CPU0213, a novel endothelin receptor antagonist, ameliorates septic renal lesion by suppressing ET system and NF-κB in rats1
Introduction
An acute renal failure (ARF) that is frequently involved in septic shock is a major medical concerns because of its high morbidity and mortality[1]. Severe infection of Gram-negative bacteria in the peritoneal cavity occurs frequently or endotoxin participates in sepsis, which is implicated with the dysfunction of multiorgans. A higher mortality was reported in septic shock patients with ARF than in those whose renal failure was not predominant. Continuous efforts are being made to discover new drugs for treating ARF and to clarify the underlying mechanisms[2].
Endothelin-1 (ET-1) is released from the normal and diseased renal tissue[3] and exaggerates renal insult in animal models[4]. The findings from clinical investigations show that plasma levels of ET-1 are present in patients with end-stage renal insufficiency and high levels of ET-1 correlated with the extent of renal dysfunction in sepsis[5]. So it is of interest to investigate an altered ET-signaling pathway in the renal insult from septic shock. An enhancement of the ET-1 signaling pathway exists in septic shock, and the first endothelin receptor antagonist, bosentan, retrieves the abnormalities in the cardiovascular system[6,7].
A novel endothelin receptor antagonist, CPU0213, was discovered by screening newly synthetic compounds in our laboratory. The potency to block the ETA and ETB receptors tested by radio receptor binding in IC50 is 2.57±1.41 and 95±34 nmol/L, respectively. The pA2 assessed by functional suppression on the activity mediated by the ETA and ETB is 8.52±0.26 and 7.28±0.04, respectively, and is more potent than that of bosentan[8,9]. It is effective in combating pulmonary hypertension, and relieving acute and chronic cardiac failure in rats. The plasma half-life of CPU0213 is not long enough (within 2 h), but the effectiveness has been proved using one daily oral dose [8–10].
The aim of the present study was to test the efficacy of CPU0213, which blocks both the ETA and ETB receptors when treating ARF in septic shock rats. There has always been controversy over whether the ETA receptor only or both the ETA and ETB receptors are activated in certain pathological progression. The nuclear factor kappa B (NF-κB) is an important factor in regulating the transcription process and is likely implicated in the renal insult of septic shock mediated by an activated ET pathway. It is hypothesized that an activated ET signaling pathway in which both the ETA and ETB receptors are activated plays a critical pathological role in promoting the acute renal dysfunction in septic shock. The upregulation of mRNA levels of the ET signaling pathway are possibly modulated by an activated NF-κB and renal abnormalities in septic shock could be attenuated following the application of the novel endothelin receptor antagonist CPU0213. CPU0213 was used to treat rats with septic shock caused by acute diffuse peritonitis due to a leakage of feces from the cecal ligation and puncture (CLP). The ability of CPU0213 to attenuate the abnormalities of ARF are possibly mediated by suppressing an activated ET pathway and upregulated NF-κB.
Materials and methods
Animals, drug and experimental protocols Fifty-four male adult Wistar rats (250–280 g, Animal Center of Nanjing Medical University) received a standard diet and free water. The treatment of the animals adhered to the guidelines of the Heath Science Center of Nanjing (Jiangsu). The animals were allowed to readjust to the new housing environment for 1 week before the experiments. CPU0213 (Figure 1) was provided from the Center of New Drug Research of China Pharmaceutical University.
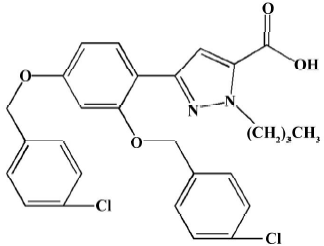
Septic shock was induced in the rats by CLP procedures according to the published literature[11]. Eight hours after surgery, the operated rats were divided randomly into 2 groups: the untreated septic shock group and CPU0213 (30 mg·kg-1·d-1 ×3 d, bid, sc) treated group. The same volume of normal saline was injected sc in the sham operation and untreated septic shock group.
Measurement of urinary protein and creatinine The 24-h urine collection of each rat was acidified with 0.1 mol/L HCl, separated by centrifugation, and the supernatant was collected and stored at -70 oC before analysis. The urinary protein and creatinine were determined by a spectrophotometric method with kits (Nanjing Jiancheng Bio-engineering Company, Nanjing, China).
