Protective effects of curcumin on methylglyoxal-induced oxidative DNA damage and cell injury in human mononuclear cells
Introduction
The reactive dicarbonyl compound methylglyoxal (MG) is a metabolic byproduct of glycolysis and is also formed in various foodstuffs during processing. MG is often found at high levels in the blood of diabetic patients, where it covalently depletes glutathione (GSH), leading to serious toxicological effects. In comparison to the parent sugar, MG is more likely to cross-link with the amino groups of various proteins, forming stable end products called advanced glycation end products (AGEs)[1,2]. In addition, MG may modify cross-linked lysine and arginine residues, leading to alterations in the protein characteristics[3]. The reaction of proteins with MG has been shown to produce reactive oxygen species(ROS) such as O2– during the glycation reaction[4]; ROS and oxidative stress can damage many biological molecules (eg, DNA) and cause various cell injuries. Studies have shown that oxidative stress and AGE formation are associated with impaired cognitive processes in diabetic patients[5,6], suggesting that this may be linked to MG toxicity.
Stressful conditions such as oxidative damage may affect cell-cell or cell-extracellular matrix (ECM) interactions. Cell-ECM interactions play important roles in many cellular events, including immunity and regulation of gene expression[7,8]. One type of cell-cell interaction is the adherence and migration of leukocytes to blood vessel endothelial cells, which plays an important role for acute inflammation during infection[8]. Our previously published study showed that the hyperosmotic shock effects induced by high glucose inhibit the binding of leukemia K562 cells to collagen I, which is reversed in the presence of ascorbic acid (a well-known antioxidant)[9]. This result implies that ROS could induce the cell-ECM attachment change. However, the precise molecular mechanism of ROS and cell-ECM interaction is unclear and needs further investigation.
Curcumin, the yellow pigmented fraction of Curcuma longa, has cosmetic effects and shows efficacy in promoting wound healing as well as treating liver ailments, urinary tract diseases and hepatitis[10]. Pharmacologically, curcumin exhibits a wide range of effects including anti-inflammatory, anti-carcinogenic, hypocholesterolemic and anti-infection activities[11–13]. The potent antioxidant effects of curcumin mediate anti-proliferative and anti-carcinogenic actions in a wide variety of cell lines and animal models[14–16], and recent studies have shown that the anti-tumor activity of curcumin is a result of its ability to induce apoptosis via caspase-3 activation[17]. However, although multiple biological functions have been described for curcumin, the precise molecular mechanisms underlying its actions are currently unknown.
MG, a metabolic byproduct of glycolysis, is found at high levels in the blood of diabetic patients. Several clinical studies have shown that diabetic patients have a higher risk of infection, which is attributed to their lower immune function[18]. In this study, we used an in vitro model to investigate the mechanism by which MG/lysine glycation is toxic to leukocytes and, therefore, determine whether the cytotoxicity of MG may be one of the principal causes of immune system dysfunction in diabetic patients. We also sought to determine whether curcumin protects mononuclear cells from MG-induced injury. We show here that curcumin can block MG-induced DNA damage and ROS production in vitro and that it inhibits MG-induced ROS generation, apoptosis, and loss of attachment in human mononuclear cells.
Materials and methods
Materials Curcumin, MG, L-lysine, 2-deoxy-D-ribose, thiobarbituric acid, cytochrome c, NAC, histopaque 1077, propidium iodide, and Hoechst 33342 were purchased from Sigma (St Louis, MO, USA). pCMV plasmid DNA was isolated from Escherichia coli (E coli) cultures and purified using the Qiagen Plasmid Midi Kit (Hilden, Germany).
Analysis of DNA strand breaks Purified plasmid DNA was incubated with 5 mmol/L lysine and 5 mmol/L MG in the presence of Cu2+ at 37 °C for 3 h. To stop the reactions, samples were frozen at -70 °C. The production of DNA strand breaks as a result of reaction of lysine with MG in vitro is enhanced by Cu2+ and, in the absence of Cu2+, it is inhibited by catalase and metal chelators[19]. This suggests that MG-induced DNA breakage is caused by trace levels of transition metals. We therefore examined the ability of curcumin to inhibit MG/lysine glycation-induced DNA strand breakage and ROS generation in the presence and absence of Cu2+. Following the reaction, the plasmid DNA was resolved by 0.75% agarose gel electrophoresis, visualized by ethidium bromide staining, and photographed.
