Growth inhibitory effect and apoptosis induced by extracellular ATP and adenosine on human gastric carcinoma cells: involvement of intracellular uptake of adenosine1
Introduction
Extracellular adenosine triphosphate (ATP) and adenosine (ADO) are important signaling molecules in both the intracellular and extracellular microenvironments of cells. ATP exerts transient physiologic and pharmacologic effects on the organism because it can be rapidly metabolized to ADO by ectotriphosphatase, ectodiphosphatase, and 5'-nucleotidase, and maintained its low physiologic concentrations. However, extracellular ATP may reach high concentrations when released exocytotically from various cell types such as neurons, platelets, basophils, and mast cells, or when released nonexocytotically from damaged cells[1]. It is well known that extracellular ATP and ADO have widespread effects on physiologic activities and play important physiologic and pathophysiologic roles in the cardiovascular system. Recently, they have been implicated in the induction of apoptosis in several cancer cell types such as human histiocytic leukemia cell line U-937[2], the mouse neuroblastoma cell line N1E-115[3,4], pancreatic cancer cells[5], colorectal carcinoma cells[6], prostate carcinoma cells[7], rat pheochromocytoma cells[8,9], and so on.
The process of programmed cell death has been suggested to play an important role in cancer diseases. Cell death by apoptosis progresses through a series of well-regulated morphological and biochemical phases, including chromatin condensation and DNA fragmentation. Recent studies have shown that ATP and ADO induces apoptosis in various cell types through receptor-mediated and non-receptor-mediated pathway, namely, the extrinsic pathway and the intrinsic pathway[10,11]. For the extrinsic pathway, A1, A2A, A2B and A3 ADO receptors[12−16], and P2X1, P2X2, P2X7, P2Y1, P2Y2 receptors[1,4,6,17−20] appear to bear apoptosis in normal cells and carcinoma cells. For the intrinsic pathway, extracellular ATP and ADO seem to induce apoptosis in epithelial cancer cells originated from the breast, the colon, and the ovary or neuroblastoma cells by the conversion of ATP to ADO and intracellular uptake of ADO[3,21].
We have investigated the antiproliferation effects of extracellular ATP and ADO on human gastric cell line, HGC-27[22]. In an attempt to gain more insight into the mechanism of ATP- and ADO-induced apoptosis in tumor tissue, we report that ATP and ADO can induce apoptosis in HGC-27 cells, in which cellular uptake of ADO plays an important role. In present study we observed the growth inhibitory and apoptotic effects of ATP and its final metabolite, ADO, on the human undifferentiated gastric cancer (HGC)-27 cells.
Materials and methods
Drugs and reagents ATP, ADO, ADO diphosphate (ADP), adenosine monophosphate (AMP), acridine orange (AO), ethidium bromide (EB), 3-(4,5-dimethylthiazol-2-yl)-2, 5-diphenyl tetrazolium bromide (MTT), aminophylline and dipyridamole were purchased from Sigma. RNase, SDS, proteinase K, trypsin and agarose were purchased from Sino-American Biotec. RPMI 1640 medium was purchased from GIBCO. Fetal bovine serum (FBS) was purchased from Hangzhou Sijiqing Biotec. Pyridoxal-phosphate-6-azophenyl-2, 4-disulphonic acid tetrasodium salt (PPADS) was purchased from Tocris Cookson. ATP, ADP, AMP and ADO were dissolved in sterile phosphate-buffered saline (PBS) and stored at -20 °C.
Cell culture HGC-27 cells, obtained from Shanghai Cell Database of Chinese Academy of Sciences, were cultured in RPMI 1640 medium supplemented with 10% (v/v) FBS, 100 U•mL-1 of penicillin, and 100 μg•mL-1 streptomycin at 37 °C in a humidified, CO2-controlled (5%) incubator.
