Liposome-mediated IL-28 and IL-29 expression in A549 cells and anti-viral effect of IL-28 and IL-29 on WISH cells1
Introduction
Human interleukin (IL)-28A, IL-28B, and IL-29, belonging to the IL-28 family, are expressed by human peripheral blood mononuclear cells (PBMC)[1,2] and monocyte-derived dendritic cells[1–3] after virus infection or stimulation with the poly I:C double-stranded RNA polymer. The genes for all 3 members of the IL-28 family are clustered on chromosome 19 in the q13.13 region[1,2]. Although the IL-28 family genomic structure resembles that of the IL-10 family, their proteins are structurally more closely related to the type I interferons (IFN) than to IL-10[4–11]. They have therefore been described independently as IFN-λ1 (IL-29), IFN-λ2 (IL-28A), and IFN-λ3 (IL-28B)[2]. IL-28A, IL-28B, and IL-29 share a common heterodimeric receptor, in which one subunit is a novel member of the class II cytokine receptor family (IL-28R or IFN-λR) and the other is identical to the second chain of the IL-10 receptor (IL-10R). Both receptor subunits are constitutively expressed in a wide variety of human cell lines and tissues[1,2], and signal through the Janus kinases-signal transducers and activators of transcription (Jak-STAT) pathway[1,2,12]. It is well documented that this receptor-ligand system is likely to contribute to antiviral or other defense activities[1,2,12].
In order to further understand the potential physiological and pathophysiological roles of IL-28 and IL-29 in humans and to make some valuable experimental tools for the production of recombinant proteins of IL-28 and IL-29, we constructed recombinant eukaryotic vectors expressing IL-28 and IL-29 cDNAs, and investigated the feasibility of liposome transfection of human A549 cells to express functional recombinant human IL-28 and IL-29.
Materials and methods
RNA isolation and RT-PCR PBMC were isolated using a multi-step Percoll gradient (Sigma, St Louis, MO, USA) from fresh peripheral blood of healthy volunteers as described previously[13]. The isolated cells were then cultured in RPMI-1640 culture medium containing 10% fetal calf serum (FCS), 2 mmol/L L-glutamine, 20 mmol/L N-2-hydroxyethylpiperazine-N'-2-ethanesulfonic acid (HEPES), 100 kU/L penicillin, 100 mg/L streptomycin at 37 °C in a 5% CO2 humidified atmosphere for 3 h prior to incubation with 50 to 100 mg/L poly I:C (Sigma) for 12 to 20 h at 37 ºC. Total cellular RNA was extracted from activated PBMC with Trizol RNA extraction solution following the protocol provided by the manufacturer (Invitrogen, Carlsbad, CA, USA). The concentration of RNA extracted was determined at wavelengths of 260 nm and 280 nm using a Beckman Coulter DU 640 Nucleic Acid and Protein Analyzer. The quality of RNA was determined by agarose gel electrophoresis. The primer sequences of the IL-28 family were designed with Primer Premier 5.0 software. All primers were synthesized by Bioasia Bioengineering (Shanghai, China). The sequences of the synthetic primers used for cloning are shown in Table 1. Of these primers, P1 and P2 were used for IL-29 polymerase chain reaction (PCR), P3 and P4 for IL-28A PCR, and P5 and P6 for IL-28B PCR. Kozak sequences[14,15] (GCC
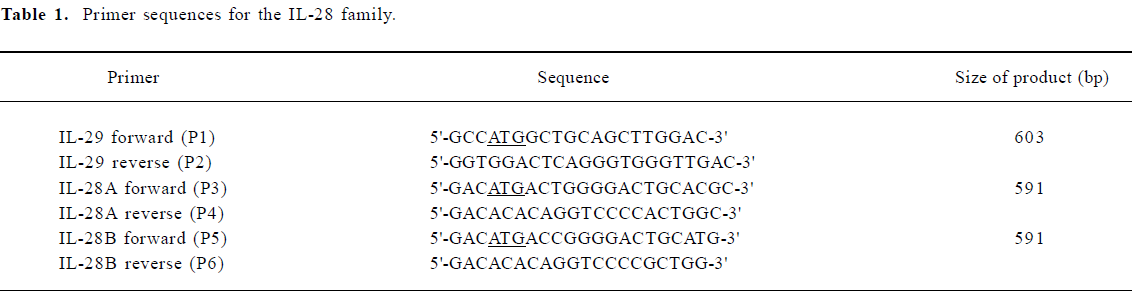
Full table