Measurement of superoxide anions in the glycation reaction Superoxide anion generation was determined by measurement of cytochrome c reduction[20]. Cytochrome c (100 µmol/L) was added to the MG and lysine reaction mixture in 10 mmol/L phosphate buffer (pH 7.4) and the reduction rate was determined as the increase in absorbance (550 nm) over 10 min at room temperature.
Measurement of hydroxyl radicals in the glycation reaction Hydroxyl radical generation was determined by measurement of thiobarbituric acid reactive 2-deoxy-D-ribose oxidation products[21]. A reaction solution containing 5 mmol/L MG, 5 mmol/L lysine and 10 mmol/L 2-deoxy-D-ribose was incubated at 37 °C for 3h in the presence or absence of 300 µmol/L Cu2+. For detection of 2-deoxy-D-ribose degradation, 200 µL of PBS, 200 µL of 2.8% (w/v) trichloroacetic acid and 200 µL of 1% (w/v) thiobarbituric acid was added, the sample was heated at 100 °C for 10 min, and the absorbance at 532 nm was measured. A reaction mixture containing MG and lysine but no 2-deoxy-D-ribose was used as the blank for comparative purposes.
Isolation of mononuclear cells and treatment with MG Human mononuclear cells were isolated from 250 mL human blood using Histopaque 1077[22]. Samples were centrifuged at 400×g for 30 min, and mononuclear cells were collected from the interface and resuspended in Tris-buffered saline (20 mmol/L Tris, 0.15 mol/L NaCl, pH 7.4). The cells were sedimented by centrifugation at 300×g for 15 min and then resuspended in RPMI. Cells were treated with various concentrations of curcumin (0–20 µmol/L) for 30 min, followed by both curcumin and 50 µmol/L MG for the indicated amount of time. Because mononuclear cells are rich in trace metal ions, the cells were not pretreated with Cu2+. After treatment, the cells were harvested and the changes in ROS generation and cell attachment were analyzed.
MTT assay Cell survival was monitored using the MTT [3-(4,5-dimethylthiazol-2-yl)-2,5-diphenyltetrazolium bromide] test. Cells were treated with various concentrations of MG followed by 100 µL of 0.45g/L MTT solution. Cells were then incubated at 37 °C for 60 min to allow color development, and then 100 µL of 20% SDS in DMF:H2O (1:1) solution was added to each well to stop the reaction. The plates were then incubated overnight at 37 °C for solubilization of the formazan products, and spectrophotometric data were measured using an ELISA reader at a wavelength of 570 nm.
Assessment of apoptosis For examination of apoptosis, cells were incubated with propidium iodide (1 µg/mL) and Hoechst 33342 (2 µg/mL) for 10 min at room temperature in the dark, and then assessed by fluorescent microscopy. In each experiment 7–10 independent fields (about 600–1000 nuclei in total) were counted per condition. Apoptotic cells were identified as propidium iodide-negative cells having condensed/fragmented nuclei visualized by Hoechst 33342 staining.
ROS assay ROS generation was measured using with 2',7'-dichlorofluorescein diacetate (DCF-DA) dye, which fluoresces in response to oxidation and can be used as an overall index of oxidative stress[23]. Cells (1.0×106) were incubated for 1 h at 37 °C in 50 µL PBS containing 20 µmol/L DCF-DA, and relative ROS units were determined using a fluorescence ELISA reader (excitation 485 nm, emission 530 nm). An aliquot of the cell suspension was lysed, the protein concentration was determined, and the results were expressed as arbitrary absorbance units per mg protein.