MTT assays The cell viability was determined by MTT assay[23]. The HGC-27 cells in exponential phase of growth were harvested and seeded in 96-well plates (Costar, USA) at a density of 10 000 cells per well, and cultured for 24 h. ATP, ADP, AMP or ADO (0.3 and 1 mmol•L-1) and control (PBS) was then added into the wells, and incubated continuously for 48 h at 37 °C with 5% CO2. A 20 μL sample of MTT solution (5 g•L-1 dissolved in PBS) was added to each well and the plates were incubated at 37 °C for 4 h. The supernatant was discarded and 150μL dimethylsulfoxide was added to dissolve the blue insoluble MTT formazan produced by mitochondrial succinate dehydrogenase. The absorbance was measured at 490 nm in a spectrophotometer (Zhengzhou Bosai Biotech, ht2010), the negative control well contained medium only. Other inhibitors such as aminophylline, PPADS and dipyridamole were added to the medium 30 min before incubating cells with ATP or ADO. All determinations were performed in quadruplicate and each experiment was repeated at least three times.
Morphological assessment of apoptotic cells induced by ATP or ADO Morphological assessments of apoptotic cells were carried out using the AO/EB double-staining method[24]. HGC-27 cells in exponential phase of growth were harvested and seeded in a 25 mL cultured flask. The cells were incubated for 24 h at 37 °C with 5% CO2, and then treated with 0.3 mmol•L-1 ATP or ADO for 48 h. Freshly isolated HGC-27 cells (1×106) were harvested in an Eppendorf centrifuge tube, centrifuged for 5 min at 1000 rpm and suspended in PBS containing fluorescence dye AO/EB (AO and EB were both at the concentration of 100 mg•L-1 in PBS). The cells were prepared and dropped on slides. The morphology of the cells was observed under fluorescence light microscope (UFX-II; Nikon, Japan) and photographed.
Agarose gel electrophoresis of DNA[25] After treatment with ATP or ADO (0.03, 0.1, 0.3, and 1 mmol×) for 72 h, HGC-27 cells (1×106) were harvested in an Eppendorf centrifuge tube and washed twice with PBS. The cells were resuspended in a cell lysis buffer (50 mmol/L Tris-HCl buffer, 20 mmol/L EDTA pH 8.0, 1% SDS) and then mixed by vortexing. After the cells were left to stand for 30 min on ice, proteinase K was added at a final concentration of 0.25 g•L-1. The cell lysates were incubated at 37 °C overnight in a water bath, and RNase was added at a final concentration of 0.5 g•L-1 and incubated at 37 °C for 1 h. The lysates were mixed with an equal volume of Tris-saturated phenol (1:1, v/v) and mildly shaken for 30 min. The mixture was centrifuged at 3000 rpm for 10 min at room temperature to separate the aqueous phase from the organic phase. Extraction of each aqueous phase was repeated, using the Tris-saturated phenol/chloroform/isopropanol (25:24:1, v/v) mixture. The aqueous phase was further extracted with an equal volume of chloroform. Two volumes of ice-cold ethanol and 0.1 volume of 3 mol•L-1 NaAc precipitated DNA were mixed in the final aqueous phase. At this point, the mixture could be stored overnight. DNA was recovered by centrifugation at 13 000 rpm for 20 min in an Eppendorf centrifuge tube. The supernatant was discarded, the DNA pellet was washed once with 70% ethanol, air-dried, and then redissolved in an appropriate volume of deionized distilled-water and electrophoresed for 3 h at a constant voltage of 60 mV on a 1.8% agarose gel containing 0.5 mg•L-1 EB, using an electrophoresis buffer (40 mmol•L-1 Tris/acetate buffer, 1 mmol•L-1 EDTA, pH 8.0). Each DNA sample contained bromophenol blue as a front-running dye. Ladder formation of oligonucleosomal DNA was made visible by ultraviolet transillumination and photographed using a Gel Imaging System (PE Company, USA).
