that prevented visible growth after incubation for 16‒20 h at 35 ℃. The antimicrobial agents were as follows: penicillin G, piperacillin, cefuroxime, ceftriaxone, cefotaxime, ceftazidime, cefepime, imipenem, aztreonam, tazobactam, ciprofloxacin, and levofloxacin (National Institute for the Control of the Pharmaceutical and Biological Products, Beijing, China), clavulanic acid (Glaxo Smith Kline, London, UK), cephalothin (Sigma, St Louis, MO, USA). The concentration of tazobactam and clavulanic acid was tested with a fixed concentration of 4µg/mL, respectively. All antimicrobial agents were incorporated into cation-adjusted Mueller-Hinton broth in serial 2-fold concentrations from 0.06 to 256 µg/mL. The quality control strains were E. coli ATCC 25922 and E. coli ATCC 35218 with every batch of clinical isolates to ensure the accurate and comparable performance of assays[15]. The inoculating concentration of bacteria was approximate 1.5×108 CFU/mL, equivalent to a 0.5 McFarland standard. The final concentration of inoculum was 5×105 CFU/mL. Then 1 mL of the adjusted inoculum was added to each tube containing l mL of antimicrobial agents in the dilution series and mixed.
The ESBL production of the transformants was detected by phenotypic confirmatory tests (cation-adjusted Mueller-Hinton broth dilution test) as recommended by the CLSI, 2005. E. coli ATCC 25922 and K. pneumoniae ATCC 700603 were used as negative and positive controls, respectively[15].
β-Lactamase preparation CTX-M mutant-encoding genes overexpressed in E. coli JM109 were grown in 0.1 L of L-B broth containing cefotaxime at 2 mg/mL for 18 h at 37 oC. The bacteria collected by centrifugation were suspended with 0.1 mmol/L phosphate-buffered saline (PBS) and disrupted by ultrasonic treatment (15 times for 10 s, each time at 20 W). After centrifugation at 20000×g for 60 min at 4 oC, the CTX-M purification was carried out as previously described[16] by ion-exchange chromatography with an SP sepharose column (Amersham Pharmacia Biotech, Piscataway, New Jersey, USA).
Determination of β-lactamase kinetic constants β-lactamase activity was determined spectrophotometrically by measuring the change in absorbency at different wavelengths for penicillin G (231 nm), cephalothin (266 nm), cefuroxime (271 nm), cefotaxime (234 nm), and ceftazidime (258 nm) in a SP-752 type recording spectrophotometer (Spectrum Instruments, Shanghai, China). The reaction mixtures were maintained at 30 oC (pH 7.0) by means of a circulating water bath, and the reaction was started by the addition of the enzyme. For all of the assays, the controls were performed by omitting from the reaction mixture the specific substrate. Kinetic parameters were estimated from a least-squares fit of Lineweaver-Burk plots with a substrate concentration ranging from 0.01 to 0.10 mmol/L[17].
Isoelectric focusing and enzyme inhibition assay Isoelectric focusing (IEF) was carried out with polyacrylamide gel containing ampholytes with a pH range of 3.0?10 (Amersham Pharmacia Biotech, USA) for the new enzymes as previously described[18]. The pellet was resuspended in 1 mL phosphate buffer (pH 7.0, 10 mmol/L), and sonicated for 10 min in a sonicator (Sonics & Material, Newtown, Connecticut, USA) in ice-cold water. After IEF, β-lactamases were detected by spreading nitrocefin (Oxiod, UK) on the gel surface. Isoelectric points (pI) were determined by comparison with those of β-lactamases with known pI: TEM-1 (pI 5.4), SHV-1 (pI 7.6), SHV-5 (pI 8.2), SHV-18 (pI 7.8), and CTX-M-5 (pI 8.8), and were calculated by using CurveExpert 1.3 software. An inhibition assay was carried out by overlaying the gels with 0.5 mmol/L nitrocefin with or without 0.3 mmol/L clavulanic acid in 0.1 mmol/L PBS (pH 7.0).
Plasmid profiling and restriction fragment length polymorphism of plasmid DNA A restriction analysis of the CTX-M-containing plasmids was carried out on plasmids extracted from transconjugants by a rapid alkaline lysis procedure. Transferable plasmids were purified, and purified plasmid DNA was digested with the PstI restriction enzyme (TaKaRa, China). DNA restriction fragment length polymorphisms (RFLP) were analyzed by electrophoresis on 0.8% agarose gels stained with ethidium bromide at 45 V for 16 h at 20 ℃.