Survival rate, hemodynamics and samples prepared The survival rate of rats was monitored within 72 h following surgical operation. At 72 h after operating, the rats were anesthetized with urethane, and a polyethylene catheter (PE 50, ID 0.58 mm, OD 0.965 mm, Becton Dickinson, San Jose, CA, USA) connected to a pressure transducer (MPA-V, Second Military Medical University, Shanghai, China) was inserted into the right common carotid. The mean arterial pressure (MAP) and heart rate (HR) were monitored using a lead II ECG. Thereafter, blood samples were withdrawn and the animals were killed. Collected blood samples were divided into two portions, one was mixed with EDTA to separate plasma, and the other was centrifuged directly to separate serum. Two kidneys were immediately taken out, decapsulated and weighed, and then the renal cortex was separated from the kidneys. A part of the renal cortex (50 mg/rat) was boiled in 1 mL 0.1% acetic acid for 10 min and then the homogenates and the supernatant were collected for ET-1 assay. A portion of renal cortex (100 mg/rat) was homogenized in normal saline and the supernatant was collected for biochemical measurements. The samples of renal cortex, plasma, serum and homogenates were stored at -70 oC before use.
Measurement of biochemistry in plasma, serum and renal cortex Plasma and renal cortex concentrations of ET-1 were determined by a radioimmunoassay method following the instructions on the radioimmunoassay kit (Beijing Northern Bioengineering Institute, Beijing, China). The serum creatinine, blood urea nitrogen, and serum and renal cortex levels of total nitric oxide synthase (tNOS), inducible NOS (iNOS), NO, xanthine oxidase (XOD), superoxide dismutase (SOD), catalase (CAT), glutathione peroxidase (GSH-PX) and malondialdehyde (MDA) were determined by spectrophotometric method with kits (Nanjing Jiancheng Bio-engineering Company).
mRNA expressions of the renal cortex by RT-PCR The total RNA was extracted from 100 mg frozen renal cortex sample by using 1 mL Trizol. The total RNA was reverse-transcripted into cDNA by AMV reverse transcriptase. To ensure a fixed amount of initial mRNA in parallel with GAPDH, amplification was performed using the following oligo-nucleotides: sense: 5'-GCT GGG GCT CAC CTG AAG G-3'; antisense: 5'-GGA TGA CCT TGC CCA CAG CC-3', pre-denaturation at 94 oC for 5 min, denaturation at 94 oC for 40 s, annealing at 63 oC for 40s, extension at 72 oC for 1 min, 34 cycles and final extension at 72 oC for 10 min. Specific oligonucleotide primers used in this experiment were as follows (Sangon): (1) tumor necrosis factor-alpha (TNF-α)[12]: sense: 5'-TAC TGA ACT TCG GGG TGA TTG TCC-3'; antisense: 5'-CGT AGG ACC CGA TGT GAC TC-3'; (2) iNOS[13]: sense: 5'-CGG ATC CTA GGC TAA CAA GAC CCA AG-3'; antisense: 5'-ATC CTT TCT AGA AAA CCT CC-3'; (3) ECE (endothelin converting enzyme)[14]: sense: 5'-CGT AGC GAT AGT CTT AGC AC-3'; antisense: 5'-GTG CCA CAC CAA AAC TAC AG-3'; (4) PreproET-1[15]: sense: 5'-AGC AAT AGC ATC AAG GCA TC-3'; antisense: 5'-TCA GAC ACG AAC ACT CCC TA-3'; (5) ETA[16]: sense: 5’-ATC GCT GAC AAT GCT GAG AG-3'; antisense: 5'-CCA CGA TGA AAA TGG TAC AG-3';(6) ETB[16]: sense: 5'-GAA AAG AGG ATT CCC ACC TG-3'; anti-sense: 5'-ACG AAC ACG AGG CAT GAT AC-3'. PCR was performed as follows by an authorized thermal cycler (Eppendorf, Hamburg, Germany): pre-denaturation at 94 oC for 5 min; denaturation at 94 oC for 1 min; annealing at 55, 52, 54, 64, 56, 57 oC for TNF-α, iNOS, ECE, PreproET-1, ETA, and ETB, respectively, for 1 min; extension at 72 oC for 1 min for 30, 28, 28, 25, 30, 30 cycles for TNF-α, iNOS, ECE, PreproET-1, ETA and ETB, respectively; and a final extension at 72 oC for 10 min.
The amplification products were separated by agarose gel electrophoresis (1.7%), stained with ethidium-bromide, and the bands were analyzed by Labworks imaging acquisition and analysis software (Ultra-Violet Products, Cambridge, UK).
