Stereoselectivity of satropane, a novel tropane analog, on iris muscarinic receptor activation and intraocular hypotension1
Introduction
Muscarinic receptors initiate many important physiological actions of acetylcholine, a major neurotransmitter in the nervous system. Molecular cloning and pharmacological studies have revealed 5 distinct muscarinic receptors referred to as M1–M5[1]. Central muscarinic receptors are involved in cognitive, behavioral, sensory, motor, and autonomic functions. The peripheral actions mediated by muscarinic receptors include a reduction of heart rate, stimulation of glandular secretion, and smooth muscle contraction[2]. Reduced or increased signaling through muscarinic receptors has been implicated in the pathophysiology of several major diseases[3]. Chemical compounds that influence central and peripheral muscarinic function have long been the focus of intensive research, since the development of new highly potent muscarinic receptor agonists could provide novel therapeutic agents useful, for example, in the treatment of glaucoma, pain, and Alzheimer’s disease.
The cholinergics were the first class of agents used for the treatment of glaucoma, a disease characterized by the degeneration of optic nerve axons and death of retinal ganglion cells, and frequently associated with high intraocular pressure[4]. It was considered that muscarinic cholinergics improved the trabecular outflow of aqueous fluid by opening trabecular meshwork action. However, it now appears that they also limit the production of aqueous humor and have protective and trophic effects on retinal ganglion cells[5–7]. As a consequence, there has recently been renewed interest in the application of muscarinic-based therapies in the treatment of glaucoma[8].
Baogongteng A (6β-acetoxy-2β-hydroxy-nortropane), a nortropane alkaloid from the Chinese herb Erycibe obtusifolia Benth, first isolated by our laboratory[9,10], has been demonstrated to posses potent agonistic activity on muscarinic receptors and was developed into an antiglaucoma agent in China. Clinical trials demonstrated that the therapeutic efficacy was similar to pilocarpine in the treatment of primary glaucoma[11–13]. However, the low amount of baogongteng A available from the herb limits its extensive clinical application. Thus, great efforts has been taken in the synthesis of baogongteng A and its analogs by our laboratory and others in recent decades[14–19]. Satropane (racemic 3α-paramethyl-benzenesulfonyloxy-6β-acetoxy-tropane), a novel tropane analog synthesized in our laboratory, was shown to be a promising candidate as a new antiglaucoma agent in our previous preclinical studies.
Data that the agonist (and the antagonist) binding sites of muscarinic receptors are asymmetrical, and hence generally capable of distinguishing between optical isomers of chiral ligands have been accumulated[20–22]. It is reasonable to suppose that enantiomers of satropane may behave as different compounds on interaction with muscarinic receptors, and the stereospecific interactions of racemic satropane at recognition sites in muscarinic receptors may result in differences in both biological and toxicological effects. Recently, we resolved satropane into a pair of enantiomers, S(–)satropane (lesatropane) and R(+)satropane (see Figure 1 for structural formula)[15].
In order to study the stereoselectivity of satropane on iris muscarinic receptor activation and intraocular hypo-tension, we investigated the pharmacological characteristics of these 2 chiral compounds by comparing their effects on muscarinic receptors in rabbit eyes in vitro and in vivo. The binding characteristics, contractile responses of isolated rabbit iris muscle, miotic response of the conscious rabbit, and intraocular hypotension of the enantiomers of satropane were investigated. The results revealed that the agonistic and hypotensive properties of satropane on rabbit eyes were stereoselective, with the S(–)isomer being its active form.
Materials and methods
Drugs R,S(±)satropane and its enantiomers were synthesized in our department, as previously described[15]. The enantiomeric excess of S(–)satropane and R(+)satropane was 98.05% and 100.00%, respectively, analyzed on a chiral HPLC column (Chiralpack AD). Carbachol, pilocarpine, atropine, pirenzepine, gallamine, 4-diphenylacetoxy-N-methylpiperi-dine (4-DAMP), and tris-(hydroxymethyl) amino methane (Tris) were obtained from Sigma (St Louis, MO, USA), and [3H]quinuclydinyl benzilate ([3H]-QNB; spec. act. 43 Ci/mmol) was from Amersham (Buckinghamshire, England).