Pulsed-field gel electrophoresis analysis Chromosomal DNA was prepared as previously described and digested with the XbaI restriction enzyme (TaKaRa, China)[19,20]. DNA fragments were separated by electrophoresis in 0.8% agarose gels (Sangon, China) and 0.5×Tris-Borate-EDTA (0.5×TBE) buffer by using a contour-clamped homogeneous electric field (Bio-Rad, Hercules, California, USA) according to the following electrophoresis conditions: 12 ℃ at 6 V/cm for 27 h with the pulse time ranging from 10 to 40 s. The DNA bands were visualized by staining of the gel with ethidium bromide and were then photographed. Clonal relationships were established, a criterion recommended by Tenover et al[19].
Results
The transferability of the CTX-M determinants was assayed in conjugation experiments using an E. coli recipient and selection of transconjugants at similar frequencies of 1×10-3−1×10-8. CTX-M genes were detected by PCR in 8 E coli C600 transconjugants. The results of the DNA sequencing with the whole ORF primer indicated that production of the CTX-M-9 group was almost identical to that of CTX-M-14. One to three point mutations occurred in 8 isolates, resulting in the amino acid substitutions compared with CTX-M-14 (AF252622), as shown in Table 3. Five novel CTX-M enzymes were determined as CTX-M-46 (AY847147), CTX-M-47 (AY847143), CTX-M-48 (AY847144), CTX-M-49 (AY847145), and CTX-M-50 (AY847146). The blaTEM genes were amplified from 8 studied isolates. All isolates were found to carry TEM-1 by nucleotide sequencing, and one was shown to harbor SHV-12 by sequence analysis. The genotypes of all transconjugants were consistent with those of their donors.
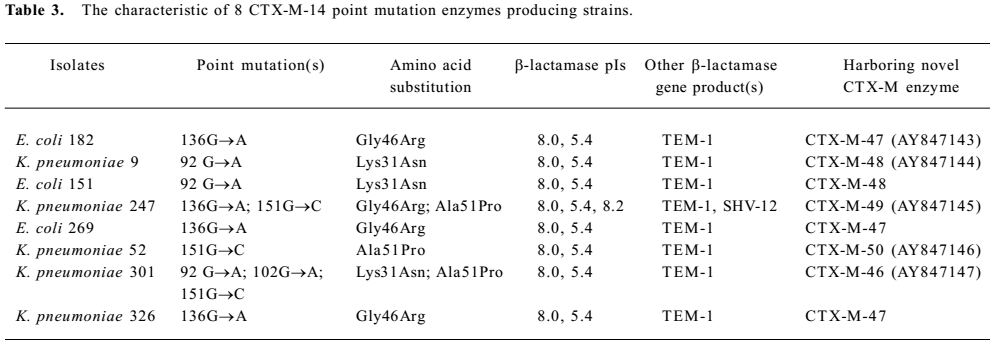
Full table
All of the wild-type strains and the transconjugants had the same resistance spectrum, which exhibited the same high resistant rate to piperacillin, ceftriaxone, and cefotaxime. All of the strains were susceptible to imipenem, but compared with the wild-type strains, the transconjugants decreased the resistant ability to all of the antimicrobial agents and obviously enhanced the susceptibility to fluoroquinolones. Five recombinant plasmids containing novel blaCTX-M were confirmed to clone into the competent cell (E. coli JM109) successfully by PCR methods. In the E. coli transformants, the MIC for ceftazidime were low (ranging from 1 to 4 µg/mL), which is characteristic for most CTX-M enzymes, and significant synergy with β-lactamases inhibition was observed with β-lactam antimicrobial agents (piperacillin, cefotaxime, and ceftazidime; Table 4).
