Fibrinogen interaction of CHO cells expressing chimeric αIIb/αvβ3 integrin
Introduction
Integrin is a family of cell adhesion molecules, which are heterodimeric transmembrane receptors composed of various α and β subunits[1]. The primary function of integrins is the mechanical connection of cells to the extracellular matrix or to other cells by binding to specific ligands[1,2]. Ligand binding to integrins leads to the generation of intracellular signals, which in concert with other signals, coordinate cell adhesion with cell migration, growth, and differentiation[3,4].
The αvβ3 integrin is found in many cell types and influences cell adhesion and migration with effects on angiogenesis, restenosis, tumor cell invasion, and atherosclerosis. It was shown to be critical in angiogenesis induced by both basic fibroblast growth factor and tumor necrosis factor (TNF)[5–7], and blocking of this integrin prevents angiogenesis in several models. Basic fibroblast growth factor and TNF costimulate αvβ3 expression on developing blood vessels in the chick chorioallantoic membrane and on the rabbit cornea[6,8]. Integrin αIIbβ3 shares the common β3 subunit with αvβ3, is a receptor for fibrinogen, von Willebrand factor (vWF), fibronectin, and vitronectin (VN), and is essential for platelet aggregation[9,10].
Integrins have been implicated in a wide variety of post-receptor occupancy events that occur as a result of ligand binding. These include the activation of cytoplasmic protein tyrosine kinases, increased intracellular pH, and gene induction[3,11]. Additionally, intracellular signaling events can modulate integrin ligand-binding affinity, a process termed “activation” or “inside-out” signaling[4,12]. This has been best characterized in the platelet fibrinogen receptor integrin αIIbβ3[13–15] . A critical feature of the function of αIIbβ3 is that it is modulated by platelet agonists. As a consequence of platelet activation triggered by platelet agonists, such as thrombin, produced from the activated coagulation cascade upon vascular injury, integrin αIIbβ3 rapidly (<1 s) switches from a low-affinity to a high-affinity ligand-binding conformation of its ectodomains through conformational changes initiated by intracellular events referred to as affinity modulation, priming, or inside-out signaling[16–18], which converge on the C-terminal cytoplasmic tails of the integrin subunits. However, the affinity regulation of αvβ3, the VN receptor, is not well understood[19,20].
The integrin α and β cytoplasmic domains plays key roles in integrin signaling[21–24]. It has been reported that the COOH-terminal Arg-Gly-Thr (RGT) sequence of β3 is important for outside-in signaling; the T755NITY759 sequence of β3 containing an NXXY motif is critical to inside-out signaling[25,26]. However, the molecular mechanisms of integrin αvβ3 affinity regulation have been hampered by the lack of a suitable model in cultured cells convenient for adhesion assay. It has been reported that the binding of αvβ3 to VN is regulated[27,28]; however, the measurement of the alternation of the adhesion affinity of the αvβ3 expressing cells to VN has proven to be difficult.
To address the role of the α and β cytoplasmic domains in the affinity regulation of αvβ3, we constructed a series of chimeric αIIb/αv and truncated β3 molecules. The extracellular and transmembrane domains of the αIIb subunit was fused to the cytoplasmic domain of the wild-type αv subunit, and the chimeric gene was stably coexpressed in Chinese hamster ovary (CHO) cells with wild-type β3 and the β3 truncations at sites COOH terminal to T741, Y747, and F754, which have been shown to occur after hydrolysis by calpain[29]. These cells were used to test the cytoplasmic domains of α and β on receptor affinity regulation and post-receptor signal transduction through binding to soluble or immobilized fibrinogen.