Generation of recombinant vectors The PCR products were separated in 1% agarose gels. The bands containing IL-28A, IL-28B, and IL-29 cDNAs were cut out and recovered from the gel using a QIAquick gel extraction kit (Qiagen, Hilden, Germany). The purified IL-28A, IL-28B, and IL-29 cDNA fragments were inserted into the pcDNA3.1/V5-His-TOPO vector (Invitrogen) using the TOPO cloning technique according to the manufacturer’s instructions. The PCR product and pcDNA3.1/V5-His-TOPO vector were mixed gently together and incubated for 5 min at room temperature. The reaction mixture was placed on ice, the vector was transformed into competent Escherichia coli TOP10 by using the CaCl2 method and then transformed clones were selected on agar plates containing ampicillin as described previously[16]. The positive recombinant clones were confirmed by colony-PCR and restriction enzyme digestion[16]. The sequences of the inserted fragments were confirmed by DNA sequencing, which was performed by Bioasia Bioengineering. The sequences were compared with those published in GenBank by using the BLAST algorithm[17].
Cell culture and transfection experiments The A549 cells, a type II alveolar epithelial cell line from human adenocarcinoma, were obtained from the Shanghai Institute for Cell Biology, Chinese Academy of Sciences (Shanghai, China). A549 cells were routinely grown in Dulbecco’s modified Eagle’s medium (DMEM) supplemented with 10% FCS in a well humidified atmosphere of 5% CO2 at 37 °C. Large-scale plasmid DNA preparations were produced by using the EndoFree plasmid Maxi kit (Qiagen). Transfection of pcDNA3.1/V5-His-TOPO-IL-28/IL-29 was conducted with Lipofectamine 2000 (Invitrogen) in 6-well plates according to the manufacturer’s instructions. pcDNA3.1/V5-His-TOPO/lac Z (Invitrogen) was used as an expression control vector to check transfection efficiency and optimize the transfection conditions. Untransfected A549 cells and liposome-DNA wells without A549 cells also served as controls. The day before transfection, A549 cells were collected, and seeded into 6-well plates at 1×105 cells per well in order to achieve 70%–80% confluence on the day of transfection. Lipofectamine 2000, diluted in serum-free DMEM, was added to the plasmid mixture and incubated for 20 min at room temperature. A549 cells were transfected by adding 3 µg plasmid and 8 µL of the original concentration of Lipofecta-mine 2000 per well. After 24 h, the transfected A549 cells were selected in the antibiotic G418 (1 g/L) for 2 weeks. The transfection efficiencies were estimated by using a β-Gal staining kit (Invitrogen) according to the manufacturer’s instructions. In transfected cells, β-galactosidase cleaved X-gal to produce a blue stain. The transfected cells were harvested and counted at 24, 36, 48, 60, 72, and 84 h after transfection and were observed under a microscope. The transfection efficiencies were the percentage of stained cells in the total population. The resistant clones containing IL-28/IL-29 mRNAs and proteins were identified by semiquan-titative RT-PCR and Western blotting, respectively.
Semiquantitative RT-PCR analysis Trizol reagent (Invitrogen) was used to extract total RNA from A549 cells that had been incubated with IL-28A, IL-28B, and IL-29 DNA transfection media 48 h after transfection. RT-PCR amplification was performed using the One Step RNA PCR kit (Qiagen) with the primers as described earlier. All reverse transcription products were also subjected to glyceraldehyde-3-phosphate dehydrogenase (GAPDH) gene amplification, which served as an internal standard. The primer set 5'-CGGAGTC-AACGGATTTGGTGGTAT-3' and 5'-AGGCTTCTCCATGG-TGGTAGAGAC-3' was used to amplify a GAPDH region of 306 bp. PCR conditions involved denaturation at 94 °C for 30 s, annealing at 60 °C for 30 s, and extension at 72 °C for 30 s for a total of 30 cycles. The RT-PCR products were detected by electrophoresis on 1% agarose gel and the bands were visualized by SYBR Green I nucleic acid gel stain (Cambrex, Rockland, ME, USA). Each band was analyzed using the Syngene Image Analysis System (Cambridge, UK). The gene expression level was determined semiquantitatively by calculating the ratio of densitometric value from the IL-28 or IL-29 gene in relation to the internal standard.