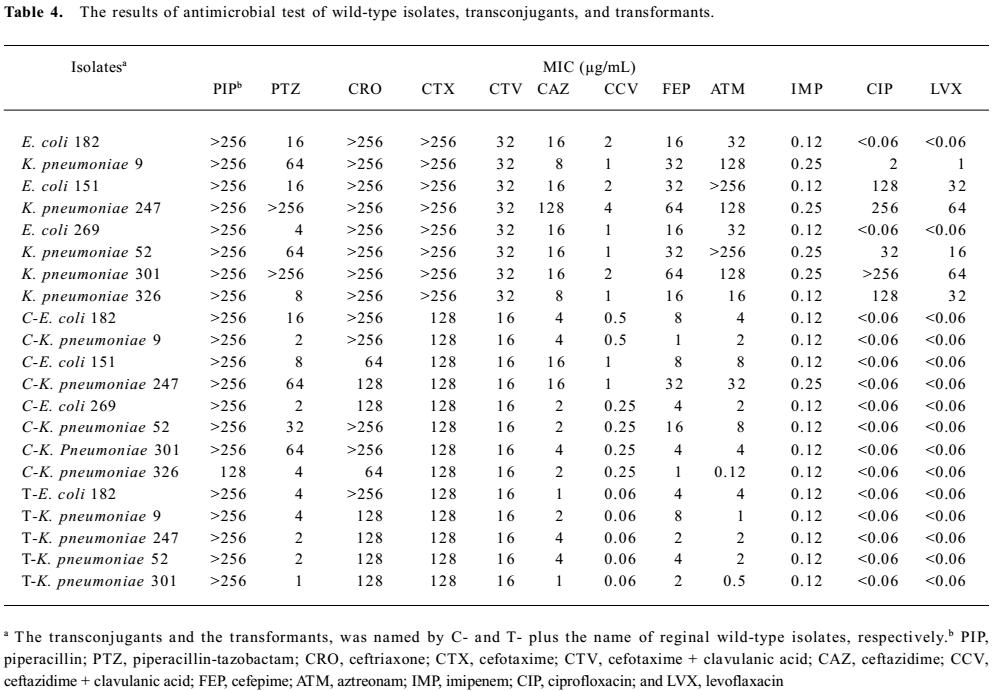
Full table
Phenotypic confirmatory testing for the ESBL production of transformants requires use of both cefotaxime and ceftazidime, alone and in combination with clavulanic acid. There was a ≥3 2-fold concentration decrease in a MIC for either antimicrobial agent tested in combination with clavulanic acid versus its MIC when tested alone. The results of the antimicrobial test showed that 5 novel CTX-M enzymes were typical for class A ESBL (Table 4).
The kinetic parameters of 5 novel CTX-M-β-lactamases were determined for a representative set of β-lactam antimicrobial agents (Table 5). The results showed that the β-lactamase exhibited a broad-spectrum activity profile, although with notable differences for different substrates. Common enzymatic features included better affinities for penicillin G than for cephalothin, cefuroxime, cefotaxime, and ceftazidime. Cephalothin was the best substrate; and the rate of hydrolysis of ceftazidime was too slow to obtain an accurate Km value.
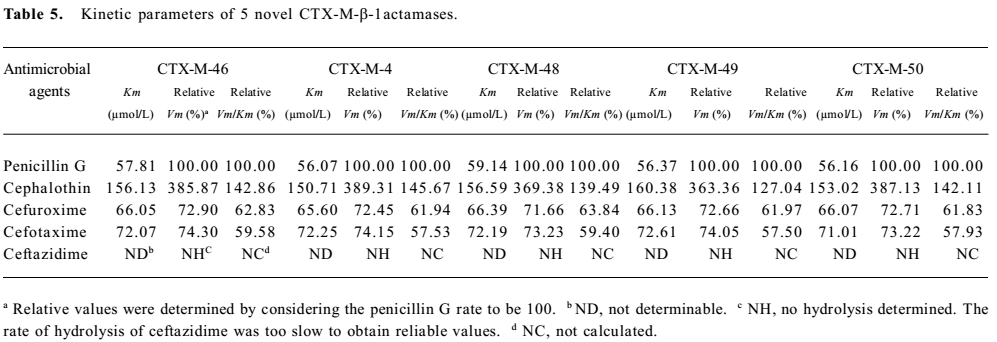
Full table
On the IEF gels, all β-lactamases produced by the 8 classical ESBL-producing isolates were inhibited by 0.3 mmol/L clavulanic acid, so the CTX-M-containing isolates that had pI 8.0 enzymes were represented CTX-M-14-derivative enzymes. Most isolates expressing CTX-M enzyme were found to produce additional β-lactamases (Table 3). The enzymes with a pI of 5.4 detected in the 8 isolates were not inhibited by clavulanic acid and thus were tentatively classified as broad-spectrum β-lactamases. A further enzyme with a pI of 8.2 was inhibited by clavulanic acid. Thus, it was considered to carry classical ESBL. The genotypes of all transconjugants were consistent with those of their donors. The pI of all transconjugants were consistent with 8 wild-type strains, and the pI of 8.0 were observed in transformants.