Materials and methods
Construction of chimeric α integrin The recombinant αIIb/αv gene, in which the αIIb cytoplasmic sequence has been replaced by the corresponding αv sequence, were prepared as follows: human wild-type αv cDNA was cloned from MDA-MB435 cells (a cell line isolated from the pleural effusion of a patient with breast carcinoma). The αIIb extracellular and transmembrane PCR products were generated under the αIIb cDNA template pcDNA3-IIb (a gift from the Shanghai Institute of Hematology, Ruijin Hospital, Shanghai, China) with primers 5´ GCTCTA-GAAGATTGGCCAGAGC-TTTGTGT 3´ and 5´ CCATCC-TCCACATGGCCAGGACC 3´ and αv cytoplasmic PCR products with primers 5´ GGCCATGTGGAG-GATGGGCTTTTTTAAAC 3´ and 5´ GGGGTACCTCAG-GCACTACCT GTCTTAT 3´. Using αIIb extracellular and transmembrane PCR products and αv cytoplasmic PCR products as templates, and with Ex Taq polymerase (Takara, Tokyo, Japan), dNTP Mix, and Ex Taq buffer in the first 3 rounds of PCR, we obtained PCR products that were then used as templates for PCR with primers 5´ GCTCTAGAA-GATTGGCCAGAGCTTTGTGT 3´ and 5´ GGGGTACCTCA-GGCACTACCTGTCTTAT 3´. The final products were digested with XbaI and KpnI and inserted into pcDNA3.1 zeo (-)digested with the same enzymes, creating a pcDNA3.1 zeo (-)αIIb/Cαv construct.
Plasmids with β3 cDNA wild type and 3 kinds of plasmids with muant β3 cDNA bearing truncations at sites T741, Y747, and F754 of the COOH-terminal, respectively, were kindly provided by the Shanghai Institute of Hematology, Ruijin Hospital. The mutations were confirmed by analysis of the recombinant cDNA in automated DNA sequencing analysis (Invitrogen, America).
Cell culture and transfection The CHO cells were grown in F12 medium supplemented with 10% fetal bovine serum, glutamine, and non-essential amino acid. Transfection was performed using Lipofectamine 2000 (Invitrogen, America). Each mutant β3 cDNA was cotransfected with αIIb/αv at a ratio of 7:1. Forty-eight hours after transfection, the cells were collected and diluted into fresh medium containing Zeocin (Invitrogen, America) at 0.2 mg/mL. The cells were cultured with selective medium every 3 to 4 d until cell foci were clearly visible. The cell colonies were then collected and transferred into 24-well plates. The cells were cultured to subconfluence before expanding to larger plates. Stable cell lines expressing proteins were maintained in the culture in 0.1 mg/mL Zeocin.
Flow cytometric analysis of chimeric integrin expression The expression of chimeric integrins was monitored by flow cytometry using CD41a (BD Pharmingen, San Diego, California, America). The transfected cells were harvested using 0.5 mmol/L EDTA in phosphate-buffered saline (PBS), washed with PBS, resuspended at a density of 1×106 cells/100 µL in modified Tyrode’s solution [2.5 mmol/L N-2-hydroxyethylpiperazine-N-2-ethanesulphonic acid (HEPES), 150 mmol/L NaCl, 2.5 mmol/L KCl, 12 mmol/L NaHCO3, 5.5 mmol/L D-glucose, 1 mmol/L CaCl2, 1 mmol/L MgCl2, and 0.1% bovine serum albumin (BSA), pH 7.4], and incubated for 30 min at 37 °C with monoclonal antibodies specific to the extracellular domain of human αIIbβ3. Next, the cells were washed and exposed to the fluorescein-isothiocyanate (FITC)-F(ab) fragment of rabbit antimouse immunoglobulin G (Santa Cruz, CA, USA) at 37 °C for 30 min, and the intensity of fluorescence was quantified in a Coulter flow cytometer (FACSCalibur, Becton Dickinson, San Jose, CA, USA).
Binding of soluble fibrinogen to transfected CHO cells The transfected CHO cells were resuspended at 1×106 cells/100 µL in modified Tyrode’s solution with 15 µg/mL Alexa Fluor 488-conjugated fibrinogen (Invitrogen, America) for 30 min at room temperature. After washing, the cells were resuspended and analyzed by flow cytometry.