Western blot analysis The cell culture supernatants, collected from A549 cells transfected with IL-28/IL-29 cDNA after 48 h, were concentrated by ultrafiltration and filtered through a 0.45 mm membrane. The proteins were then separated by sodium dodecyl sulfate-polyacrylamide gel electrophoresis (SDS-PAGE) before being transferred to polyvinyli-dene fluoride (PVDF) membranes (Millipore, Bedford, MA, USA). The membranes were first incubated with 4% bovine serum albumin (BSA) and 0.02% Tween-20 in phosphate-buffered saline (PBS) at room temperature for 1 h in order to block non-specific protein binding sites, and then incubated with a 1:1000 dilution of mouse anti-His6 antibody (Invitrogen) at 37 ºC for 30 min. After the membranes were washed, a 1:1500 dilution of horseradish peroxidase conjugated goat anti-mouse IgG was added to the membranes for 30 min at 37 ºC. Visualization was conducted with diaminobenzidine (DAB). Each band was analyzed on Syngene Image Analysis System. The protein expression level was determined by calculating the ratio of densitometric value in the pcDNA3.1/V5-His-TOPO-IL-28/IL-29-transfected group in relation to that of the pcDNA3.1/V5-His-TOPO-IL-28A-transfected group.
Antiviral assay The antiviral activity of IL-28 and IL-29 was assessed on the basis of their protective effect on human immortalized amnion epithelial cell line (WISH cells) against vesicular stomatitis virus (VSV) infection as described by Familletti et al[23] and Sheppard et al[1] with some modifica-tions. A commercial preparation of IFN α-2b (intron A) obtained from Schering-Plough (County Cork, Ireland) was employed as the positive control. Protein concentration was determined by using the Coomassie Plus protein assay kit (Pierce, Rockford, IL, USA) according to the manufacturer’s instructions. Briefly, cell culture supernatants (IL-28 or IL-29) were concentrated by ultrafiltration and filtered through a 0.45 mm membrane. IL-28 or IL-29 and IFN α-2b were diluted in Eagle’s minimum essential medium (MEM) containing 10% FBS to a final concentration of 10 µg/L (assay starting concentration). An equal number of WISH cells was plated in each well of 96-well plates and treated with 2-fold serial dilutions of human IFN α-2b, IL-28, or IL-29, respec-tively, for 24 h. The cells were then infected with VSV until controls (medium alone wells) were fully killed by the virus (1–2 d). Cells that were not killed by virus were visualized by staining with crystal violet. The experiments were repeated 3 times in duplicate. Antiviral activity was expressed as the amount of IFN α-2b, IL-28, or IL-29 required for 50% protection of WISH cells against death caused by VSV infection.
Statistical analysis All data are expressed as mean±SD and statistically analyzed by using the t-test. P<0.05 was considered significant.
Results
Construction of recombinant vectors The pcDNA3.1/V5-His-TOPO is supplied linearized with single 3' deoxythymidine (T) overhangs for TA cloning. Taq polymerase has a nontemplate-dependent terminal transferase activity that adds a single deoxyadenosine (A) to the 3' ends of PCR products. This allows PCR inserts to ligate efficiently into the vector. A map of the constructed pcDNA3.1/V5-His-TOPO-IL-28/IL-29 plasmid is shown in Figure 1. Kozak sequences were included at the translational initiation sites by designing the 5' PCR primer. The PCR products were expressed as a fusion to the V5 epitope and a polyhistidine tag for detection or purification.