Western blot analysis The renal cortex (approximately 100 mg), which had been snap frozen in liquid nitrogen, was homogenized in lysis buffer (50 mmol/L Tris-HCl, pH 8.0, 150 mmol/L NaCl, 0.02% Sodium Azide, 0.1% SDS, PMSF 100 mg/mL, Aprotinin 1 mg/mL 1% NP-40, 0.5% sodium deoxy-cholate) using a tissue homogenizer. The lysate was analyzed for protein content using a Bradford assay (Bio-Rad, Hercules, CA, USA). Equal amounts of protein were resolved under reducing conditions on an 8% SDS-polyacrylamide gel. Immunoblotting was performed using a monoclonal antibody that specifically recognizes the IκB-binding region on the p65 subunit to detect activated NF-κB (Santa Cruz Biotechnology, Santa Cruz, CA, USA) at a dilution of 1:100 in non-fat milk: Tris buffer. The membrane was subsequently probed with a secondary anti-goat antibody conjugated to horseradish peroxidase (Santa Cruz Biotechnology) at a dilution of 1:1000 and detected with 0.1% 3,3'-diaminobenzi-dine/0.01% hydrogen peroxide. The bands were analyzed by Labworks imaging acquisition and analysis software (Cambridge, UK).
Statistical analysis Data were expressed as mean±SD. The one way ANOVA assay was performed and differences between two groups were compared by the Student-Newman-Keuls (SNK) test. The results were considered significant at P<0.05.
Results
Survival rate, MAP and HR The survival time and rate of the untreated septic shock group were 38.2±3.3 h and 9.1% (P<0.01) against zero deaths found in the sham operation group. A marked fall in MAP (-36.9%) and an increased HR (30.7%) were found in the CLP group, which showed a sustained hypotensive status due to septic shock. The treatment of CPU0213, which was instituted 8 h after the diffused infection of peritoneal cavity by the feces leakage from the punctured cecum, prolonged the survival time and rate by 53.6±4.0 h and 35.2%. It was significantly effective to raise the MAP and reduce the HR rather than untreated group (P<0.01) (Figure 2, Table 1).
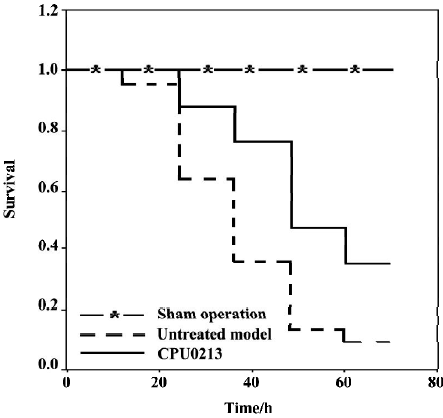
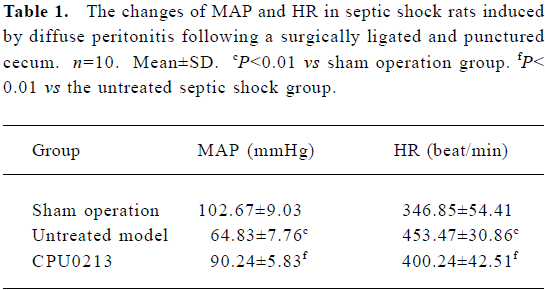
Full table
Serum creatinine, blood urea nitrogen, urinary protein, creatinine and the renal weight index Serum creatinine and blood urea nitrogen levels increased by 202.8% and 92.9%, respectively (P<0.01) in the untreated septic shock group. After administration of CPU0213, the elevated serum creatinine and blood urea nitrogen decreased significantly (P<0.01) in contrast to the untreated group (Figure 3, Table 2).
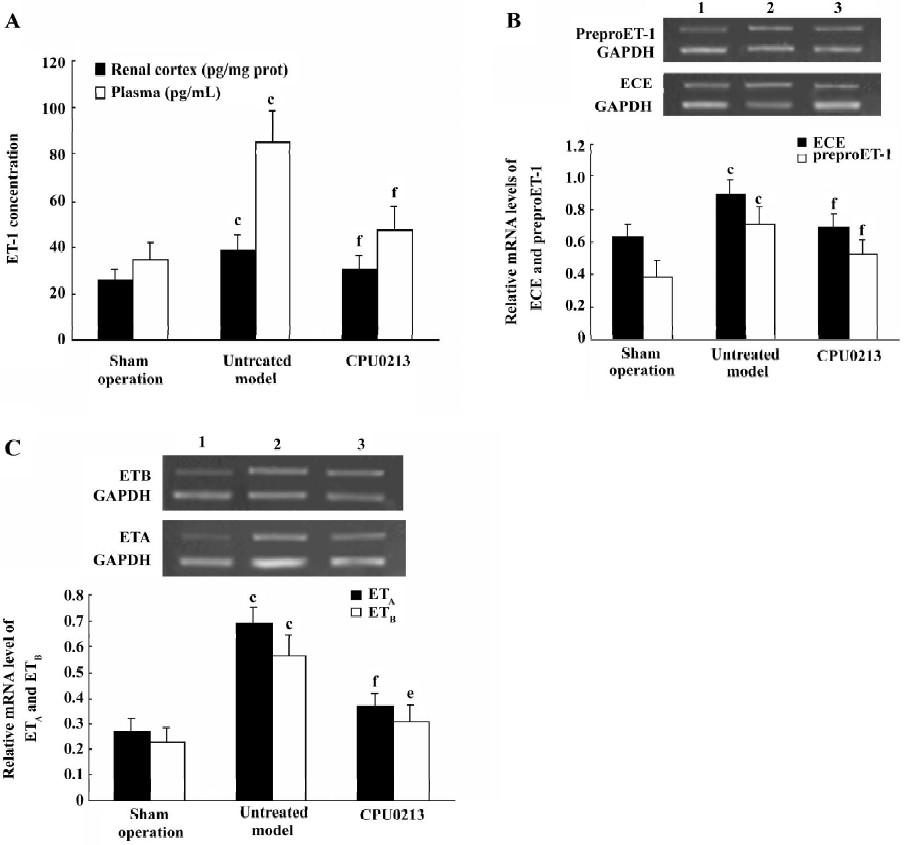