Adhesion of CHO cell lines to immobilized fibrinogen In total, 96-well plates were coated overnight at 4 °C with 25 µg/mL fibrinogen in 0.5 mmol/L NaHCO3 (pH 8.3). The wells then were blocked with 2% BSA-PBS at 37 °C for 2 h. Cell suspension (3×104 cells/well in F12 with 1% BSA) was added to the ligand-coated microtiter wells and incubated for 90 min at 37 °C in a CO2 incubator. After 3 washes, cell spreading was examined under an inverted microscope (40×objective lens). In the quantitative assays, 50 µL of 0.3% p-nitrophenyl phosphate in 1% Triton X-100 and 50 mmol/L sodium acetate (pH 5.0) were added to 96 wells and incubated at 37 °C for 1 h. The reaction was stopped by adding 50 µL of 1 mol/L NaOH. The results were determined by reading the optical density at a 405 nm wavelength.
Antibodies, proteins, and reagents Monoclonal antibodies against the integrin αIIbβ3 complex (CD41a) were purchased from BD Pharmingen (America). Alexa Fluor 488-conjugated human fibrinogen were purchased from Invitrogen (America). The integrin αIIb cDNA clone in pCDNA3 and β3 in the pCDM8 vector were provided by the Shanghai Institute of Hematology, Ruijin Hospital.
Results
Gene expression of αIIb/αv and β3 in CHO cells To study the structure-functional relationship of β3 integrins, we generated 1 wild-type and 3 C-terminal truncated β3 genes and cotransfected these genes with the chimeric αIIb/αv gene in Chinese hamster ovary (CHO) cells. The CHO cells cotransfected with wild-type αIIb and β3 genes were used as controls. Accordingly, 5 CHO cell lines were established.
In order to assess the cell surface expression of recombinant integrins transfected in CHO cells, we used flow cytometry to detect the reactivity of monoclonal antibodies specific to the extracellular domain of the human β3 chain (CD61). As shown in Figure 1, all the mutants used in the present study exhibited similar levels of cell surface expression of the β3 subunit. Since all these cell lines express the αIIbβ3 complex in the outer side of cell surfaces, we then used a flow cytometer to evaluate their expression with the monoclonal antibodies specific to the extracellular complex of human αIIbβ3 (CD41a). As shown in Figure 2, all the cell lines expressed similar levels of the CD41a antigen, indicating similar levels of the αIIbβ3 complex on these cells.
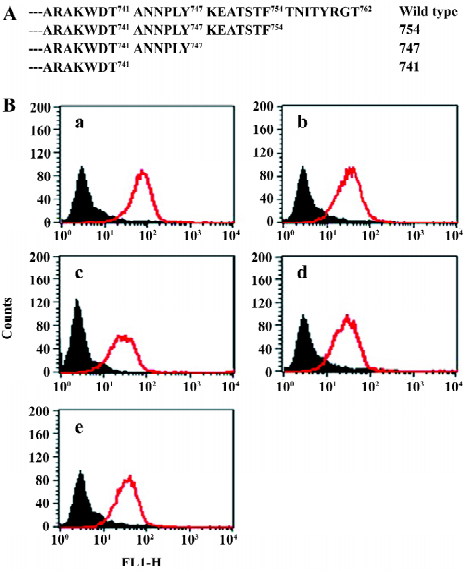
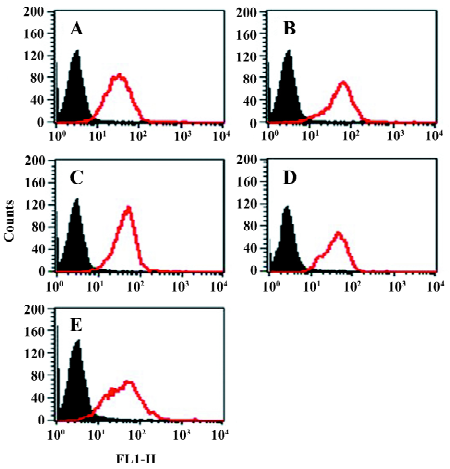