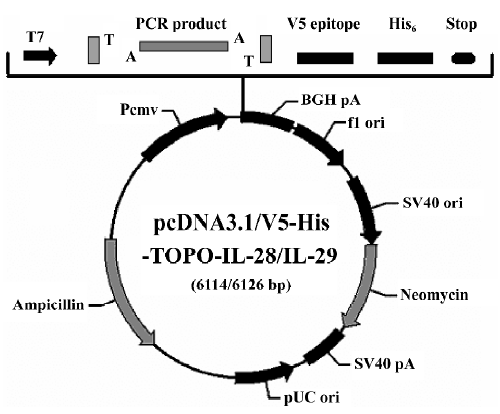
Identification and transfection efficiency of the constructed vectors After single digestion of pcDNA3.1/V5-His-TOPO-IL-28A/IL-28B with the restriction enzyme XhoI (Promega, Madison, WI, USA), 2 DNA fragments of approximately 5.7 kb and 115 bp were released as expected (Figure 2). After digestion of pcDNA3.1/V5-His-TOPO-IL-29 with restriction enzyme PstI (Promega), 3 DNA fragments of approximately 4.7 kb, 1.5 kb, and 600 bp were released as expected (Figure 2).
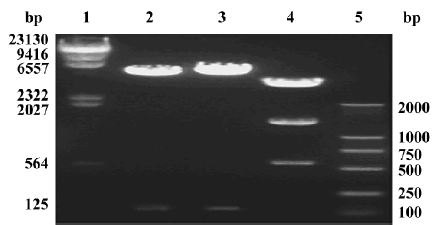
The insert DNA sequences encoding IL-28A, IL-28B, and IL-29 were analyzed by an automatic sequence analyzer and, by using the BLAST algorithm, were found to have 100% identity to the published sequences in GenBank[17]. Kozak sequences at the 5' translational initiation sites of IL-28A, IL-28B, and IL-29 cDNAs were designed. The flanking nucleotide sequences around the starting codon fulfilled Kozak’s rule (GACATGA and GCCATGG)[14,15]. In addition, more than 20 clones of IL-28B were sequenced and 5 of these clones were identified as a new sequence by bidirectional and repetitive sequence analysis. This sequence is very closely related to IL-28B. There are 5 base differences between it and IL-28B, which cause 3 amino acid differences. The nucleotide sequences of the modified IL-28A, IL-28B, and IL-29 and this novel sequence were accepted by GenBank (acces-sion N
A549 cells were transfected with pcDNA3.1/V5-His-TOPO/lac Z and stained with X-gal; the percentage of cells staining blue was in the range of 20%–45%. A maximum transfection efficiency was achieved 48 h after transfection and was approximately 45% (Figure 3).
IL-28 and IL-29 mRNA expression in transfected A549 cells Total RNA extracted from A549 cells was reverse transcribed in order to determine if IL-28A-, IL-28B-, and IL-29-transfected cells could express IL-28A, IL-28B, and IL-29 mRNAs. Using RT-PCR techniques with IL-28 or IL-29 primers, approximately 600 bp fragments were found in pcDNA3.1/V5-His-TOPO-IL-28/IL-29-transfected cells, but not in untransfected A549 cells (Figure 4). No significant difference in mRNA levels was found among IL-28A, IL-28B, and IL-29 (P<0.05). Using RT-PCR techniques with GAPDH primers, a 306 bp fragment was found not only in pcDNA3.1/V5-His-TOPO-IL-28/IL-29-transfected A549 cells, but also in the pcDNA3.1/V5-His-TOPO/lac Z-transfected cells and in the negative control (untransfected) A549 cells (Figure 4). No fragment was found in the liposome-DNA transfection control without A549 using RT-PCR with IL-28, IL-29, or GAPDH primers (Figure 4).