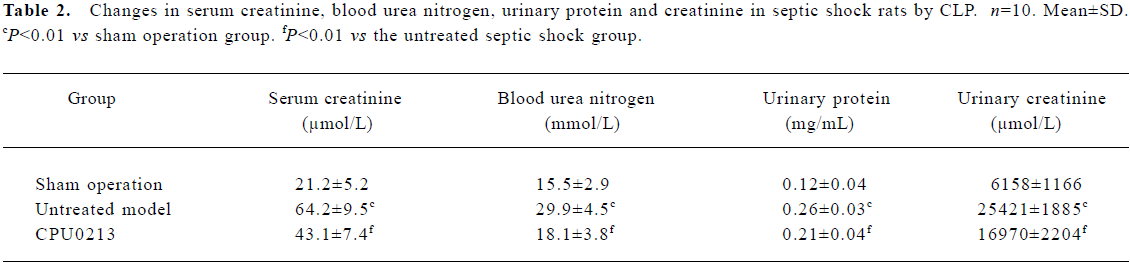
Full table
The total urinary protein and creatinine levels in urine in 24 h increased by 116.7% and 312.8%, respectively (P<0.01), in the untreated septic shock group, and reduced markedly (P<0.01) with administration of CPU0213 in contrast to the untreated group (Table 2).
The weight index of the kidney (renal weight/body weight; g/100 g) increased by 8.5% (P<0.01) in the untreated septic shock group, 0.77±0.02 versus the sham operation group 0.71±0.03; and a regression by treatment with CPU0213 was noticed down to 0.73±0.02, P<0.01 in comparison to the untreated septic shock group. The bodyweight (g) among three groups showed no changes: sham operation group, 263.9±11.5; untreated septic shock group, 257.3±12.7; and CPU0213 group, 260.9±10.1.
Activated ET pathway Plasma and renal cortex ET-1 concentrations increased significantly by 142.8% and 49.6% (P<0.01) in the untreated septic shock group, and the mRNA expression levels of ECE, preproET-1, ETA and ETB receptors were significantly upregulated in the renal cortex tissue of the septic kidney, with an increase in abundance of mRNA by 41.3%, 82.1%, 155.6%, and 143.5%, respectively (P<0.01) in contrast to the sham operation group. This indicated that an activated ET pathway was prominent in the renal cortex in the presence of septic shock. The application of CPU0213 led to dramatic regression in upregulation of the renal ET system. An elevated ET-1 in the plasma and renal cortex were significantly suppressed against the untreated group (P<0.01) and toward the control, respectively. CPU0213 was capable of reducing the upregulated mRNA expressions of the ECE, PreproET-1, ETA and ETB receptors toward the control (P<0.01) in contrast to the untreated septic shock group (Figure 3A−3C).
Oxidative status in serum and renal cortex The changes in the redox system significantly showed a status of oxidative stress in septic shock rats following CLP. In the untreated septic shock group, the XOD and MDA levels increased by 68.6% and 53.8% in serum and by 52.8% and 225.6% in the renal cortex, (P<0.01), respectively. Decreases in CAT, GSH-PX and SOD levels of -80.9%, -50.8% and -19.6% in the serum and -57.1%, -28.6%, and -19.9% in the renal cortex, respectively, were found in contrast to the sham operation group (P<0.01). CPU0213 significantly ameliorated the impaired redox system by reducing the altered parameters toward the normal (P<0.01) versus the untreated, respectively (Figure 4, Tables 3, 4).