Function of chimeric αIIb/αvβ3 integrins as a fibrinogen receptor It is known that αIIbβ3 is a specific receptor for fibrinogen[9,30,31]. Thus, we first examined fibrinogen binding to the cell surface αIIbβ3 complex after the replacement of the αIIb cytoplasmic domain by the αv cytoplasmic domain. This was assessed by measuring the amount of fluorescence-labeled soluble fibrinogen bound to the resuspended cells without any treatment in a flow cytometer. As shown in Figure 3, the cells expressing αIIb/αvβ3 bound soluble fibrinogen. This binding was inhibited by Arg-Gly-Asp-Ser (RGDS peptide), but the cells expressing wild-type αIIbβ3 could not bind soluble fibrinogen. Thus, unlike natural αIIbβ3 integrin, activation was not required for the cells bearing chimeric genes to bind soluble fibrinogen. This also indicated that the cells bearing the chimeric αIIb/αv gene formed the αIIbβ3 complex in a correct conformation on the cell surfaces, because RGD-dependent fibrinogen binding is a restricted functional marker highly specific for αIIbβ3[32,33]. Then we examined the soluble fibrinogen binding of the cells with the C-terminal truncated β3 at the intracellular compartment. As demonstrated in Figure 4, soluble fibrinogen bound to αIIb/αvβ3/741, β3/747, and β3/754 cells at comparably high levels as to αIIb/αvβ3/762 (WT) cells, showing that αIIb/αvβ3 was in a constant activation state regardless of the truncation of β3 within the intracellular tail.
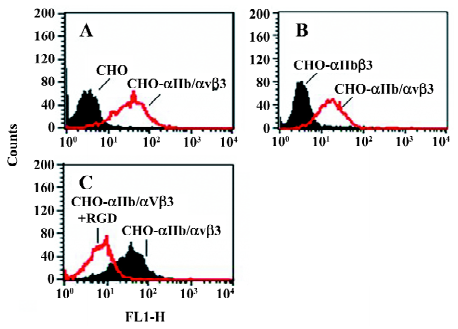
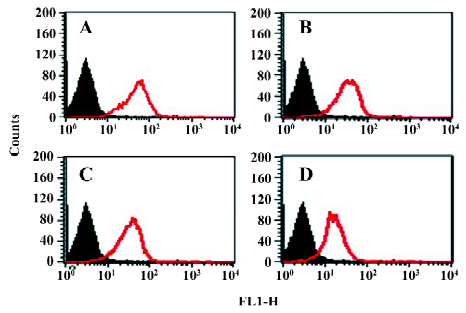
Integrins mediate cell adhesion and spreading on the fibrinogen matrix Another important function of β3 integrins is cell adhesion to the extracellular matrix. The cytoplasmic chain of β3, especially its C-terminal, has been proven to play an important role in integrin signaling and to regulate its function. We further examined the behavior of these cell lines in adhesion and spreading on the fibrinogen matrix.
The adhesive properties of the cells expressing αIIb/αvβ3 containing full-length or truncated β3 subunit proteins were studied. The cell lines β3/741, β3/747, β/754, and β3/762, the cell line αIIbβ3, as well as the non-transfected control cell lines, were added to fibrinogen-coated polystyrene wells. As shown in Figure 5, non-transfected CHO cells lacking αIIb/αvβ3 were incapable of adhering to the fibrinogen matrix. CHO cells expressing αIIbβ3 and the cells expressing αIIb/αvβ3/762 (WT) adhered to and spread well on the fibrinogen matrix. In contrast, all CHO cells displaying truncated β3 in the αIIb/αvβ3 complex, including β3/741, β3/747, and β3/754, failed to adhere to the fibrinogen matrix. These results showed that the full length of β3 was required for the cell adhesion to immobilize fibrinogen; any deletion of the C-terminal sequence of β3 would abolish αIIb/αvβ3-mediated adhesion and spreading.