Purified plasmids from 8 transconjugants of strains were digested with PstI, and the resulting fragments were separated on 0.8% agarose gels (Figure 1). The overall results showed a similarity between plasmids in E. coli 269 and E coli 182, thus suggesting identity in the plasmids harboring CTX-M-47 on these strains. However, the other 6 transconjugants did not show similarity in their RFLP patterns. Therefore, the CTX-M-46, CTX-M-48, CTX-M-49, and CTX-M-50 genes were harbored in different plasmids on these strains, and so the possibility that a mobile genetic element might be involved in the dissemination of the CTX-M-14-derivation gene in these plasmids cannot be ruled out.
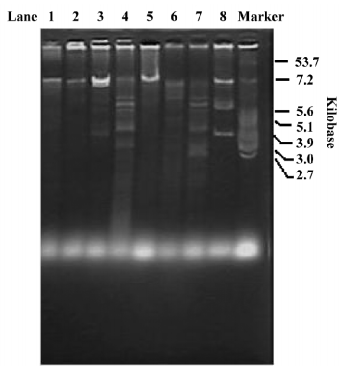
Pulsed-field gel electrophoresis analysis (PFGE) was performed to determine whether clonal spreading was responsible for the dissemination of CTX-M-14 derivatives. Based on the criteria previously described by Tenover et al[15], that is, differences of no more than 3 bands belonged to the epidemiologically-related strains, and differences of more than 6 bands belonged to the epidemiologically-unrelated strains. The 8 CTX-M-14-derivative strains isolated from 3 hospitals belonged to different clones, except for E. coli 269 and E. coli 182, which exhibited closely-related isolates (Figure 2).
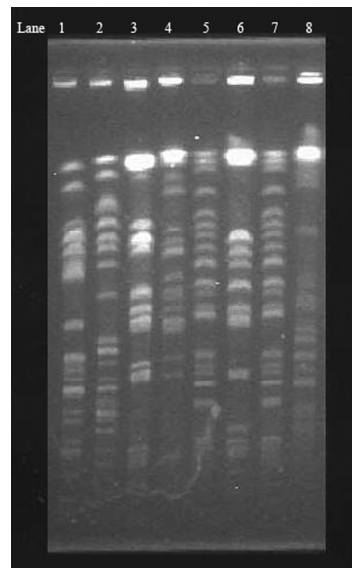
The sources from which the 8 isolates producing 5 novel CTX-M enzymes were recovered, as well as the selected clinical features of the patients carrying these organisms, are summarized in Table 1. Positive cultures of CTX-M enzyme producers were obtained from 8 patients after 72 h of hospitalization. CTX-M-48 producers were isolated from 2 patients in the Intensive Care Unit (ICU). Patient 3 had accepted urethral catheterization. Patients 1, 5, and 8 had been hospitalized for 3 to 6 months before clinical presentations of their infections. Patient 4 had little history of hospitalization before she underwent gastrectomy in hospital. Since it was not known that they were infected with ESBL producers, patient 6 was treated with piperacillin, cefotaxime, and ceftriaxone, and patient 7 was treated with cefuroxime, cefotaxime, ciprofloxacin, and levofloxacin.
Discussion
In recent reports, ESBL phenotypes were detected in 45% of K. pneumoniae strains from Latin America, 23% from the Western Pacific, 23% from Europe, 8% from the United States, and 5% from Canada[21]. CTX-M enzymes were initially isolated from strains in Europe and Argentina in the late 1980s and early 1990s[22]. Since then, the plasmid-encoded CTX-M enzyme, which is one of the most prevalent types in Asian countries, has now been encountered on 5 continents owing to the ease of global travel; blaCTX-M may easily be spread worldwide as a means to adapt to environmental hazards for bacterial growth and has a high survival capacity in the environment. The inherent ability of K. pneumoniae and E. coli may facilitate the development of resistance profiles with the widespread use of antimicrobial agents through the selection of strains with ever-accumulating antimicrobial resistance profiles in hospital environments. Numerous reports from eastern Europe, Asia, and South America have identified CTX-M-type ESBL.