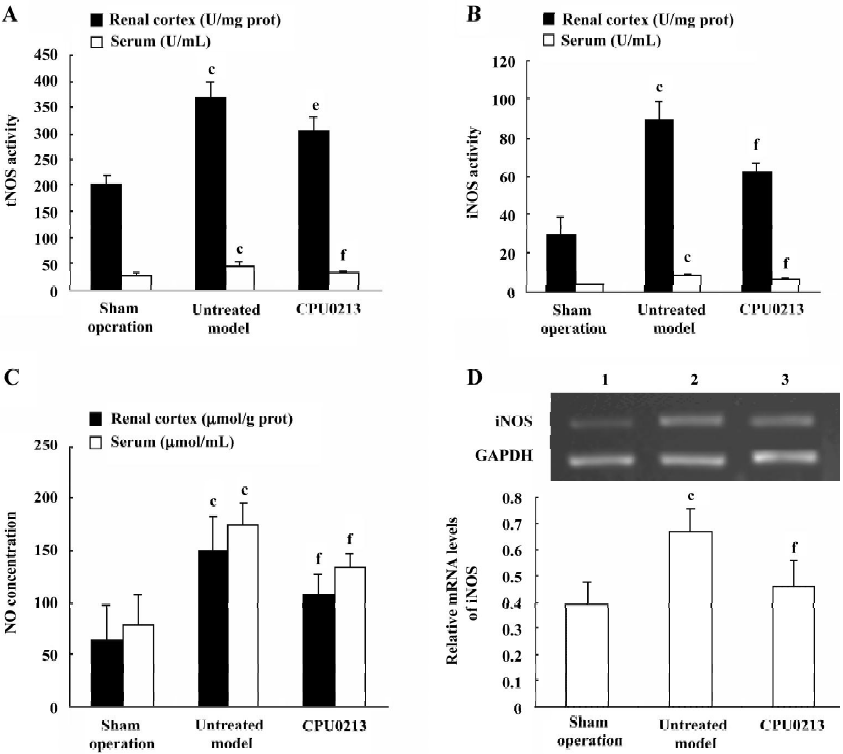
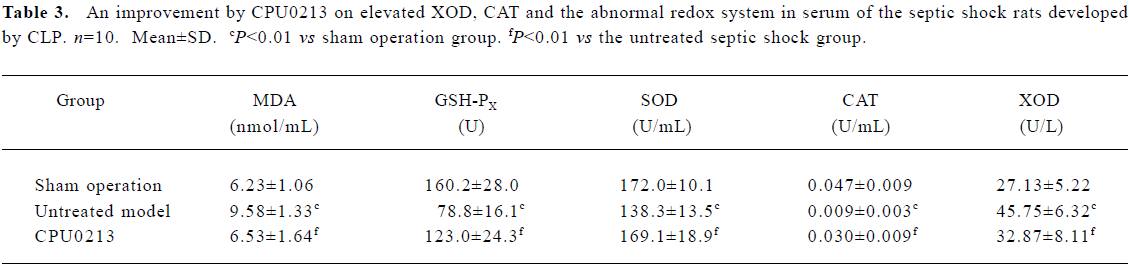
Full table
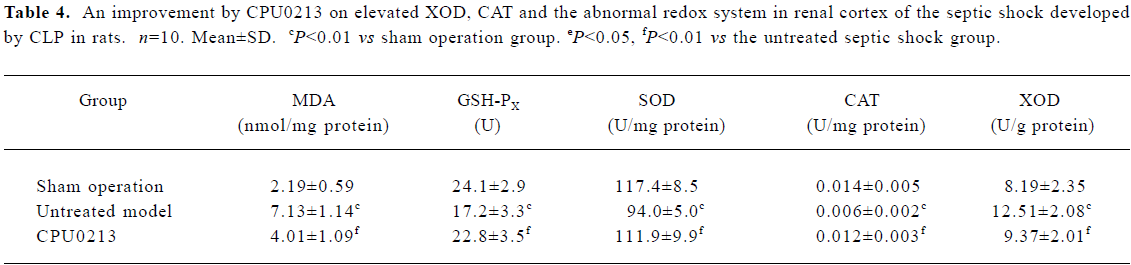
Full table
NOS in the serum and renal cortex The activity of tNOS, iNOS and NO levels in serum and renal cortex were, in the untreated septic shock group, significantly increased by 63.6%, 175.8%, and 125.4% in serum, and 83.8%, 208.0%, and 134.7% in the renal cortex (P<0.01), respectively. The mRNA levels of iNOS in renal cortex of the untreated group were significantly upregulated by 78.1% (P<0.01) versus the sham operation group. CPU0213 significantly attenuated the abnormal activity of tNOS, iNOS and NO in both serum and renal cortex (P<0.01), versus the untreated septic shock group, respectively. A decline in the mRNA expression of the iNOS in the renal cortex was also achieved by CPU0213 (Figure 4A−4D).