Analysis of attachment ability changes in mononuclear cells Mononuclear cells were treated with or without various concentrations of MG and lysine for 24 h and then allowed to adhere to collagen-coated Petri dishes (50 µg/mL) at 37 °C for 90 min in standard control medium. Cells were stained with trypan blue and the attachment was quantitatively evaluated by microscopy.
Statistics Data were analyzed by both one-way ANOVA and Student’s t-test. Data are presented as mean±SD. Differences were considered significant at P<0.05.
Results
Curcumin prevents MG/lysine-induced plasmid DNA strand breaks and ROS production Recent studies have shown that the glycation of MG by lysine in the presence of Cu2+ may lead to oxidative damage of DNA via the production of ROS[19]. As part of our search for compounds that can modulate the glycation-induced DNA damage and ROS production, we examined the effects of curcumin. In preliminary experiments, cells were pretreated with a high concentration of curcumin (200 µmol/L). We found that 200 µmol/L curcumin could effectively attenuate glycation reaction-induced DNA strand breakage both in the presence and absence of Cu2+ (Figure 1A). Cu2+ has been shown to enhance DNA damage caused by the glycation reaction in vitro[19], suggesting that curcumin is a naturally occurring compound that can potently protect DNA from damage by the glycation reaction. We further examined the dose-dependence of the effects of curcumin on glycation reaction-induced DNA damage. We found that incubation of plasmid DNA with MG and lysine-induced DNA strand breakage, as shown by decreases in the supercoiled form and increases in the nicked circular form. Preincubation with 12.5–50 µmol/L curcumin dose-dependently inhibited this DNA damage (Figure 1B). In addition, pretreatment with concentrations higher than 50 µmol/L (100–200 µmol/L) also dose-dependently decreased glycation-induced DNA damage (data not shown). Moreover, the enhancement of DNA strand breaks in the reaction of lysine with MG by Cu2+ were also inhibited by curcumin (Figure 1). Similarly, cytochrome c reduction experiments revealed that while superoxide anion formation increased time-dependently when MG and lysine were incubated together (Figure 2A), this effect was dose-dependently blocked by the addition of curcumin (Figure 2B).
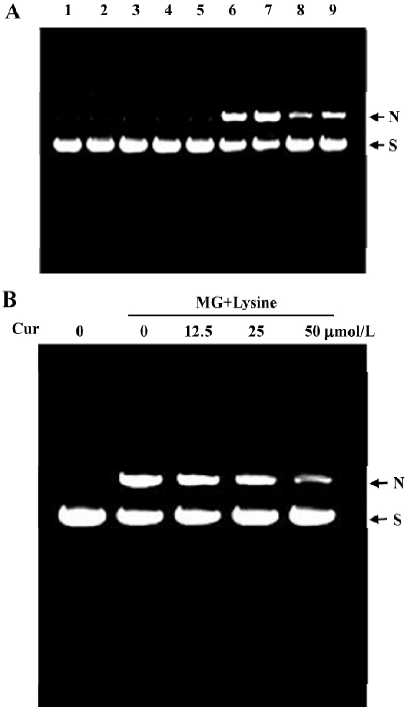
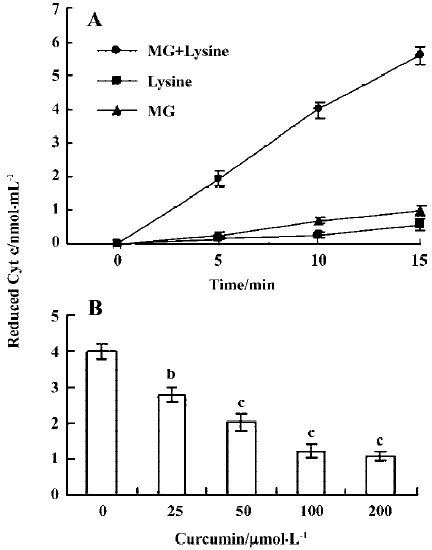
Previous studies have shown that protein glycation induces superoxide anions and subsequent H2O2 generation[24], and that the observed DNA strand breakage is induced by hydroxyl radicals produced by the reaction of H2O2 with Cu2+ [19]. We thus performed a thiobarbituric acid-reactive substance (TBARS) experiment to measure the effect of curcumin on MG glycation-induced generation of hydroxyl radicals. Our results revealed that TBARS production (about 8.6 nmol/mL) during the incubation of 2-deoxy-D-ribose with MG and lysine was dose-dependently inhibited by treatment with curcumin (Figure 3A). In addition, curcumin also significantly inhibited the generation of hydroxyl radicals in samples containing MG, lysine and Cu2+ (Figure 3B). Collectively, these results indicate that curcumin could prevent the DNA strand breaks and ROS generation by glycation of MG/lysine in vitro.