Animal and tissue preparation New Zealand rabbits (2.5±0.5 kg, Certificate N
Radioligand-receptor binding assay The iris muscle was minced with scissors in ice-cold 50 mmol/L Tris buffer (pH 7.4). The tissue was then homogenized in 1g of 20 mL (w/v) volume ice-cold 0.32 mol/L sucrose in Tris buffer using a Waring blender (IKA, Staufen, Germany) and further disrupted with an Ultraturrax tissuemizer (IKA, Staufen, Germany). The crude homogenate was centrifuged for 10 min at 1000×g and the resulting supernatant was centrifuged for 60 min at 20 000×g. The pellet was resuspended in Tris buffer as a crude membrane fraction. All the procedures were performed at 4 °C. In the saturation binding assay, the membranes (0.1 mg protein) were incubated vibrantly at 32 °C for 30 min with 0.05–1.1 nmol/L [3H]QNB in a total volume of 0.4 mL. The reaction was terminated by rapid filtration through GF/C glass fiber filters and washed 3 times with ice-cold Tris buffer. The protein concentration was determined with the micro BCA kit (Pierce, Rockford, IL, USA), using bovine serum albumin as the standard. For the competition binding assays, iris muscle membranes (0.1 mg protein) were incubated with 0.4 nmol/L [3H]-QNB at 32 °C for 60 min with increasing concentrations of the agonists carbachol, pilocarpine, S(–)satropane, and R(+)satropane, respectively, in a total volume of 0.4 mL. All the dilutions for the agonists were made in Tris buffer. Non-specific binding was measured in the presence of 10 µmol/L atropine sulfate and accounted for 5%–12% of the total binding. Assays were performed in duplicate.
Isolated iris contraction assay The freshly prepared iris muscle was mounted in 10 mL organ chambers containing modified Krebs-Henseleit solution containing (in mmol/L): 118 NaCl, 4.7 KCl, 2.5 CaCl2, 25 NaHCO3, 1.2 MgSO4, 1.2 KH2PO4, 11.0 glucose, and 0.5 EDTA·Na2. The bath was continuously aerated with aO2:CO2 mixture (95%:5%) and kept at a constant temperature of 37 °C. The preparation was connected vertically to a force-displacement transducer under a resting tension of 500 mg. Preparations were allowed to equilibrate for at least 60 min before the drug addition, during which the buffer solution was refreshed every 15 min. Isometric contractions were recorded using a PowerLab/8sp life analysis system (AD-Instruments, Australia). In order to confirm the viability of the tissue, preparation was exposed to a high potassium concentration (60 mmol/L KCl) following the stabilization period. After washout replacement with normal medium and return to the original baseline, the cumulative concentration–response curves were obtained for carbachol, pilocarpine, R,S(±)-satropane, S(–)satropane, and R(+)satropane, respectively. The concentration was increased as soon as a stable response to the previous concentration appeared. The contractile responses of the iris muscle to each dose of the muscarinic agonists are expressed as percentages of that elicited by 100 μmol/L carbachol. In our pilot study, carbachol at this concentration could induce the maximum contraction. No significant desensitization was observed for at least 2 consecutive concentration–response curves for the compounds. Accordingly, no more than 2 complete curves were recorded for each tissue. In order to investigate whether the active isomer of satropane produced iris contraction through the muscarinic receptor and to determine the subtype of the receptor mediating the effects, muscarinic receptor selective antagonist atropine and muscarinic receptor subtype selective antagonists piren-zepine (for M1), gallamine (for M2), and 4-DAMP (for M3) were used[23,24]. The antagonists were added to the preparations 20 min before the administration of the agonists. Different sets of preparations (1, 10, and 100 μmol/L) of the inactive isomer of satropane were pre-incubated to determine if the isomer without agonistic effect elicits an antagonist profile. All concentrations of drugs are expressed as the final concentration in the organ bath.