TNF-α and NF-κB in the renal cortex In the untreated septic shock group, the mRNA abundance of the TNF-α was significantly upregulated by 57.1% (P<0.01) versus the sham operation group, and was regressed by CPU0213 (P<0.01) versus the untreated septic shock group (Figure 5 A). The relative protein amount of activated NF-κB in renal cortex of the untreated septic shock group increased significantly by 110.0% (P<0.01) versus the sham operation group, and reduced dramatically by CPU0213 (P<0.01) versus the untreated group (Figure 5B).
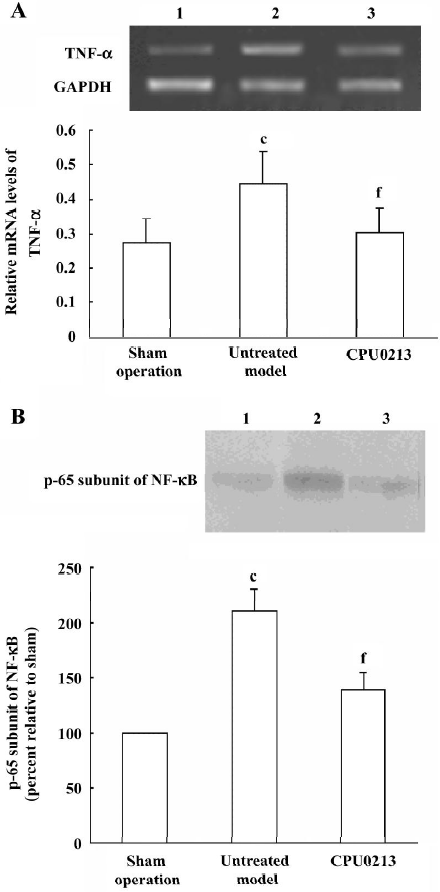
Discussion
The septic shock model of rats was well established by manifestations of a sustained hypotension, an increased HR and a high mortality in the untreated septic shock group following the CLP. The acute compromised renal function was manifested by an increment in serum creatinine, blood urea nitrogen, 24-h urinary protein and creatinine. The excessive proteinuria was sourced from an increase in the permeability of the damaged glomerular membrane and the renal tubular cell injury in septic kidney. The significant increase of the serum and urinary creatinine reflected the development of ARF. A renal insult from inflammatory reaction in the kidney was also manifested by an increment in the renal weight index in the untreated septic shock group. These pathological changes in the kidney were attributed to the maladaptive hemodynamics and the excessive activated cytokines: TNF-α[17], iNOS[18], free radicals[19] and excessive ET-1 released in septic shock.
ET-1 is expressed in the glomerular and tubular cells and an excess of ET-1 is released from the mesangial cells in response to ischemia and toxic insult[3]. The renal cortex produces the ET-1, which is critically related to renal injury[20]. The half-life of the released ET-1 is as short as 15 min and an elevated plasma ET-1, which is more than that in the renal tissue, is sourced and multiplied by continuous and profound biosynthesis and release from other organs, such as the heart, vasculature, and lungs, which are attacked in septic shock. In the renal cortex, an increased ET-1 level in combination with an upregulation of the mRNA of preproET-1 and ECE provide an over-activity of the ET system, which is implicated in toxic insult to the renal glomerular filtration and tubular function. An over-activated ET system was also manifested by an upregulation of both the ETA and ETB receptors, indicating that there is a co-contribution of the ETA and the ETB receptors to the renal abnormalities in response to septic shock. The findings of the ETB receptors involved in the septic kidney is in keeping with the facts that the ETB constricts blood vessels in pathological conditions and the suppression of the activated ETB in breast cancer provides more efficacies to paclitaxel[21], and both the ETA and ETB are involved in the failing rat heart. So an activation of the ETB takes an active part in the insult to the kidney in septic shock and the benefit is likely sourced from the dual blockade of the ETA and ETB by CPU0213.
An increase in the blood pressure in the response to CPU0213 improves the renal perfusion to bring about regression of the abnormality. An endothelin receptor antagonist had been proved to be more beneficial against the angiotensin receptor type 1 (AT1) blocker to improve ischemia-induced acute renal failure and a comparison was made between bosentan and losartan[22]. Furthermore, a combination of losartan with bosentan did not produce a relief comparable to bosentan alone because of a hypotensive response that is harmful to the diseased kidney developed by the AT1 blocking effect.