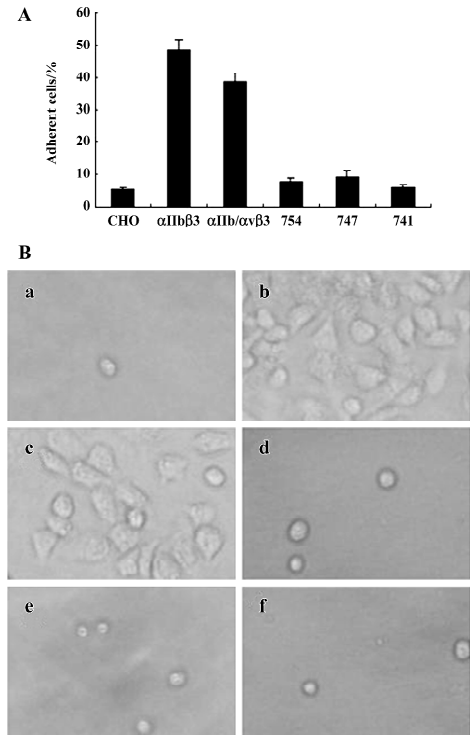
Discussion
In this study, we generated the chimeric αIIb/αvβ3 integrin protein, which fused the extracellular and transmembrane sequences of αIIb to the intracellular sequences of αv and successfully expressed this chimeric integrin on the surface of CHO cells. This model was designed to test whether such a chimeric molecule is functionally active and if any signal transduction can be observed by the ligand binding assay and cell adhesion assay. Since αIIbβ3 binding to fibrinogen is extremely well documented and easy to test, this chimeric integrin was expected to provide a useful model for the study of signal transduction mediated by the αvβ3 intracellular domains.
The activation of αIIbβ3 has been well documented[12–14]. The natural αIIbβ3 on the platelets was inactive and turns into an active form once the cells are stimulated by agonists, such as thrombin, through inside-out signaling[30,34,35]. Our results are in agreement with this notion since fibrinogen did not bind to the CHO cells expressing αIIbβ3, while these cells expressed high levels of the β3 chain (CD61) and αIIbβ3 complex (CD41a). There are also some reports about the constitutive activation of αIIbβ3 by demonstrating that the chimeric α subunits with the extracellular and transmembrane of αIIb joined to the cytoplasmic domains of α5, α6A, or α6B conferred a high affinity state to the recombinant integrins[36]. In the present study, we showed that once the αIIb cytoplasmic tail was substituted by the αv tail, the extracellular αIIbβ3 receptors were constitutively active for their ligands in the absence of the inside-out signals. The activated state of the receptors remained unimpaired with the truncations of the cytoplasmic sequences of β3, as we could see the significant amount of the soluble fibrinogen bound to the cells expressing these truncated versions of integrin β3. This binding was specifically mediated by αIIb/αvβ3 because it was completely inhibited by the RGD peptide. Based upon our data and those of others, we propose a model for integrin activation, in which the αIIb and β3 chains within extracellular domains tend to expose fibrinogen-binding sites. This tendency is however blocked by the interaction of the intracellular part of the αIIb and β3 subunits. Accordingly, any structural alternation that impairs the interaction of the αIIb and β3 subunits within the intracellular domains will activate the integrin. We believe that the proposal of this model would be beneficial in understanding the mechanisms of integrin activation which is thought to be regulated by inside-out signaling[12–14]. It is tempting to speculate that the disturbance of the interaction between the intracellular and transmembrane domains of the αIIb and β3 subunits, in the case of the chimeric α subunit as in this study or of talin interaction, causes a “deblockade” of the suppressed receptor activity and this will be responsible for integrin activation[37,38].