The wild-type isolates, transconjugants, and transformants of our data exhibited a moderate or high resistance to cefotaxime and ceftriaxone; however, most of them are susceptible to ceftazidime and aztreonam, except for E. coli 151, K. pneumoniae 247, and K. pneumoniae 301. We consider that the reason is other ESBL involved in the resistant patterns. K. pneumoniae 247, producing the SHV-12 enzyme, obviously accounts for the resistance ability to ceftazidime. E. coli 151 and K. pneumoniae 301, with broad-spectrum β-lactamase (TEM-1), were simultaneously detected. This could not be easily explained and questions still remain. Therefore, we supposed that multicopies of plasmids or/and the hyperproduction of TEM-1 in the wild-type strain may account for the resistant property, which is resistant to ceftazidime, and found that aztreonam declined in its transconjugants[23]. In addition, the wild-type isolates and the transconjugants displayed resistance to the 2 fluoro-quinolones (ciprofloxacin and levofloxacin). We can clearly see the difference between the 2 groups of strains: most of the wild-type strains were resistant to ciprofloxacin and levofloxacin. However, all of the transconjugants were susceptible to ciprofloxacin and levofloxacin. Therefore, we infer that the resistance to ciprofloxacin and levofloxacin in our strains is not plasmid-borne, but chromosomally mediated.
Like CTX-M-9, CTX-M-14, has been described in China, Korea, France, Japan, and Spain since 2002[24]. Enzymes of the CTX-M-9 group were defined as a pI ranged from 7.9 to 8.2, and had a higher catalytic activity against cefotaxime than against ceftazidime and aztreonam. To our knowledge, amino acid residues Asn104, Asn132, Phe160, Gly232, Ser237, and Arg276 are thought to play an important role in the catalytic properties in the CTX-M β-lactamases[16,25]. The substitution of Ser237, which is known to enhance hydrolysis of cefotaxime, is observed in CTX-M-14[16,26]. The amino acid substitutions at positions 164, 179, 238, and 240[27], which are associated with the expansion of the spectrum of activity towards oxyimino-cephalosporins and aztreonam in TEM- and SHV-type ESBL [16,28], were not observed in CTX-M-46 to CTX-M-50, for which the MIC of aztreonam and ceftazidime were low. In our report, the 3 substitution sites (Lys31Asn, Gly46Arg, and Ala51Pro) do not belong to the active sites or are not adjacent to the indirect active sites, such as the Ω loop, which plays an important role in influencing substrates, so we supposed that the substitutions would not result in the resistance pattern changing, or a neutral mutation. From our data, we also concluded that cefotaxime was the specific substrate to the 5 CTX-M-14 derivatives as their parent enzymes.
In this study, the PFGE of XbaI-digested genomic DNA and the electrophoresis of the PstI-digested blaCTX-M-containing plasmid showed that 8 isolates and the resistant plasmids had diverse patterns despite 1-3 amino acid substitutions occurring intensively from the same parent enzyme from the same hospital. The closely-related restriction patterns only were found between strains of E. coli 269 and E coli 182, which were obtained from the First Affiliated Hospital of Anhui Medical University and the First People抯 hospital of Hefei, respectively. As both are teaching hospitals, the patients, interchange between the 2 hospitals was frequent. We excluded 2 isolates obtained from the same patient (their addresses were indefinite). Therefore, we conclude that CTX-M-14-derivatives could be the multiplex genesis or 2 patients could come from the same area, but the definite mechanisms that 8 CTX-M-14 derivatives occurred remain unknown.
Spreading between patients can be easy when a bacterium with an antimicrobial resistance mechanism has been established, especially if this mechanism is associated with plasmids or other mobile genetic elements. We should alert the medical community of the increase of these β-lactamases in our area (especially isolates collected from patients with underlying diseases in the hospital environment, such as ICU). These hospitals are large-scale general hospitals, and the interchange of patients who come from other areas between them is frequent. Therefore, it is necessary to strengthen the surveillance of antimicrobial resistance in local areas and exchange data between different areas. There is the extremely important epidemiology significance in this work to prevent dissemination of resistant genes.