A considerable number of recent studies suggest that oxidant stress plays a major role in the progression of ARF[23]. When the endogenous antioxidant reserve is compromised the renal glomeruli and tubules are predisposed to damage following an oxidative stress episode. Under stimulation by the ROS, an excessive release of ET-1 is anticipated and the ROS is actually a component of the down-stream events of the activated ET signaling pathway[24]. An increase in ROS production is also subsequent to exaggerated ET-1 production[25]. Suppression on the ET receptors is followed by substantial inhibition on ROS production leading to a dramatic attenuation in the balance between the enzymatic activities of the redox system. The activation of the ET receptors induces an exacerbated iNOS mRNA expression and activity. A copious amount of NO, which is produced from an activated iNOS, belongs to the non-physiological component as a consequence to inflammatory reaction of the septic shock [15]. An increased non-physiological NO offers an opportunity to form a toxic peroxynitrite ONOO·-, which exaggerates the status of oxidative stress in the renal tissue. An activation of the iNOS contributes significantly to the deleterious effects of endotoxin in producing hypotension, renal injury and dysfunction and cardiac depression[16–18]. Free radicals stimulating cytokine expression and formation promote apoptotic cell death in the failing renal cells[21]. These abnormalities can be subsided dramatically by a dual endothelin receptor antagonist, CPU0213.
The transcription processes in the nucleus are accelerated under the septic conditions, which result from several mechanisms. An excessive ROS can stimulate the transcription in the nuclear to upregulate the mRNA abundance of the ET system and iNOS, in accordance with previously published reports[5,22]. The NF-κB, a post-transcription regulating factor, is retained in cytoplasm as an inactivated form in quiescent cells in association with its inhibitor, the inhibitory κB (IκB), which masks the nuclear localization sequence of NF-κB. In septic shock, in response to bacterial endotoxin and ROS, the IκB is phosphorylated by IκB kinase (IKK) and detached from NF-κB allowing NF-κB to translocate into the nucleus where it binds to target genes to promote transcription. The TNF-α is actively implicated in acute renal failure by endotoxemia[26]. There is an interaction among the TNF-α, NF-κB, and ET system[27]. It is indicated that an interlink between the ET-1, NF-κB and TNF-α is tight[28] and an enhanced ET-signaling pathway frequently activates the NF-κB, which in turn upregulates transcription and post-transcription of bioactive substances in the presence of the enhanced ET pathway. This occurs in renal insult by septic shock. Activation of the ET system and upregulation of NF-κB and TNF-α are also closely linked with ROS in the pathological progression involving the MAPK pathway[29].
Recovery of upregulation of NF-κB protein and TNF-α, preproET-1 and ECE mRNA are achieved following the ETA and ETB blockade by CPU0213, consequently ameliorating the ARF in septic shock. Remarkably, even the medication was delayed as long as 8 h after septic infection, the effect was still significant. It indicated that CPU0213 was potent to attenuate the abnormalities in ARF in septic shock.
In conclusion, the upregulated ETA and ETB receptors are involved in the activated ET system, which is linked with upregulated NF-κB, TNF-α, iNOS and oxidative stress in the pathological process of ARF in septic shock. The application of an endothelin receptor antagonist, CPU0213, is effective and rational due to its dual blockage of the two upregulated ETA and ETB receptors.
Acknowledgment
We are grateful to Prof Min JI who originally synthesized the novel compound and Mr Wei YUAN for his assistance in this experiment.
References
- Schrier RW, Wang W. Acute renal failure and sepsis. N Engl J Med 2004;351:159-69.
- Schramm L, Weierich T, Heldbreder E, Zimmermann J, Netzer KO, Wanner C. Endotoxin-induced acute renal failure in rats: effects of L-arginine and nitric oxide synthase inhibition on renal function. J Nephrol 2005;18:374-81.
- Sorrkin A, Kohan DE. Physiology and pathology of endothelin-1 in renal mesangium. Am J Physiol Renal Physiol 2003;285:F579-98.
- Iskit AB, Guc O. Effect of endothelin and nitric oxide on organ injury, mesenteric ischemia, and survival in experimental model of septic shock. Acta Pharmacol Sin 2003;24:953-7.
- Wanecek M, Weitzberg E, Rudehill A, Oldner A. The endothelin system in septic and endotoxin shock. Eur J Pharmacol 2000;27:1-15.
- Krivoruchko IA, Boiko VV, Gusak IV, Andreeshchev SA. The role of endothelin-1 in the pathogenesis of septic shock in the abdominal sepsis. Klin Khir 2004;1:30-3.
- Ishimaru S, Shichiri M, Minehita S, Hirata Y. Role of endothelin-1/endothelin receptor system in endotoxic shock rats. Hypertens Res 2001;24:119-26.
- Dai DZ, Ji M, Huang M. Activity of endothelin receptor antagonist and selective blocking-effects of compound CPU0213 on ETA and ETB. J China Pharm Univ 2004;35:552-7. Chinese..
- Liu Q, Ji M, Dai DZ. Matrix metalloproteinases changes in rat hypertrophic myocardium induced by levothyroxine and the effect of a new endothelin receptor antagonist CPU0213. J China Pharm Univ 2004;35:150-5. Chinese..