Determination of apoptosis by flow cytometric analysis After the cells were incubated with the different concentration of ATP or ADO for 48 h, they were harvested by centrifugation, washed with ice-cold PBS once and fixed in 70% ethanol at 4 °C overnight. The cells were then washed once with ice-cold PBS and resuspended in PBS (pH 7.4) containing 0.5% pepsin, 5 mg•L-1 EB and RNase at room temperature for 30 min. Finally cells were analyzed by flow cytometry on a FACS420 (Becton Dickinson, USA) equipped with an argon ion laser (488 nm), using the HP-300 Consort 30 software to determine percentage of the apoptotic cells and the proportion of cells in G0/G1, S, G2/M phases of the cell cycle. The proliferation index (PI) of cells was calculated by the following formula:
Statistical analysis The data shown were mean values of at least three independent experiments and expressed as Mean±SD. Statistical analysis was performed by one-way ANOVA and the Student’s t-test, using statistical software SPSS 10.0. Statistical significance was set at a level of P<0.05.
Results
Effects of ATP or ADO on the cell cycle and PI of HGC-27 cells The cell cycle phase and PI value of HGC-27 cells changed, when exposed to ATP or ADO at concentrations of 0.03, 0.1, 0.3, 1 mmol•L-1 for 48 h. The proportion of cells in the G0/G1- phase of cell cycle was significantly increased, S-phase of cells and PI value were significantly decreased after sustained incubation of HGC-27 cells with ATP or ADO (0.1, 0.3, 1 mmol•L-1). The proportion of cells in G2/M-phase of cell cycle was significantly decreased, when exposed to ATP (0.1, 0.3, 1 mmol•L-1) or ADO (0.1, 0.3 mmol•L-1). These data suggest that ATP and ADO inhibited the cell proliferation via G0/G1- phase delay (Table 1 and Figure 1A, 1B).
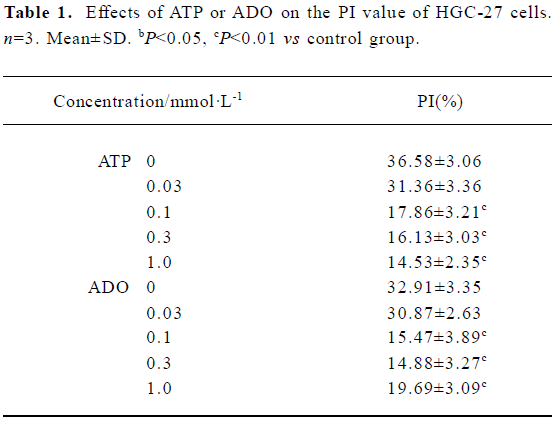
Full table
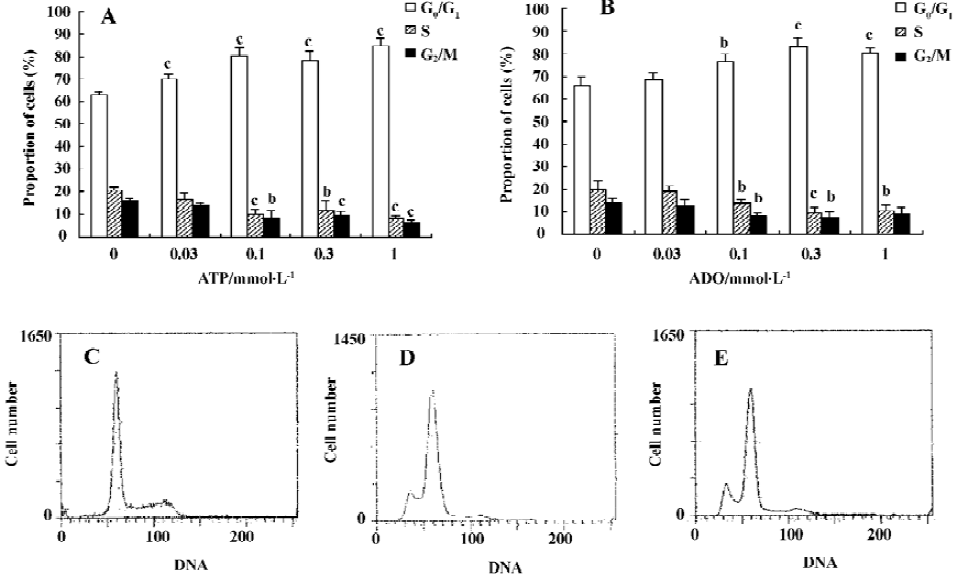
Morphological changes of HGC-27 cells induced by ATP or ADO Under fluorescence light microscope, the tumor cells exposed to 0.3 mmol•L-1 ATP or ADO displayed morphological changes of apoptosis by AO/EB double-staining, such as cell shrinkage, chromatin condensation, cell nuclear fragmentation, cell nucleous disappearance, increased nuclei fluorescence or labeled orange or red-orange color (Figure 2A-2C).