We showed that both the cells expressing αIIbβ3 and the cells expressing chimeric αIIb/αvβ3 molecules adhered to and spread well on immobilized fibrinogen in a similar manner. In contrast, the 3 cell lines expressing truncated β3 at the C-terminal failed to adhere firmly and spread. Our data showed that the truncations of β3 did not alter the soluble fibrinogen binding capacity of αIIb/αvβ3, but it did alter the cell adhesion on immobilized fibrinogen, indicating that fibrinogen binding capacity is not sufficient to support fibrinogen-mediated cell adhesion and spreading. In fact, it showed that the cell spreading on immobilized fibrinogen was a more complicated phenomenon and depended on the integrity of intracellular β3, which has been reported to play a key role in signal transduction[4,25,26]. It is known that binding of ligands to αIIbβ3 not only forms adhesive bonds between platelets, but also transmits outside-in signals to induce a series of cellular responses, such as protein phosphorylation[39,40], elevation of intracellular Ca2+ [41], and cytoskeleton reorganization[42], leading to cell spreading and the stabilization of cell adhesion[43–45]. In particular, the hydrolysis of short peptides at the C-terminal of the β3 chain has been shown to be involved in this signal transmission[25,29]. Therefore, the failure of the cells with truncated β3 to adhere to and spread on immobilized fibrinogen could be due to the disruption of the outside-in signals that occurred in natural αIIbβ3. This interpretation underlines once again the importance of the regulatory role of β3 within the intracellular αvβ3 complex in outside-in signal transmission. However, further study is needed to elucidate the detailed mechanisms.
Our data indicate that the intracellular interactions within the αvβ3 cytoplasmic tail of the cell line CHO αIIb/αvβ3 regulates the adhesion function of the extracellular αIIbβ3 domain to immobilize fibrinogen and consequent spreading. Therefore, the cell line CHO αIIb/αvβ3 will be a useful model for studying the intracellular protein-protein interaction in which αvβ3 intracellular domains are involved. Furthermore, this model is potentially useful for the drug screening of active substances interfering in αvβ3-mediated signaling. In addition, as we showed, the chimeric integrin αIIb/αvβ3 was constitutively activated for ligand binding with no need of agonist stimulation, which suggests that the cell model would be also a useful tool in drug screening of new substances interfering with fibrinogen binding to αIIbβ3, a critical step during platelet aggregation and blood clot formation.
References
- Albelda SM, Buck CA. Integrins and other cell adhesion molecules. FASEB J 1990;4:2868-80.
- Hynes RO. Integrins: versatility, modulation and signaling in cell adhesion. Cell 1992;69:11-25.
- Schwartz MA, Schaller MD, Ginsberg MH. Integrins: emerging paradigms of signal transduction. Annu Rev Cell Dev Biol 1995;11:549-99.
- Hynes RO. Integrins: bidirectional, allosteric signaling machines. Cell 2002;110:673-87.
- Brooks PC, Clark RAF, Cheresh DA. Requirement of vascular integrin αvβ3 for angiogenesis. Science 1994;264:569-71.
- Sepp NT, Li LJ, Lee KH, Brown EJ, Caughman SWW, Lawley TJ, et al. Basic fibroblast growth factor increases expression of the αvβ3 complex on human microvessel endothelial cells. J Invest Dermato 1994;103:295-9.
- Varner JA, Cheresh DA. Integrins and cancer. Curr Opin Cell Biol 1996;8:724-30.
- Friedlander M, Brooks PC, Shaffer RW, Kincaid CM, Varner JA, Cheresh DA. Definition of two angiogenic pathways by distinct αv integrins. Science 1995;27:1500-2.