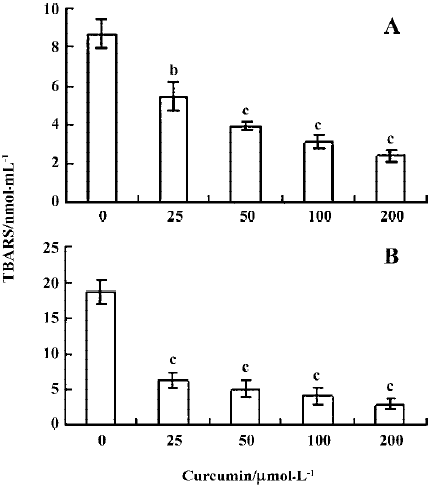
Curcumin prevents MG-induced ROS formation and apoptosis in mononuclear cells We next examined the cytotoxicity of MG and the protective effect of curcumin in mononuclear cells. Mononuclear cells were incubated without or with 2.5–20 μmol/L curcumin and then exposed to 50 µmol/L MG. Cell viability was determined by MTT assay. Curcumin alone (20 μmol/L) had no effect on cell viability (Figure 4A), but it dose-dependently inhibited MG-induced cell death (Figure 4A). A number of studies have shown that MG stimulates oxidative stress in cells, and that ROS effectively induces apoptosis[25]. Accordingly, we examined whether ROS formation occurs in MG-treated mononuclear cells, and analyzed the effects of curcumin on ROS formation using DCF-DA as the detection reagent[23]. Our results revealed that MG treatment increased the intracellular ROS content in mononuclear cells (Figure 4B). Pretreatment with curcumin significantly attenuated this increase (Figure 4B), indicating that curcumin acts as an ROS scavenger in this system. Next, we examined the effect of curcumin on MG-induced cell apoptosis. Curcumin was found to dose-dependently inhibit MG-induced apoptosis (Figure 4C). MG did not induce necrotic cell death in mononuclear cells as determined by LDH assay (data not shown). In addition, pretreatment with N-acetyl cysteine (NAC, 300 μmol/L), a frequently used ROS scavenger, reduced MG-induced increases in intracellular ROS levels and apoptosis in mononuclear cells (Figure 4B and 4C). Interestingly, under our assay conditions, curcumin appeared to be more potent than NAC at inhibiting MG-induced oxidative stress and apoptosis. These results indicate that curcumin can prevent MG-induced apoptosis by acting as a ROS scavenger. Collectively, these findings indicate that curcumin prevents MG-induced cell apoptosis by its ability to act as a ROS scavenger.