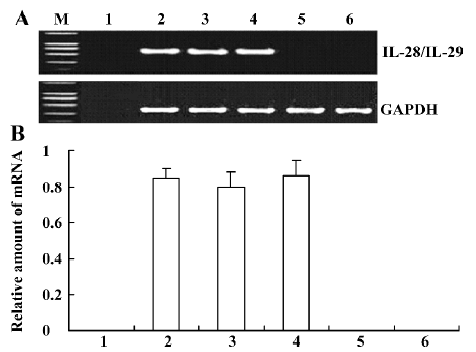
IL-28 and IL-29 protein expression in transfected A549 cells The inserted IL-28A, IL-28B, and IL-29 genes were significantly expressed in the eukaryotic expression system. This was confirmed by the finding that the clearly blocked bands in Western blots appeared at approximately 25 kDa for IL-28A or IL-28B, and in the region between 23 and 36 kDa for IL-29, with a major band at approximately 36 kDa. The level of the major 36 kDa IL-29 protein expression was higher than those of IL-28A and IL-28B (Figure 5). The C-terminal peptide, containing the V5 epitope and the polyhistidine region, added approximately 5 kDa to the fusion proteins. There is a possible site for N-linked glycosylation (NTS) in IL-29 that is not present in IL-28A or IL-28B[2]. The glycosyla-tion of IL-29 makes it up to 11 kDa heavier.
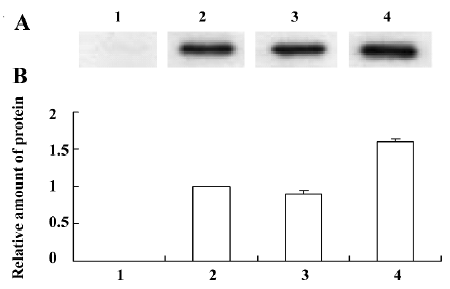
Antiviral activity The antiviral activity of the IL-28 and IL-29 expressed in the culture supernatants of A549 cells was estimated by their protective effect on WISH cells against infection by VSV and compared with a commercial preparation of IFN α-2b (intron A). Antiviral activity was measured as the amount of IFN α-2b, IL-28, or IL-29 required for 50% protection of the cells from cytopathic effect (CPE). The IL-28 and IL-29, like IFN α-2b (intron A), were able to protect WISH cells from viral-induced cytopathic effect when applied 24 h before adding VSV. The antiviral activity of IL-29 was higher than that of IL-28A or IL-28B, but lower than that of IFN α-2b (Figure 6).
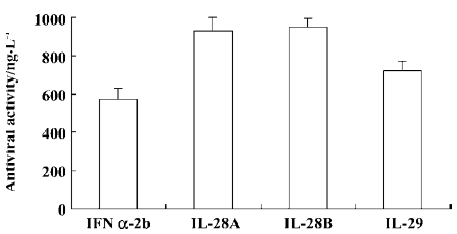
Discussion
In the present study we developed new plasmid vectors containing human IL-28 and IL-29 cDNAs, that is, pcDNA3.1/V5-His-TOPO-IL-28/IL-29, and investigated their expression in mammalian cells in vitro. Plasmid DNA-liposome complexes were transfected into A549 cells, and successful transcription was confirmed by RT-PCR and Western blotting in transfected A549 cells. In addition, we demonstrated that IL-28 and IL-29 had specific antiviral activity similar to type I IFN (IFN α-2b) in VSV-infected human WISH cells.
The pcDNA3.1/V5-His-TOPO vector is a highly efficient, one-step cloning strategy for the direct insertion of Taq polymerase-amplified PCR products into a plasmid vector. No ligase, post-PCR procedures or PCR primers containing specific sequences are required. The PCR product can be expressed as a fusion to the V5 epitope and polyhistidine tag for detection and purification; or, by designing the 3' PCR primer with a stop codon, the PCR product can be expressed as a native protein. For the sake of easier detection and purification of recombinant IL-28 and IL-29 proteins, the pcDNA3.1/V5-His-TOPO-IL-28/IL-29 fusion expression vector was constructed. A specific antibody against the polyhistidine C-terminus was used to detect the fusion proteins of IL-28 and IL-29 in the present study.
The pcDNA3.1/V5-His-TOPO vector does not contain an ATG initiation codon. When we designed the PCR primers to clone our DNA sequences, an ATG initiation codon and optimal sequences for translation initiation (Kozak sequences) were incorporated into forward (sense) primers. The Kozak sequence is contiguous to the ATG start codon, which can greatly increase the efficiency of translation and subsequently the overall expression of the target gene[14,15].