Pupil diameter and induced ocular hypertension measurements The compounds were dissolved in physiological saline (9 g/L sodium chloride solution) and each eye was instilled 100 µL eye-drop solution. In each following test, 6 different dosing studies (20 eyes of 10 rabbits per group) were evaluated in random order and included physiological saline, pilocarpine (2%), S(–)satropane (0.015%, 0.03%, and 0.06%) and R(+)satropane (0.12%; w/v).
For measuring the pupil diameter, conscious rabbits were placed in restraint boxes to which they had been habituated, with unrestricted head or eye movements. The pupil diameter (in mm) was measured with a Castroviejo caliper under normal room lighting and readings were taken before (taken as 0 min) and at 15, 30, 60, 120, 180, and 240 min after the application of compounds.
Water loading-induced ocular hypertension and methylcellulose-induced ocular hypertension were assessed as described by Konno et al with slight modifications[25–27]. Briefly, the orogastric administration of 100 mL/kg (37 °C) of distilled water into rabbits was for acute ocular hypertension. For inducing methylcellulose-induced ocular hypertension, a single injection of 2% methylcellulose (in sterile saline solution) with a 30 gauge needle was introduced into the posterior ocular chamber of the eyes of the rabbits anesthetized by an intravenous injection of 30 mg/kg sodium pentobarbital and a topical administration of 1% tetracaine.
The intraocular pressure of both eyes was measured using Schiotz tonometers (Suzhou Medical Instruments, Suzhou, China) immediately before (taken as 0 min or h) and at 15, 30, 60, 120, and 180 min after the administration of distilled water in water loading-induced ocular hypertension test or at 0.5, 2, 4, 6, and 24 h after the injection of methylcellulose in the methylcellulose-induced hypertension test. The compounds tested were instilled immediately after the administration of distilled water or methylcellulose.
Statistics and data analysis In the binding tests, non-linear curve fitting by GraphPad PRISM 4.0 (San Diego, CA, USA) was used to generate affinity (Kd) and capacity (Bmax) values for [3H]-QNB and the competition parameters. The apparent dissociation constants (Ki) were calculated from IC50 values according to the Cheng-Prusoff equation, and the values were expressed as pKi (–lg Ki). The only variables constrained in the analysis were those that were experimentally determined, namely, the dissociation constant for [3H]-QNB and the non-specific binding of [3H]-QNB. For the iris contraction assay, EC50 (concentration of an agonist produce 50% of the maximal response of the agonist) values were calculated by means of non-linear curve fitting of sigmoidal dose-response logistic transformation using PRISM 4.0. The negative logarithm to base 10 of the equilibrium dissociation constant pKb values for the antagonists were determined by Schild analysis. Data were expressed as the mean±SD of 3 independent experiments unless otherwise stated.
The statistically significant differences were determined by Student’s t-test or by ANOVA, as appropriate. Differences were considered statistically significant if P<0.05.
Results
Enantiomers of satropane binding to iris muscle The binding of [3H]-QNB was saturable. The dissociation equilibrium constant (Kd) and receptor density (Bmax) were determined to be 0.22±0.09 nmol/L and 1.25±0.08 pmol·mg–1 protein (n=3), respectively.
Displacement of [3H]-QNB binding was performed using carbachol, pilocarpine, S(–)satropane and R(+)satropane. [3H]-QNB binding on the muscarinic receptor was inhibited by the compounds in a concentration-dependent manner (Figure 2). The maximum inhibition and the pKi values of the compounds against [3H]-QNB binding are summarized in Table 1. With the exception of R(+)satropane, all the compounds were completely against the labeled ligand binding with muscarinic receptors in the iris muscle.