Five novel CTX-M enzymes are clinical variants found in Anhui Province. The continuing evolution of genes encoding ESBL which are caused by the misuse of antimicrobial agents (especially the empirical administration of cefotaxime in serious infections) are reflected in the increasingly large number of derivative of β-lactamases, and their widespread dissemination on resistant plasmids significantly limits therapeutic choices. The rational use of other antimicrobial agents may improve the situation. In addition, this research emphasizes that improving the detection of ESBL with molecular procedures and the implementation of appropriate infection control procedures will provide a more accurate assessment of their prevalence and lead to more rational uses of antimicrobial agents in order to prevent clinical complications[29], which in turn will reduce the selection and spread of organisms producing these enzymes. We also should increase efforts in surveillance and the study of risk factors (ie hospitalized time and invasive operation) associated with the acquisition of these isolates. This will guide future prevention and control measures, which will continue to present challenges for clinical microbiologists and clinicians alike[30].
References
- Pitout JD, Hossain A, Hanson ND. Phenotypic and molecular detection of CTX-M-β-lactamases produced by Escherichia coli and Klebsiella spp. J Clin Microbiol 2004;42:5715-21.
- Bush K. New beta-lactamases in gram-negative bacteria: diversity and impact on the selection of antimicrobial therapy. Clin Infect Dis 2001;32:1085-9.
- Bonnet R. Growing group of extended-spectrum beta-lactamases: the CTX-M enzymes. Antimicrob Agents Chemother 2004;48:1-14.
- Moland ES, Black JA, Hossain A, Hanson ND, Thomson KS, Pottumarthy S. Discovery of CTX-M-like extended-spectrum beta-lactamases in Escherichia coli isolates from five US states. Antimicrob Agents Chemother 2003;47:2382-3.
- Tzouvelekis LS, Tzelepi E, Tassios PT, Legakis NJ. CTX-M-type beta-lactamases: an emerging group of extended-spectrum enzymes. Int J Antimicrob Agents 2000;14:137-42.
- Pagani L, Dell’Amico E, Migliavacca R, D’Andrea MM, Giacobone E, Amicosante G, et al. Multiple CTX-M-type extended-spectrum beta-lactamases in nosocomial isolates of Enterobacteriaceae from a hospital in northern Italy. J Clin Microbiol 2003;41:4264-9.
- Poirel L, Kämpfer P, Nordmann P. Chromosome-encoded Ambler class A beta-lactamase of Kluyvera georgiana, a probable progenitor of a subgroup of CTX-M extended-spectrum beta-lactamases. Antimicrob Agents Chemother 2002;46:4038-40.
- Decousser JW, Poirel L, Nordmann P. Characterization of a chromosomally encoded extended-spectrum class A beta-lactamase from Kluyvera cryocrescens. Antimicrob Agents Chemother 2001;45:3595-8.
- Humeniuk C, Arlet G, Gautier V, Grimont P, Labia R, Philippon A. Beta-lactamases of Kluyvera ascorbata, probable progenitors of some plasmid-encoded CTX-M types. Antimicrob Agents Chemother 2002;46:3045-9.
- Li H, Li JB. Detection of five novel CTX-M-type extended spectrum beta-lactamases with one to three CTX-M-14 point mutations in isolates from Hefei, Anhui Province, China. J Clin Microbiol 2005;43:4301-2.
- Liebana E, Gibbs M, Clouting C, Barker L, Clifton-Hadley FA, Pleydell E, et al. Characterization of beta-lactamases responsible for resistance to extended-spectrum cephalosporins in Escherichia coli and Salmonella enterica strains from food-producing animals in the United Kingdom. Microb Drug Resist 2004;10:1-9.
- Nagano N, Nagano Y, Cordevant C, Shibata N, Arakawa Y. Nosocomial transmission of CTX-M-2 beta-lactamase-producing Acinetobacter baumannii in a neurosurgery ward. J Clin Microbiol 2004;42:3978-84.
- Bou G, Martínez-Beltrán J. Cloning, nucleotide sequencing, and analysis of the gene encoding an AmpC β-lactamase in Acinetobacter baumannii. Antimicrob Agents Chemother 2000;44:428-32.