- Liu Q, Dai DZ. Influence of a new endothelin receptor antagonist CPU20213 on expression of mRNA of MMPs and TIMPs in hypertrophic myocardium induced by L-thyroxin in rats. Chin J Clin Pharmacol Ther 2004;9:281-4. Chinese..
- Okamoto I, Abe M, Shibata K, Shimizu N, Sakata N, Katsuragi T, et al. Evaluating the role of inducible nitric oxide synthase using a novel and selective inducible nitric oxide synthase inhibitor in septic lung injury produced by cecal ligation and puncture. Am J Resp Crit Care Med 2000;162:716-22.
- Liu LM, Dubick MA. Hemorrhagic shock-induced vascular hyporeactivity in the rat: relationship to gene expression of nitric oxide synthase, endothelin-1, and select cytokines in corresponding organs. J Surg Res 2005;125:128-36.
- Beck KF, Sterzel RB. Cloning and sequencing of the proximal promoter of the rat iNOS gene: activation of NF-κB is not sufficient for transcription of the iNOS gene in rat mesangial cells. FEBS Lett 1996;394:263-7.
- Ikeda K, Wada Y, Sanematsu H. Regulatory effect of experimental diabetes on the expression of endothelin receptor subtypes and their gene transcripts in the rat adrenal gland. J Endocrinol 2001;168:163-75.
- Barker S, Khan NQ, Wood EG. Effect of an antisense oligodeoxy-nucleotide to endothelin-converting enzyme-1c (ECE-1c) on ECE-1c mRNA, ECE-1 protein and endothelin-1 synthesis in bovine pulmonary artery smooth muscle cells. Mol Pharmacol 2001;59:163-9.
- Kobayashi T, Myauchi T, Sakai S. Expression of endothelin-1, ETA and ETB receptors, and ECE and distribution of endothelin-1 in failing rat heart. Am J Physiol 1999;276:H1197-H206.
- Cunningham P, Dyanov HM, Park P, Wang J, Newell KA, Qui RJ. Acute renal failure in endotoxemia is caused by TNF acting directly on TNF receptor-1 in kidney. J Immunol 2002;168:5817-23.
- Cohen RI, Hassell AM, Marzouk K. Renal effects of nitric oxide in endotoxemia. Am J Respir Crit Care Med 2001;164:1890-5.
- Salvemini D, Cuzzocrea S. Oxidative stress in septic shock and disseminated intravascular coagulation. Free Radic Biol Med 2002;339:1173-85.
- Kato A, Hishida A. Amelioration of post-ischaemic renal injury- by contralateral uninephrectomy: a role of endothelin-1. Nephrol Dial Transplant 2001;16:1570-6.
- Rajeshkumar NV, Rai A, Gulati A. Endothelin B receptor agonist, IRL 1620, enhances the anti-tumor efficacy of paclitaxel in breast tumor rats. Breast Cancer Res Treat 2005;94:237-47.
- Jerkic M, Miloradovic Z, Jovovic D, Mihailovic-Stanojevic N, Elena JVR, Nastic-Miric D. Relative roles of endothelin-1 and angiotensin II in experimental post-ischemic acute renal failure. Nephrol Dial Transplant 2004;19:83-94.
- Wang W, Jittikanont S, Falk SA, Li P, Feng L, Gengaro PE. Interaction among nitric oxide, reactive oxygen species, and antioxidants during endotoxemia-related acute renal failure. Am J Physiol Renal Physiol 2003;284:F532-F7.
- Laplante MA, Wu R, Moreau P, De Champlain J. Endothelin mediates superoxide production in angiotensin II-induced hypertension in rats. Free Radic Biol Med 2005;38:589-96.
- Kahler J, Mendel S, Weckmuller J, Orzechowski HD, Mittmann C, Koster R, et al. Oxidative stress increases synthesis of big endothelin-1 by activation of the endothelin-1 promoter. J Mol Cell Cardiol 2000;32:1429-37.
- Cunningham P, Dyanov HM, Park P, Wang J, Newell KA, Qui RJ. Acute renal failure in endotoxemia is caused by TNF activating directly on TNF receptor-1 in kidney. J Immunol 2002;168:5817-23.
- Chang J, Zhang C, Tani-Ishii N, Shi S, Wang CY. NF-kappaB activation in human dental pulp stem cells by TNF and LPS. J Dent Res 2005;84:994-8.
- Lee SB, Schorey JS. Activation and mitogen-activated protein kinase regulation of transcription factors Ets and NF-kappaB in mycobacterium-infected macrophages and role of these factors in tumor necrosis factor alpha and nitric oxide synthase 2 promoter function. Infect Immun 2005;73:6499-507.
- Zhang GX, Kimura S, Nishiyama A, Shokoji T, Rahman M, Yao L. Cardiac oxidative stress in acute and chronic isoproterenol-infused rats. Cardiovasc Res 2005;65:230-8.