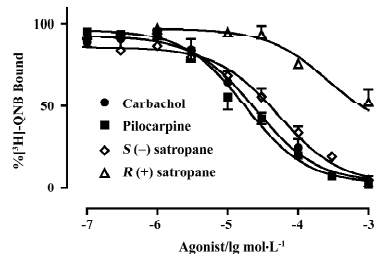
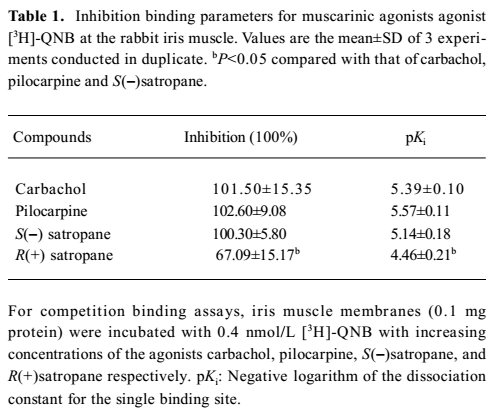
Full table
Enantiomers of satropane on the contraction of isolated rabbit iris muscle The cumulative addition of carbachol, pilocarpine, and S(–)satropane to the isolated iris muscle produced a concentration-dependent contractile response (Figure 3). However, R(+)satropane did not induce any contractile response up to the concentration of 300 μmol/L. The parameters of the dose–response are shown in Table 2. Carbachol and S(–)satropane were the most potent, and pilocarpine was least potent with approximate one-ninth potency of carbachol and S(–)satropane. The efficacy of carbachol, pilocarpine, and S(–)satropane varied. Carbachol was most efficacious, inducing the maximum contraction of the iris muscle (0.41±0.11 g). Pilocarpine was least efficacious, eliciting less than 23% of the maximal response to carbachol (P<0.01). S(–)satro-pane stimulated the contraction of the iris muscle, with the maximum contraction near that of carbachol (P>0.05) and greater than that of pilocarpine (P<0.01).
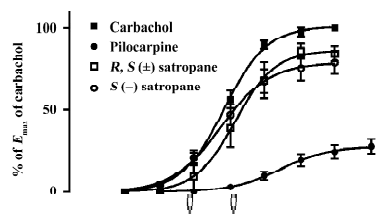
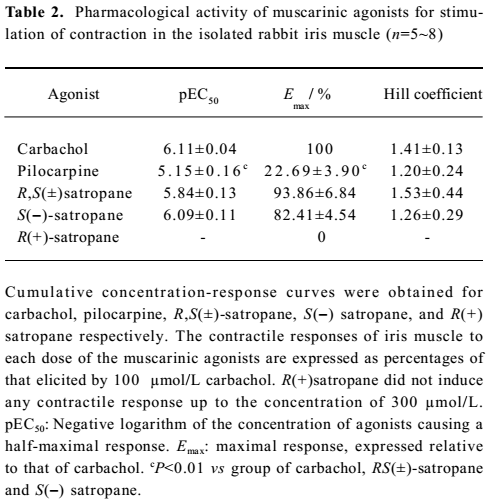
Full table
Effect of S(–)satropane on the contraction of the isolated rabbit iris muscle under the pre-incubation of the muscarinic receptor antagonists or R(+)satropane Pre-incubation of the preparations with the various concentrations of muscarinic receptor selective antagonist atropine or the M3 subtype selective antagonist 4-DAMP made the dose-response curves of S(–)satropane shift rightward in a parallel manner. The pKb values of atropine and 4-DAMP were 9.12±0.09 and 9.10±0.08, respectively. The M1 subtype selective antagonist pirenzepine up to 100 nmol/L and M2 subtype selective antagonist gallamine up to 1 μmol/L failed to shift the S(–)satropane concentration–response curve (Figure 4). Pre-incubation with R(+)satropane up to 100 μmol/L had no effect on the iris contraction induced by carbachol (data not shown).