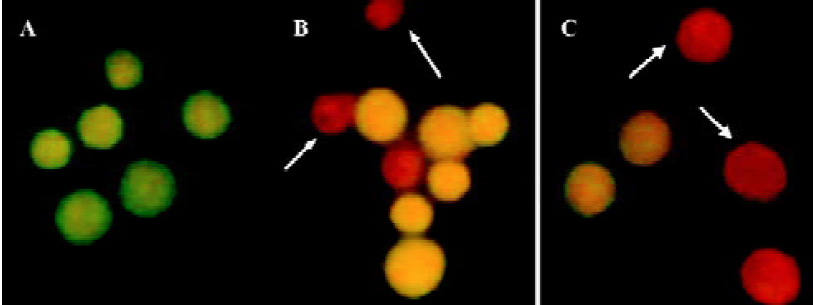
Agarose gel electrophoresis results of HGC-27 cells induced by ATP or ADO By the agarose gel electrophoresis, a ladder-like pattern of DNA fragmentation obtained from HGC-27 cells treated with 0.1‒1 mmol•L-1 ATP or ADO appeared in agarose gel electrophoresis, indicating that ATP and ADO induced apoptosis of HGC-27 tumor cells (Figure 3A, 3B).
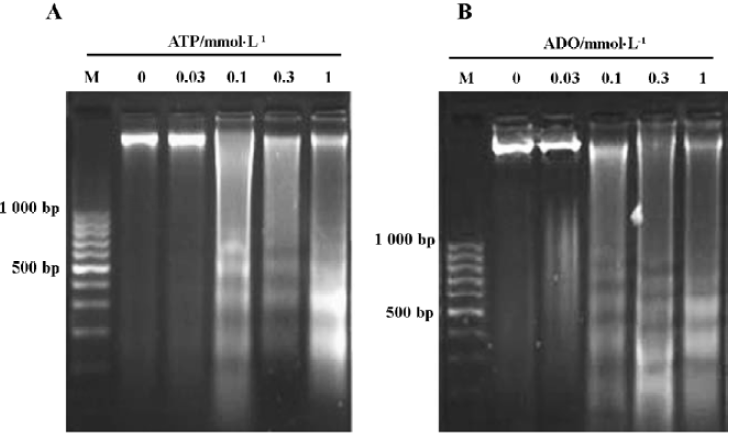
Apoptotic rate of HGC-27 cells induced by ATP or ADO The method used for this part was the analysis of the sub-G1 peak in the cell cycle. ATP or ADO induced the apoptosis of HGC-27 cells in a dose-dependent manner at concentrations between 0.03‒1 mmol•L-1 for 48 h. The apoptotic rate of HGC-27 cells treated with ATP or ADO were markedly higher than the control. The maximum apoptotic rate of HGC-27 cells exposed to ATP or ADO (1 mmol•L-1) for 48 h was (13.53±1.52)% or (15.90±1.15)%, respectively (Table 2 and Figure 4A-4E).