- Quinn MJ, Byzova TV, Qin J, Topol EJ, Plow EF. Integrin alphaIIbbeta3 and its antagonism. Arterioscler Thromb Vasc Biol 2003;23:945-52.
- Fullard JF. The role of the platelet glycoprotein IIb/IIIa in thrombosis and haemostasis. Curr Pharm Des 2004;10:1567-76.
- Juliano R. Signal transduction by integrins and its role in the regulation of tumor growth. Cancer Metastasis Rev 1994;13:25-30.
- Calderwood DA. Integrin activation. J Cell Sci 2004;117:657-66.
- Vinogradova O, Velyvis A, Velyviene A, Hu B, Haas T, Plow E, et al. A structural mechanism of integrin αIIbβ3 “inside-out” activation as regulated by its cytoplasmic face. Cell 2002;110:587-97.
- Leisnera TM, Yuana WP, DeNofriob JC, Liua J, Parisea LV. Tickling the tails: cytoplasmic domain proteins that regulate integrin αIIbβ3 activation. Curr Opin Hematol 2007;14:255-61.
- Wegener KL, Partridge AW, Han J, Pickford AR, Liddington RC, Ginsberg MH, et al. Structural basis of integrin activation by talin. Cell 2007;128:171-82.
- Miranti CK, Brugge JS. Sensing the environment: a historical perspective on integrin signal transduction. Nat Cell Biol 2002;4:83-90.
- Shimaoka M, Takagi J, Springer TA. Conformational regulation of integrin structure and function. Annu Rev Biophys Biomol Struct 2002;31:485-16.
- Liddington RC, Ginsberg MH. Integrin activation takes shape. J Cell Biol 2002;158:833-39.
- Xiong JP, Stehle T, Diefenbach B, Zhang R, Dunker R, Scott DL, et al. Crystal structure of the extracellular segment of integrin αvβ3. Science 2001;294:339-45.
- Ahrens IG, Moran N, Aylward K, Meade G, Moser M, Assefa D, et al. Evidence for a differential functional regulation of the two β3-integrins αvβ3 and αIIbβ3. Exp Cell Res 2006;312:925-37.
- O’Toole TE, Katagiri Y, Faull RJ, Peter K, Tamura R, Quaranta V, et al. Integrin cytoplasmic domains mediate inside-out signal transduction. J Cell Biol 1994;124:1047-59.
- Vinogradova O, Haas T, Plow EF, Qin J. A structural basis for integrin activation by the cytoplasmic tail of the alpha IIb-subunit. Proc Natl Acad Sci USA 2000;97:1450-55.
- Aylward K, Meade G, Ahrens I, Devocelle M, Moran N. A novel functional role for the highly conserved α-subunit KVGFFKR motif distinct from integrin αIIbβ3 activation processes. J Thromb Haemost 2006;4:1804-12.
- Ginsberg MH, Yaspan B, Forsyth J, Ulmer TS, Campbell ID, Slepak M. A membrane-distal segment of the integrin alpha IIb cytoplasmic domain regulates integrin activation. J Biol Chem 2001; 276: 22 514–21.
- Xi XD, Bodnar RJ, Li ZY, Lam SCT, Du XP. Critical roles for the COOH-terminal NITY and RGT sequences of the integrin β3 cytoplasmic domain in inside-out and outside-in signaling. J Cell Biol 2003;162:329-39.
- Liu J, Jackson CW, Gruppo RA, Jennings LK, Gartner TK. The beta3 subunit of the integrin alphaIIbbeta3 regulates alphaIIb-mediated outside-in signaling. Blood 2005;105:4345-52.
- Yamanouchi J, Hato T, Tamura T, Fujita S. Suppression of integrin activation by the membrane-distal sequence of the integrin αIIb cytoplasmic tail. Biochem J 2004;379:317-23.