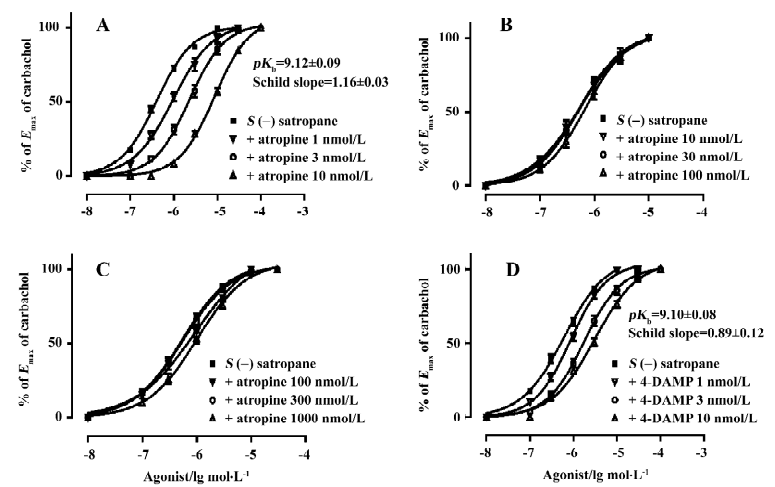
Effect of enantiomers of satropane on conscious rabbit pupil diameter The basal pupil size was 6.01±0.03 mm before drug treatment. 0.12% R(+)satropane failed to induce miosis. Pilocarpine (2%) and S(–)satropane (0.015%, 0.03%, and 0.06%) significantly decreased the pupil diameter after the topical administration until 120 min, with the maximal effect at 15 or 30 min (Figure 5). At 15 min, pilocarpine decreased the pupil diameter to 4.21±0.16 mm, and at 30 min, it decreased to 4.32±0.09 mm. The significance of miosis produced by S(–)satropane was concentration dependent, with 0.06% S(–)satropane eliciting the maximum effect. The pupil diameter was lowered to 3.51±0.16 mm at 30 min after instillation with 0.06% S(–)satropane compared to 6.12±0.15 mm after instillation with physiological saline. S(–)satropane was more potent in inducing miosis than pilocarpine.
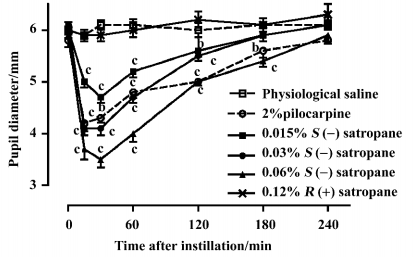
Effect of enantiomers of satropane on water loading-induced and methylcellulose-induced ocular hypertension The basal intraocular pressure was 2.65±0.08 kPa (n=20) before drug treatment. Water loading caused a rapid increase in intraocular pressure to 4.70±0.17 kPa at 15 min, 4.54±0.15 kPa at 30 min, and decreased near to the baseline at 180 min. Eye dropping of pilocarpine (2%) and S(–)satropane (0.03% and 0.06%) significantly suppressed water loading-induced ocular hypertension from 15 min after the administration of water until 120 min. S(–)satropane was more potent in reducing intraocular pressure than pilocarpine (Figure 6A).
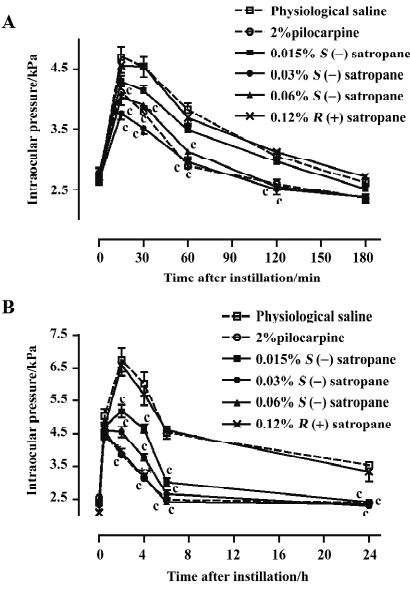
The injection of methylcellulose into the posterior chamber of the rabbit eye produced an elevation in intraocular pressure, which reached its maximum of 6.75±0.36 kPa 2 h after the injection and decreased until it was stabilized at a level of approximately 3.53–4.54 kPa for 20 h (Figure 6B). Pilocarpine (2%) and S(–)satropane (0.015%, 0.03%, and 0.06%) significantly suppressed methylcellulose-induced ocular hypertension throughout the whole duration tested. S(–)satropane was more potent in reducing intraocular pressure than pilocarpine. R(+)satropane neither suppressed water loading-induced nor methylcellulose-induced ocular hypertension.