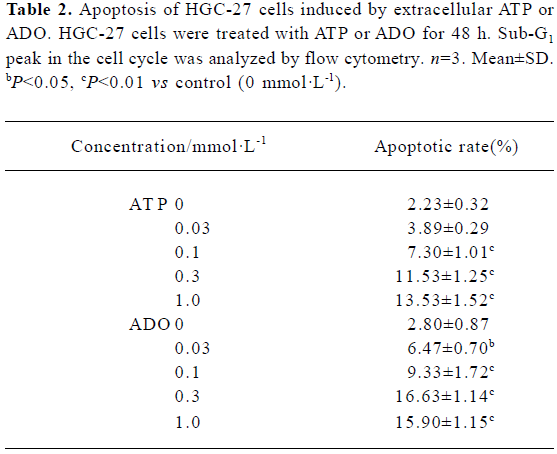
Full table
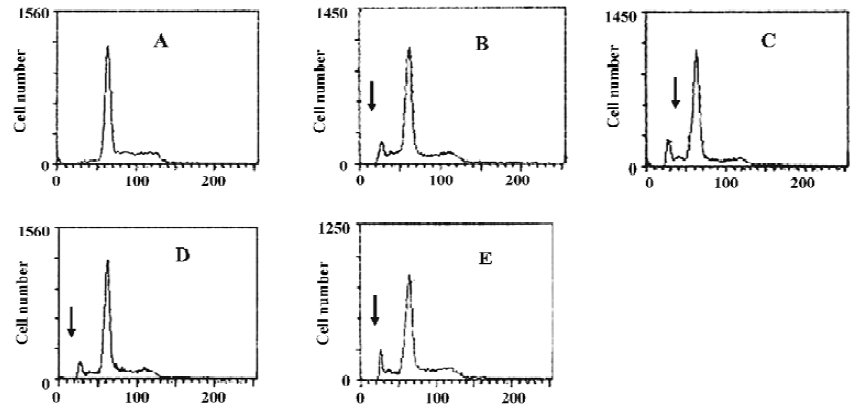
Effects of ATP metabolites, ADO-uptake inhibitors and antagonists of P receptors on cell viability The effects of ADP and AMP on HGC-27 cells were examined to find out whether the metabolites of ATP also inhibit the growth of HGC-27 cells. Both ADP and AMP reduced cell viability (Figure 5). We previously mentioned that ADO also caused apoptosis in these cells. Therefore, the inhibitors that were able to block ADO-induced apoptosis were tested to investigate the involvement of ADO in ATP-induced apoptosis. Dipyridamole is an inhibitor of the nucleoside transporter and blocks the transport of ADO into the cell. We showed that this inhibitor (10 μmol•L-1) was able to block ATP- and ADO-induced apoptosis in HGC-27 cells. The antagonists of P1 and P2 receptors, aminophylline and PPADS, were used. Both were non-selective antagonists of P receptors. Neither aminophylline (0.1 mmol•L-1) nor PPADS (30 μmol•L-1) were able to block the apoptosis induced by ATP and ADO (Figure 6).
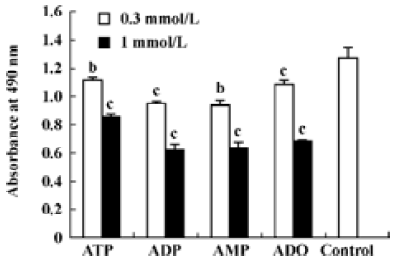
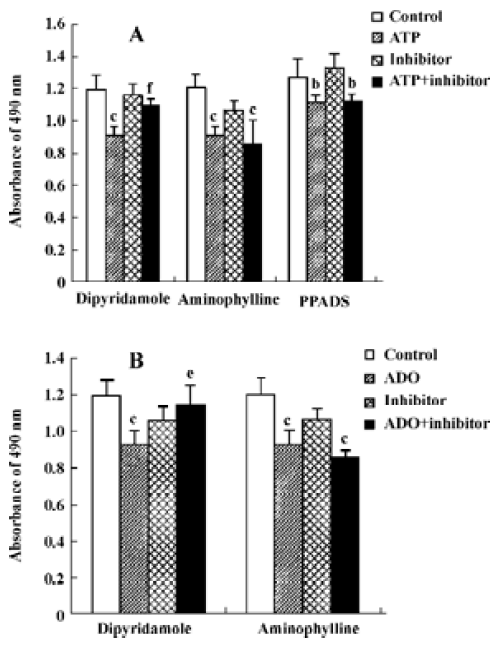
Discussion
ATP and its related compounds are widespread transmitters for extracellular communication in many cell types. By coupling to specific purinergic receptors, ATP is involved in a large variety of cellular functions. Receptors for purines and pyrimidines (P receptors) are divided into two major classes termed as ADO or P1 receptors, for which ADO is the principal natural ligand, and P2 receptors, for which ATP, ADP, UTP and UDP is the principal natural ligand. To date four P1 receptor subtypes have been identified, A1, A2A, A2B and A3, all coupled to G proteins with distinct tissue distribution and pharmacological properties. The P2 receptors are divided into two families: the ligand-gated ion channels (P2X) and the G protein-coupled receptors (P2Y)[20,26]. ATP and ADO are known to inhibit cell growth and to induce apoptosis in various tumor models[2-8,16,18,19,21,22,25,27,28]. Both growth inhibition and programmed cell death were previously considered to be mediated mainly by P1-receptors and P2-receptors[1,4,6,10,17−20]. Here we provide evidence that extracellular ATP induces apoptosis and causes cell-cycle arrest in human undifferentiated gastric cancer cells, and that ADO plays an important role in this.