Gene transfer involves the delivery of a therapeutic protein-encoding nucleic acid to target cells. The DNA consists of one or more genes to be expressed in the target cell and the sequences controlling their expression. A variety of gene transfer vectors, including retroviruses, adenoviruses, adeno-associated viruses, liposomes, and molecular conjugates, have been experimentally used in gene transfer to many different types of cells and organs[18,19], each having its advantages and disadvantages. Viral vectors significantly increase the efficacy of transfection, but viral proteins may elicit an immune response within the targeted host or tissue. Although non-viral gene delivery is generally associated with low transfection efficiency and transient expression of the gene product, there are several reasons for us to choose a plasmid-liposome (capsules made from lipids) complex to transfect the A549 cell line. First, they are easy to handle and can apply to a wide spectrum of cells[20–22]. Second, they are relatively non-immunogenic as a result of lack of proteins. Third, they can carry exogenous material of essentially unlimited size. Finally, they cannot replicate or recombine to form infectious agents. Our results demonstrated that DNA-liposome transfection was an effective method for delivery of IL-28 and IL-29 plasmid DNA into A549 cells. IL-28 and IL-29 expressed in A549 cells by liposome-DNA transfection, like type I IFNs (IFN α-2b), were able to protect human WISH cells from virus-induced cell death. The antiviral activity of IL-28 and IL-29 generated in the current study confirmed at least that our transfection methods can produce functional IL-28 and IL-29. The potential of IL-28 and IL-29 in clinical applications is still unknown, but our results and those of other studies[1,2,12,24–28] indicate that these cytokines deserve further investigation for similar applications as already proven for type I IFNs such as viral infections and tumors.
In conclusion, IL-28 and IL-29 can be expressed in mammalian cells with liposome-DNA transfection, which provides a new tool for the functional study of those cytokines in humans.
Acknowledgement
We are grateful to Prof William ORR (Department of Pathology, University of Manitoba, Canada) for his critical reading of the manuscript.
References
- Sheppard P, Kindsvogel W, Xu W, Henderson K, Schlutsmeyer S, Whitmore TE, et al. IL-28, IL-29 and their class II cytokine receptor IL-28R. Nat Immunol 2003;4:63-8.
- Kotenko SV, Gallagher G, Baurin VV, Lewis-Antes A, Shen M, Shah NK, et al. IFN-λs mediate antiviral protection through a distinct class II cytokine receptor complex. Nat Immunol 2003;4:69-77.
- Coccia EM, Severa M, Giacomini E, Monneron D, Remoli ME, Julkunen I, et al. Viral infection and Toll-like receptor agonists induce a differential expression of type I and l interferon as in human plasmacytoid and monocyte-derived dendritic cells. Eur J Immunol 2004;34:796-805.
- Renauld JC. Class II cytokine receptors and their ligands: key antiviral and inflammatory modulators. Nat Rev Immunol 2003;3:667-76.
- Kotenko SV. The family of IL10-related cytokines and their receptors: related, but to what extent? Cytokine Growth Factor Rev 2002;13:223-40.
- Dumoutier L, Renauld JC. Viral and cellular interleukin-10 (IL-10)-related cytokines: from structures to functions. Eur Cytokine Netw 2002;13:5-15.
- Langer JK, Cutrone EC, Kotenko SV. The Class II cytokine receptor (CRF2) family: overview and patterns of receptor-ligand interactions. Cytokine Growth Factor Rev 2004;15:33-48.
- Li M, He S. IL-10 and its related cytokines for treatment of inflammatory bowel disease. World J Gastroenterol 2004;10:620-5.
- Dumoutier L, Lejeune D, Hor S, Fickenscher H, Renauld JC. Cloning of a new type II cytokine receptor activating signal transducer and activator of transcription (STAT)1, STAT2 and STAT3. Biochem J 2003;370:391-6.