- Boettiger D, Huber F, Lynch L, Blystone S. Activation of αvβ3–vitronectin binding is a multistage process in which increases in bond strength are dependent on Y747 and Y759 in the cytoplasmic domain of β3. Mol Biol Cell 2001;12:1227-37.
- Du X, Saido TC, Tsubuki S, Indig FE, Williams MJ, Ginsberg MH. Calpain cleavage of the cytoplasmic domain of the integrin β3 subunit. J Biol Chem 1995; 270: 26 146–51.
- Marguerie GA, Plow EF, Edgington TS. Human platelets possess an inducible and saturable receptor specific for fibrinogen. J Biol Chem 1979;254:5357-63.
- Savage B, Saldivar E, Ruggeri ZM. Initiation of platelet adhesion by arrest onto fibrinogen or translocation on von Willebrand factor. Cell 1996;84:289-97.
- Pytela R, Pierschbacher MD, Ginsberg MH, Plow EF, Ruoslahti E. Platelet membrane glycoprotein IIb/IIIa: member of a family of Arg-Gly-Asp-specific adhesion receptors. Science 1986;231:1559-62.
- Ruoslahti E, Pierschbacher MD. New perspectives in cell adhesion: RGD and integrins. Science 1987;238:491-7.
- Chung J, Gao AG, Frazier WA. Thrombospondin acts via integrin-associated protein to activate the platelet integrin alphaIIbbeta3. J Biol Chem 1997; 272: 14 740–6.
- Chen YP, Djaffar I, Pidard D, Steiner B, Cieutat AM, Caen JP, . Platelet signal transduction pathways: could we organize them into a ‘hierarchy’? Proc Natl Acad Sci USA 1992; 89: 10 169–73.
- O’Toole TE, Ylanne J, Culley BM. Regulation of integrin affinity states through an NPXY motif in the β subunit cytoplasmic domain. J Biol Chem 1995;270:8553-8.
- Kim M, Carman CV, Springer TA. Bidirectional transmembrane signaling by cytoplasmic domain separation in integrins. Science 2003;301:1720-5.
- Cram EJ, Schwarzbauer JE. The talin wags the dog: new insights into integrin activation. Trends Cell Biol 2004;14:55-7.
- Golden A, Brugge JS, Shattil SJ. Role of platelet membrane glycoprotein IIb–IIIa in agonist-induced tyrosine phosphorylation of platelet proteins. J Cell Biol 1990;111:3117-27.
- Ling K, Doughman RL, Iyer VV, Firestone AJ, Bairstow SF, Mosher DF. Tyrosine phosphorylation of type I gamma phospha-tidylinositol phosphate kinase by Src regulates an integrin-talin switch. J Cell Biol 2003;163:1339-49.
- Pelletier AJ, Bodary SC, Levinson AD. Signal transduction by the platelet integrin αIIbβ3: induction of calcium oscillations required for protein tyrosine phosphorylation and ligand-induced spreading of stably transfected cells. Mol Biol Cell 1992;3:989-98.
- Jenkins AL, Nannizzi-Alaimo L, Silver D, Sellers JR, Ginsberg MH, Law DA. Tyrosine phosphorylation of the beta3 cytoplasmic domain mediates integrin-cytoskeletal interactions. J Biol Chem 1998;273:13878-85.
- Parise LV. Integrin αIIbβ3 signaling in platelet adhesion and aggregation. Curr Opin Cell Biol 1999;11:597-1.
- Fox JE, Lipfert L, Clark EA, Reynolds CC, Austin CD, Brugge JS. On the role of the platelet membrane skeleton in mediating signal transduction. Association of GPIIb-IIIa, pp60c-src, pp62c-yes, and the p21ras GTPase-activating protein with the membrane skeleton. J Biol Chem 1993; 268: 25 973–84.
- Wonerow P, Pearce AC, Vaux DJ, Watson SP. A critical role for phospholipase Cgamma2 in alphaIIbbeta3-mediated platelet spreading. J Biol Chem 2003;278:37520-9.