In the present study, the HGC-27 cell line was chosen to study the possible role of ATP and ADO in human gastric cancer diseases. Our results show that ATP and ADO reduced cell viability, caused cell arrest and induced apoptosis in this cell line. Therefore, we investigated the mechanisms involved, in particular the role of ATP and ADO receptors and ADO transporters. We assessed which of the P receptors were present in HGC-27 cells and also whether nucleoside transporters were present. We used a series of inhibitors, including antagonists of P1 receptors and P2 receptors (ie, aminophylline and PPADS, respectively) and a blocker of ADO transporters, dipyridamole[3,4,26]. The effects of ATP and ADO on HGC-27 cell death were not affected by aminophylline and PPADS, indicating that P1 and P2 receptors might not mediate them. In contrast, they were significantly inhibited by dipyridamole, suggesting that ADO transporter might play an important role on the apoptosis induced by ATP and ADO.
Apoptosis induced by ADO in GT3-TKB human gastric cancer cells has already been suggested by Saitoh et al, who determined the effects of the ADO (0.1−20 mmol稬-1) on mitochondrial membrane potentials[27]. They demonstrated that ADO-induced GT3-TKB cell death was significantly inhibited by dipyridamole. Their results also rule out the possibility for the implication of P1 receptors. But the effects of ATP on GT3-TKB cells were not involved in their studies. Other studies, however, showed that some subtypes of P2 receptor had been implicated in the induction of apoptosis in several cell lines, such as P2X7 receptor in macrophages, microglial and dendritic cells; P2X2 receptor in rat pheochromocytoma cells, PC12; P2Y2 receptor in colorectal carcinoma cell lines (HT29, Colo320 DM) and oesophageal cancer cells, Kyse-140[4,6,17−19]. Abbracchio et al[29] and Kohno et al[16] also suggested the involvement of the A3 receptor in ADO-induced apoptosis using 2-Cl-IB-MECA (in rat astrocytes and the human promyelocytic leukemia cell line HL-60 cells, respectively). Both studies used high-agonist concentrations (10 mmol稬-1), rendering a selective effect question.
Normally, ATP is very rapidly broken down to ADP and AMP, and ADO is the final metabolite. Schrier et al[4] reported, ATP breakdown in a medium without cells was not as fast as that in medium with cells (t1/2: 4 h vs 30 min). ATP itself was completely broken down by N1E-115 cells in 60−120 min. We have shown that ADO could cause apoptosis in this cell line. ATP- and ADO-induced apoptosis were decreased by the inhibition of ADO-uptake using the nucleoside transport blocker dipyridamole. These results suggest that ATP induces apoptosis in HGC-27 cells via extracellular breakdown to ADO, and extracellular ATP and ADO induces apoptosis by their uptake into cells through ADO trans-porters. Thus, it was argued that the effects of ATP and ADO might be subsequent to the uptake of ADO by the cells. However, we could not exclude the possibility that the mechanism of apoptosis induced by ATP and ADO might also be due to the possible involvement of other routes as most antagonists of P receptors to date are non-selective and have other pharmacological effects. Therefore, further pathways leading to ATP- and ADO-induced apoptosis in HGC-27 cells need to be identified. Whether these same effects occur in vivo is yet to be established.