Discussion
Muscarinic receptors play key roles in the central and peripheral nervous system. Molecular cloning and pharmacological studies have revealed 5 distinct muscarinic receptors referred to as M1–M5[24]. The iris-ciliary body contains parasympathetic innervation and contributes to the regulation of intraocular pressure and pupil diameter[28]. The main effects on iris contractility and outflow facilitation are mediated by muscarinic stimulation[29,30]. A number of techniques have revealed that it is the M3 subtype that appears to be the most abundant muscarinic receptor expressed in the iris of humans and other mammals. The contraction of the iris by muscarinic agonists is also primary mediated by the M3 receptor[31–34].
In our study, the enantiomers of satropane inhibited [3H]-QNB binding on the muscarinic receptor in a concentration-dependent manner. S(–)satropane completely competed against the labeled ligand as carbachol and pilocarpine did, whereas R(+)satropane did not. It is likely that R(+)satropane has very weak binding affinity with muscarinic receptors in the iris muscle. R(+)satropane did not induce miosis or suppress hypertensive intraocular pressure induced by water loading or by methylcellulose posterior ocular chamber injection, but S(–)satropane induced these effects at a much lower concentration than pilocarpine. Moreover, in the isolated iris assay, R,S(±)satropane and S(–)satropane produced a potent contractile response, while R(+)satropane did not. Pre-incubation with R(+)satropane had no effect on the iris contraction induced by carbachol, indicating that R(+)satropane did not behave like an antagonist. The potency and the efficacy of S(–)satropane was similar to carbachol. In this way, S(–)satropane behaved like a fully or highly efficacious partial agonist, whereas R(+)satropane elicited neither agonistic nor antagonistic activity in inducing the contraction of the iris muscle both in vitro and in vivo. The contractile responses of S(–)satropane on the isolated iris muscle were blocked by muscarinic receptor antagonist atropine and M3 subtype selective antagonist 4-DAMP, but hardly by M1 subtype selective antagonist pirenzepine and M2 subtype selective allosteric antagonist gallamine. The effects of S(–)satropane is mediated by a M3-like receptor subtype in the iris muscle.
Besides lesatropane, many other 6β-acetoxy(nor)tropane analogs have been demonstrated to elicit potent agonist activity at muscarinic receptors[18,35–38]. However, their tropane counterparts, such as atropine, scopolamine, anisodine, and anisodamine, are generally known potent muscarinic receptor antagonists. The tropane alkaloids appear as useful tools to study the physiological roles and provide an interesting starting point for the analysis of structure–activity relationships at muscarinic receptors. The significance of molecular chirality is widely recognized in life sciences[39–41]. Although the use of chiral drugs predates modern medicine, it is only since the 1980s has there been a significant increase in the development of chiral pharmaceutical drugs, primarily due to the recognition that enantiomers often have different bioactivity and metabolic fates. Additional isomers in a compound are no longer considered “silent passengers”, but potential contaminants (so-called isomeric ballast)[42]. The enantiomers of satropane behave as different compounds on interaction with muscarinic receptors, and the stereospecific interactions of racemic satropane at recognition sites in muscarinic receptors may result in differences in both biological and toxicological effects. In the present study, S(–)satropane rather than R(+)satropane elicited agonistic activity on muscarinic receptors and suppressed hypertensive intraocular pressure. It is possible that R(+)satropane without pharmacodynamic effects could behave as a potential contaminant with the administration of racemic satropane to patients. The chemical and pharmacodynamic separation of the opposite configurations of satropane is likely to assist in further research and discovery of this kind of muscarinic receptor agonist. The exploration and development of single isomer drugs may bring significant advances in treatment options.
In conclusion, satropane exhibits significant agonistic effect on the iris muscarinic receptor, and the agonistic and hypotensive properties of satropane on rabbit eyes are stereoselective with the S(–)isomer lesatropane being its active form.
References
- Brown DA. Acetylcholine. Br J Pharmacol 2006;147 Suppl 1:S120-6.
- Eglen RM, Choppin A, Dillon MP, Hegde S. Muscarinic receptor ligands and their therapeutic potential. Curr Opin Chem Biol 1999;3:426-32.
- Wess J. Muscarinic acetylcholine receptor knockout mice: novel phenotypes and clinical implications. Annu Rev Pharmacol Toxicol 2004;44:423-50.