- Pestka S, Krause CD, Sarkar D, Walter MR, Shi Y, Fisher PB. Interleukin-10 and related cytokines and receptors. Annu Rev Immunol 2004;22:929-79.
- Fickenscher H, Hor S, Kupers H, Knappe A, Wittmann S, Sticht H. The interleukin-10 family of cytokines. Trends Immunol 2002;23:89-96.
- Dumoutier L, Tounsi A, Michiels T, Sommereyns C, Kotenko SV, Renauld JC. Role of the interleukin-28 receptor tyrosine residues for antiviral and antiproliferative activity of IL-29/IFN-lambda 1: similarities with type I interferon signaling. J Biol Chem 2004;279:32269-74.
- Ebner S, Neyer S, Hofer S, Nussbaumer W, Romani N, Heufler C. Generation of large numbers of human dendritic cells from whole blood passaged through leukocyte removal filters: an alternative to standard buffy coats. J Immunol Methods 2001;252:93-104.
- Kozak M. An analysis of 5'-noncoding sequences from 699 vertebrate messenger RNAs. Nucleic Acids Res 1987;15:8125-48.
- Kozak M. Pushing the limits of the scanning mechanism for initiation of translation. Gene 2002;299:1-34.
- Sambrook J, Fritsch EF, Maniatis T. Molecular cloning: a laboratory manual. 3rd ed. Cold Spring Harbor: Cold Spring Harbor Laboratory Press; 2001.
- Altschul SF, Gish W, Miller W, Myers EW, Lipman DJ. Basic local alignment search tool. J Mol Biol 1990;215:403-10.
- Schwickerath O, Brouns G, Thrasher A, Kinnon C, Roes J, Casimir C. Enhancer-deleted retroviral vectors restore high levels of superoxide generation in a mouse model of CGD. J Gene Med 2004;6:603-15.
- Edelstein ML, Abedi MR, Wixon J, Edelstein RM. Gene therapy clinical trials worldwide 1989–2004: an overview. J Gene Med 2004;6:597-602.
- Oku N, Yamazaki Y, Matsuura M, Sugiyama M, Hasegawa M, Nango M. A novel non-viral gene transfer system: polycation liposomes. Adv Drug Deliv Rev 2001;52:209-18.
- Choi WJ, Kim JK, Choi SH, Park JS, Ahn WS, Kim CK. Low toxicity of cationic lipid-based emulsion for gene transfer. Biomaterials 2004;25:5893-903.
- Song D, Wang HL, Wang S, Zhang XH. 5-Hydroxytryptamine-induced proliferation of pulmonary artery smooth muscle cells are extracellular signal-regulated kinase pathway dependent. Acta Pharmacol Sin 2005;26:563-7.
- Familletti PC, Rubinstein S, Pestka S. A convenient and rapid cytopathic effect inhibition assay for interferon. Methods Enzymol 1981;78:387-94.
- Osterlund P, Veckman V, Siren J, Klucher KM, Hiscott J, Matikainen S, et al. Gene expression and antiviral activity of alpha/beta interferons and interleukin-29 in virus-infected human myeloid dendritic cells. J Virol 2005;79:9608-17.
- Brand S, Beigel F, Olszak T, Zitzmann K, Eichhorst ST, Otte JM, et al. IL-28A and IL-29 mediate antiproliferative and antiviral signals in intestinal epithelial cells and murine CMV infection increases colonic IL-28A expression. Am J Physiol Gastrointest Liver Physiol 2005;289:G960-8.
- Meager A, Visvalingam K, Dilger P, Bryan D, Wadhwa M. Biological activity of interleukins-28 and -29: comparison with type I interferons. Cytokine 2005;31:109-18.
- Brand S, Zitzmann K, Dambacher J, Beigel F, Olszak T, Vlotides G, et al. SOCS-1 inhibits expression of the antiviral proteins 2',5'-OAS and MxA induced by the novel interferon-lambdas IL-28A and IL-29. Biochem Biophys Res Commun 2005;331:543-8.
- Robek MD, Boyd BS, Chisari FV. Lambda interferon inhibits hepatitis B and C virus replication. J Virol 2005;79:3851-4.