Additionally, Lu et al[28] reported growth inhibition of ATP (0.23 mg/mL) on MGC-803 human gastric cancer cells. It was found that ATP inhibited the proliferation and arrested cell cycle in the S-phase. Our results showed that ATP arrested cell cycle in the G0/G1 phase. The reason why our results were not in agreement with those of Lu et al[28] may be that we chose different kinds of gastric-cancer cell lines.
In conclusion, our data shows that extracellular ATP and ADO reduce cell viability, cause cell-cycle arrest and induce apoptosis. P receptors may not play a major role in ATP- and ADO-induced apoptosis in HGC-27 cells, but the uptake of ADO is required for apoptosis in this cell line. These data show that extracellular ATP and ADO are potent inducers of apoptosis. This makes ATP and ADO important compounds to consider when examining apoptosis in cancer diseases. We think that the investigation of further effects of ATP and ADO on tumor cells may provide innovative treatment strategies for gastric cancer.
References
- Nihei OK, de Carvalho AC, Savino W, Alves LA. Pharmacologic properties of P2Z/P2X7 receptor characterized in murine dendritic cells: role on the induction of apoptosis. Blood 2000;96:996-1005.
- Schneider C, Wiendl H, Ogilvie A. Biphasic cytotoxic mechanism of extracellular ATP on U-937 human histiocytic leukemia cells: involvement of adenosine generation. Biochim Biophys Acta 2001;1538:190-205.
- Schrier SM, van Tilburg EW, van der-Meulen H, Ijzerman AP, Mulder GJ, Nagelkerke JF. Extracellular adenosine-induced apoptosis in mouse neuroblastoma cells: studies on involvement of adenosine receptors and adenosine uptake. Biochem Pharmacol 2001;61:417-25.
- Schrier SM, Florea BI, Mulder GJ, Nagelkerke JF, Ijzerman AP. Apoptosis induced by extracellular ATP in the mouse neuroblastoma cell line N1E-115: studies on involvement of P2 receptors and adenosine. Biochem Pharmacol 2002;63:1119-26.
- Yamada T, Okajima F, Akbar M, Tomura H, Narita T, Yamada T, et al. Cell cycle arrest and the induction of apoptosis in pancreatic cancer cells exposed to adenosine triphosphate in vitro. Oncol Rep 2002;9:113-7.
- Hopfner M, Maaser K, Barthel B, von Lampe B, Hanski C, Riecken EO, et al. Growth inhibition and apoptosis induced by P2Y2 receptors in human colorectal carcinoma cells: involvement of intracellular calcium and cyclic adenosine monophosphate. Int J Colorectal Dis 2001;16:154-66.
- Janssens R, Boeynaems JM. Effects of extracellular nucleotides and nucleosides on prostate carcinoma cells. Br J Pharmacol 2001;132:536-46.
- Sun AY, Chen YM. Extracellular ATP-induced apoptosis in PC12 cells. Adv Exp Med Biol 1998;446:73-83.
- Bronte V, Macino B, Zambon A, Rosato A, Mandruzzato S, Zanovello P, et al. Protein tyrosine kinases and phosphatases control apoptosis induced by extracellular adenosine 5'-triphosphate. Biochem Biophys Res Commun 1996;218:344-51.
- Ferre S, Fredholm BB, Morelli M, Popoli P, Fuxe K. Adeno-sinedopamine receptor-receptor interactions as an integrative mechanism in the basal ganglia. Trends Neurosci 1997;20:482-7.
- Khoa ND, Montesinos MC, Reiss AB, Delano D, Awadallah N, Cronstein BN. Inflammatory cytokines regulate function and expression of adenosine A2A receptors in human monocytic THP-1 cells. J Immunol 2001;167:4026-32.