- Schwartz M. Neurodegeneration and neuroprotection in glaucoma: development of a therapeutic neuroprotective vaccine: the Friedenwald lecture. Invest Ophthalmol Vis Sci 2003;44:1407-11.
- Ellis DZ, Nathanson JA, Rabe J, Sweadner KJ. Carbachol and nitric oxide inhibition of Na,K-ATPase activity in bovine ciliary processes. Invest Ophthalmol Vis Sci 2001;42:2625-31.
- Miki A, Otori Y, Morimoto T, Okada M, Tano Y. Protective effect of donepezil on retinal ganglion cells in vitro and in vivo. Curr Eye Res 2006;31:69-77.
- Pereira SP, Medina SV, Araujo EG. Cholinergic activity modulates the survival of retinal ganglion cells in culture: the role of M1 muscarinic receptors. Int J Dev Neurosci 2001;19:559-67.
- Duncan G, Collison DJ. Role of the non-neuronal cholinergic system in the eye: a review. Life Sci 2003;72:2013-9.
- Yao TR, Chen ZN. Chemical studies on Erycibe Obtusifolia. Bao Gong Teng. Isolation and preliminary study on a new myotic constitutuent Bao Gong Teng A. Acta Pharm Sin 1979;14:731-4.
- Yao TR, Chen ZN, Yi DN, Xu GY. Chemical investigation of Bao Gong Teng (Erycibe obtusifolia Benth). Acta Pharm Sin 1981;16:582-8.
- Sun C. Studies on the new drug Bao Jia Su benzoate-A prasymp-thomimetic tropane isolated from a Chinese medicinal herb Bao Gong Teng. Acta Univ Med Sec Shanghai 1987;1:100-4.
- Zhou WB. A preliminary report on treatment of glaucoma with an alkaloid from erycibe obtusifolia Benth. Zhonghua Yan Ke Za Zhi 1981;17:65-8.
- Wang LP. Myotic activities of Baogongteng A and its analogues. Chin Tradit Herb Drugs 1992;23:205-8.
- Xiang Z, Zhou JE, Chen ZN, Wang LP, Wang HN, Yao TR, et al. Studies on synthesis of baogongteng A—a new myotic agent. Acta Pharm Sin 1989;24:105-9.
- Yang L, Wang H. The preparation and bioactivities of chiral analogs of baogongteng A. Acta Pharm Sin 1998;33:832-5.
- Song X, Wang H, Yao TR. Analogs of Baogongteng A. Chin Pharm J 1995;30:168-71.
- Niu YY, Yang LM, Lu Y. Study of synthesis and stereochemistry of Baogongteng A and its analogs. Chem World 2003: 491–5.
- Daly JW, Gupta TH, Padgett WL, Pei XF. 6 beta-Acyloxy(nor)tropanes: affinities for antagonist/agonist binding sites on transfected and native muscarinic receptors. J Med Chem 2000;43:2514-22.
- Zhang Y, Liebeskind LS. Organometallic enantiomeric scaffolding: organometallic chirons. Total synthesis of (-)-Bao Gong Teng A by a molybdenum-mediated [5 + 2] cycloaddition. J Am Chem Soc 2006;128:465-72.
- Waelbroeck M, Hou X, Wehrle J, Mutschler E, Van Tilburg E, Menge W, et al. Stereoselective interaction of uncharged esters at four muscarinic receptor subtypes. Eur J Pharmacol 1996;303:221-6.
- Gao ZG, Liu CG. Competitive and allosteric binding of 2 alpha-DHET and its optical isomers to rat cardiac muscarinic receptors. Eur J Pharmacol 1995;289:369-73.
- Scapecchi S, Matucci R, Bellucci C, Buccioni M, Dei S, Guandalini L, et al. Highly chiral muscarinic ligands: the discovery of (2S,2'R,3'S,5'R)-1-methyl-2-(2-methyl-1,3-oxathiolan-5-yl)pyrrolidine 3-sulfoxide methyl iodide, a potent, functionally selective, M2 partial agonist. J Med Chem 2006;49:1925-31.
- Cembala TM, Sherwin JD, Tidmarsh MD, Appadu BL, Lambert DG. Interaction of neuromuscular blocking drugs with recombinant human m1-m5 muscarinic receptors expressed in Chinese hamster ovary cells. Br J Pharmacol 1998;125:1088-94.