- Ishii Y, Ohno A, Taguchi H, Imajo S, Ishiguro M, Matsuzawa H. Cloning and sequence of the gene encoding a cefotaxime-hydrolyzing class A beta-lactamase isolated from Escherichia coli. Antimicrob Agents Chemother 1995;39:2269-75.
- Clinical and Laboratory Standards Institute/NCCLS. Performance standards for antimicrobial susceptibility testing; Fifteenth informational supplement. CLSI/NCCLS document M100-S15 2005; 25: 1–167.
- Bonnet R, Dutour C, Sampaio JL, Chanal C, Sirot D, Labia R, et al. Novel cefotaximase (CTX-M-16) with increased catalytic efficiency due to substitution Asp-240→Gly. Antimicrob Agents Chemother 2001;45:2269-75.
- Pechèe JC, Guay R, Dubois J, Letarte R. Hydrolysis of cefotaxime by a beta-lactamase from Bacteroides fragilis. Antimicrob Agents Chemother 1980;17:1001-3.
- Stürenburg E, Kühn A, Mack D, Laufs R. A novel extended-spectrum beta-lactamase CTX-M-23 with a P167T substitution in the active-site omega loop associated with ceftazidime resistance. J Antimicrob Chemother 2004;54:406-9.
- Tenover FC, Arbeit RD, Goering RV, Mickelsen PA, Murray BE, Persing DH, et al. Interpreting chromosomal DNA restriction patterns produced by pulsed-field gel electrophoresis: criteria for bacterial strain typing. J Clin Microbiol 1995;33:2233-9.
- Machado E, Cantón R, Baquero F, Galán JC, Rollán A, Peixe L, et al. Integron content of extended-spectrum-β-lactamase-producing Escherichia coli strains over 12 years in a single hospital in Madrid, Spain. Antimicrob Agents Chemother 2005;49:1823-9.
- Winokur PL, Canton R, Casellas JM, Legakis N. Variations in the prevalence of strains expressing an extended-spectrum beta-lactamase phenotype and characterization of isolates from Europe, the Americas, and the Western Pacific region. Clin Infect Dis 2001;32 Suppl 2:S94-103.
- Bauernfeind A, Grimm H, Schweighart S. A new plasmidic cefotaximase in a clinical isolate of Escherichia coli. Infection 1990;18:294-8.
- Wu PJ, Shannon K, Phillips I. Effect of hyperproduction of TEM-1 beta-lactamase on in vitro susceptibility of Escherichia coli to beta-lactam antibiotics. Antimicrob Agents Chemother 1994;38:494-8.
- Quale JM, Landman D, Bradford PA, Visalli M, Ravishankar J, Flores C, et al. Molecular epidemiology of a citywide outbreak of extended-spectrum beta-lactamase-producing Klebsiella pneumoniae infection. Clin Infect Dis 2002;35:834-41.
- Ma L, Ishii Y, Chang FY, Yamaguchi K, Ho M, Siu LK. CTX-M-14, a plasmid-mediated CTX-M type extended-spectrum beta-lactamase isolated from Escherichia coli. Antimicrob Agents Chemother 2002;46:1985-8.
- Ambler RP, Coulson AF, Frere JM, Ghuysen JM, Joris B, Forsman M, et al. A standard numbering scheme for the class A beta-lactamases. Biochem J 1991;276:269-70.
- Barthélémy M, Péduzzi J, Bernard H, Tancrède C, Labia R. Close amino acid sequence relationship between the new plasmid-mediated extended-spectrum beta-lactamase MEN-1 and chromosomally encoded enzymes of Klebsiella oxytoca. Biochim Biophys Acta 1992;1122:15-22.
- Knox JR. Extended-spectrum and inhibitor-resistant TEM-type beta-lactamases: mutations, specificity, and three-dimensional structure. Antimicrob Agents Chemother 1995;39:2593-601.
- Rahal JJ, Urban C, Horn D, Freeman K, Segal-Maurer S, Maurer J, et al. Class restriction of cephalosporin use to control total cephalosporin resistance in nosocomial Klebsiella. JAMA 1998;280:1233-7.
- Pitout JD, Gregson DB, Church DL, Elsayed S, Laupland KB. Community-wide outbreaks of clonally related CTX-M-14 beta-lactamase-producing Escherichia coli strains in the Calgary health region. J Clin Microbiol 2005;43:2844-9.