- Trincavelli ML, Falleni A, Chelli B, Tuscano D, Costa B, Gremigni V, et al. A2A adenosine receptor ligands and proinflammatory cytokines induce PC 12 cell death through apoptosis. Biochem Pharmacol 2003;66:1953-62.
- Bohm SK, Grady EF, Bunnett NW. Regulatory mechanisms that modulate signalling by G-protein-coupled receptors. Biochem J 1997;322:1-18.
- Wakade TD, Palmer KC, McCauley R, Przywara DA, Wakade AR. Adenosine-induced apoptosis in chick embryonic sympathetic neurons: a new physiological role for adenosine. J Physiol 1995;488:123-38.
- Tanaka Y, Yoshihara K, Tsuyuki M, Kamiya T. Apoptosis induced by adenosine in human leukemia HL-60 cells. Exp Cell Res 1994;213:242-52.
- Kohno Y, Sei Y, Koshiba M, Kim HO, Jacobson KA. Induction of apoptosis in HL-60 human promyelocytic leukemia cells by adenosine A3 receptor agonists. Biochem Biophys Res Commun 1996;219:904-10.
- Coutinho-Silva R, Perfettini JL, Persechini PM, Dautry-Varsat A, Ojcius DM. Modulation of P2Z/P2X7 receptor activity in macrophages infected with Chlamydia psittaci. Am J Physiol Cell Physiol 2001;280:C81-9.
- Maaser K, Hopfner M, Kap H, Sutter AP, Barthel B, von Lampe B, et al. Extracellular nucleotides inhibit growth of human oesophageal cancer cells via P2Y2-receptors. Br J Cancer 2002;86:636-44.
- Fujita N, Kakimi M, Ikeda Y, Hiramoto T, Suzuki K. Extracellular ATP inhibits starvation-induced apoptosis via P2X2 receptors in differentiated rat pheochromocytoma PC12 cells. Life Sci 2000;66:1849-59.
- Burnstock G. Purinergic signaling and vascular cell proliferation and death. Arterioscler Thromb Vasc Biol 2002;22:364-73.
- Barry CP, Lind SE. Adenosine-mediated killing of cultured epithelial cancer cells. Cancer Res 2000;60:1887-94.
- Wang MX, Ren LM, Shan BE. Effects of extracellular adenosine triphosphate and adenosine on tumor cell proliferation. Chin Pharmacol Bull 2003;19:1242-6.
- Situ ZQ, Wu JZ. Cell Culture. Xi-an: World Book’s Publishing Company; 1996. 186-8.
- Wang CY, Sheng RL, Wang F, Ding XJ, Qiu NL. Fluorescence method of apoptotic morphology studying by acridine orange and ethidium bromide double stained cells. Chin J Pathophysiol 1998;14:104-6.
- Kim KT, Yeo EJ, Choi H, Park SC. The effect of pyrimidine nucleosides on adenosine-induced apoptosis in HL-60 cells. J Cancer Res Clin Oncol 1998;124:471-7.
- Fredholm BB, Abbracchio MP, Burnstock G, Daly JW, Harden TK, Jacobson KA, et al. Nomenclature and classification of purinoceptors. Pharmacol Rev 1994;46:143-56.
- Saitoh M, Nagai K, Nakagawa K, Yamamura T, Yamamoto S, Nishizaki T. Adenosine induces apoptosis in the human gastric cancer cells via an intrinsic pathway relevant to activation of AMP-activated protein kinase. Biochem Pharmacol 2004;67:2005-11.
- Lu GZ, Lin ZX, Zhang ZQ, Zhou LX, Xu GW. Studies on mechanism about ATP inhibited the proliferation of MGC-803 cells. Shi Yan Sheng Wu Xue Bao 1994;27:137-9.
- Abbracchio MP, Ceruti S, Brambilla R, Franceschi C, Malorni W, Jacobson KA, et al. Modulation of apoptosis by adenosine in the central nervous system: a possible role for the A3 receptor. Pathophysiological significance and therapeutic implications for neurodegenerative disorders. Ann N Y Acad Sci 1997;825:11-22.