- Caulfield MP, Birdsall NJ. International Union of Pharmacology. XVII. Classification of muscarinic acetylcholine receptors. Pharmacol Rev 1998;50:279-90.
- Konno T, Ohnuma SY, Uemoto K, Uchibori T, Nagai A, Kogi K, et al. Effects of 2-alkynyladenosine derivatives on intraocular pressure in rabbits. Eur J Pharmacol 2004;486:307-16.
- Manni G, Lambiase A, Centofanti M, Mattei E, De Gregorio A, Aloe L, et al. Histopathological evaluation of retinal damage during intraocular hypertension in rabbit: involvement of ganglion cells and nerve fiber layer. Graefes Arch Clin Exp Ophthalmol 1996;234 Suppl 1:S209-13.
- Zhu MD, Cai FY. Development of experimental chronic intraocular hypertension in the rabbit. Aust N Z J Ophthalmol 1992;20:225-34.
- Bucolo C, Campana G, Di Toro R, Cacciaguerra S, Spampinato S. Sigma 1 recognition sites in rabbit Iris-Ciliary body: topical sigma 1-site agonists lower intraocular pressure. J Pharmacol Exp Ther 1999;289:1362-9.
- Barilan A, Nachman-Rubinstein R, Oron Y, Geyer O. Muscarinic blockers potentiate beta-adrenergic relaxation of bovine iris sphincter. Graefes Arch Clin Exp Ophthalmol 2003;241:226-31.
- Gil D, Spalding T, Kharlamb A, Skjaerbaek N, Uldam A, Trotter C, et al. Exploring the potential for subtype-selective muscarinic agonists in glaucoma. Life Sci 2001;68:2601-4.
- Ishizaka N, Noda M, Yokoyama S, Kawasaki K, Yamamoto M, Higashida H. Muscarinic acetylcholine receptor subtypes in the human iris. Brain Res 1998;787:344-7.
- Woldemussie E, Feldmann BJ, Chen J. Characterization of muscarinic receptors in cultured human iris sphincter and ciliary smooth muscle cells. Exp Eye Res 1993;56:385-92.
- Gil DW, Krauss HA, Bogardus AM, WoldeMussie E. Muscarinic receptor subtypes in human iris-ciliary body measured by immunoprecipitation. Invest Ophthalmol Vis Sci 1997;38:1434-42.
- Collison DJ, Coleman RA, James RS, Carey J, Duncan G. Characterization of muscarinic receptors in human lens cells by pharmacologic and molecular techniques. Invest Ophthalmol Vis Sci 2000;41:2633-41.
- Yu AY, Sun C. 6 beta-Acetoxy nortropane and its muscarinic receptor kinetics. Acta Pharmacol Sin 1990;11:394-400.
- Sun C, Yu AY, Yao WZ, Feng JM, Cui YY. The pharmacological and clinical studies of 6 beta-acetoxynortropane in ophthalmology. Acta Univ Med Sec Shanghai 1991;11:199-203.
- Qiu Y, Chen HZ, Wu XJ, Jin ZJ. 6 beta-Acetoxy nortropane regulated processing of amyloid precursor protein in CHOm1 cells and rat brain. Eur J Pharmacol 2003;468:1-8.
- Pei XF, Gupta TH, Badio B, Padgett WL, Daly JW. 6beta-Acetoxynortropane: a potent muscarinic agonist with apparent selectivity toward M2-receptors. J Med Chem 1998;41:2047-55.
- Yoon TP, Jacobsen EN. Privileged chiral catalysts. Science 2003;299:1691-3.
- Koeller KM, Wong CH. Enzymes for chemical synthesis. Nature 2001;409:232-40.
- Srinivas NR. Drug disposition of chiral and achiral drug substrates metabolized by cytochrome P450 2D6 isozyme: case studies, analytical perspectives and developmental implications. Biomed Chromatogr 2006;20:466-91.
- Ariens EJ. Racemic therapeutics—ethical and regulatory aspects. Eur J Clin Pharmacol 1991;41:89-93.