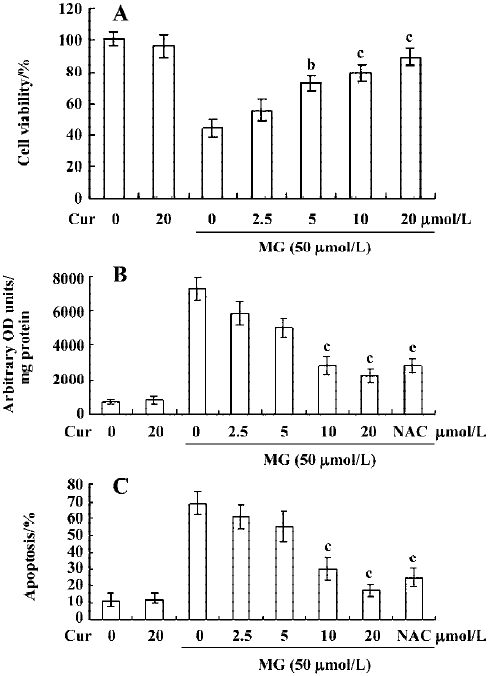
Curcumin prevents MG-induced decreases in the collagen I attachment ability of mononuclear cells The number of mononuclear cells attached to collagen I-coated Petri dishes decreased with MG treatment, but this effect was dose-dependently blocked by pretreatment of curcumin (Figure 5A). Propidium iodide and Hoechst 33342 staining revealed that the adherent cells were not apoptotic in the control and MG-treated groups; all apoptotic cells were found in the nonattached (suspended) fractions (Figure 5B). These data support the possibility that MG decreases the attachment ability of mononuclear cells by inducing apoptotic injury. To investigate the effects of curcumin and NAC (a well-known ROS scavenger) on the observed decrease in attachment ability, cells were pretreated with these compounds prior to MG treatment. Curcumin- or NAC-pretreated cells showed higher levels of attachment to collagen I than cells treated with MG alone (Figure 5C). Collectively, these results suggest that curcumin prevents MG-induced attachment changes by virtue of its ability to act as a ROS scavenger.
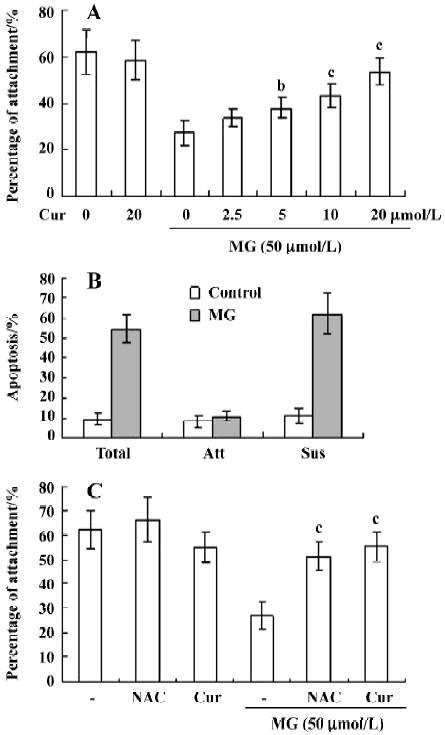
Discussion
The major MG production pathways are amino acid breakdown, glycolysis bypass, and acetone metabolism[26], and high MG levels have been associated with a number of physiological and pathological conditions, including diabetes mellitus (DM)[27]. Studies have shown that MG treatment can increase intracellular ROS levels in the following ways: (1) ROS production from the glycation reaction of MG with amino acids or proteins; (2) depletion of glutathione during MG metabolism, leading to decreased scavenging of intracellular ROS; and (3) MG-induced modification or inactivation of ROS scavenger enzymes such as superoxide dismutases, glutathione peroxidases and glutathione transferases[4,28,29]. These findings are important because oxidative DNA damage is thought to play a critical role in aging, mutagenesis, and carcinogenesis[30]. Here, we showed that MG glycation directly induces oxidative stress and free radical production in vitro and that these effects could be dose-dependently blocked by curcumin.
MG is not only produced through glycolytic metabolism, but is also found in various beverages and foodstuffs, including freshly brewed coffee and soy sauce[30]. Indeed, the consumption of 2–3 cups of coffee per day (common world-wide) can lead to a daily MG intake of up to 1 mg[31]. Normal individuals have a blood concentration of approximately 1 µmol/L MG, but the plasma MG levels in diabetics can be 3–6 times the normal amount[32], and may persist for several months or years in diabetic patients. Local tissue levels of MG can be extremely high, with concentrations up to 310 µmol/L found in Chinese hamster ovary cells[33].
Here, we showed that physiological levels of MG can induce DNA cleavage and ROS generation and decrease cellular adhesion in mononuclear cells and that curcumin dose-dependently inhibits these processes. The decreased attachment ability of MG-treated mononuclear cells (Figure 5) might be associated with the MG-induced ROS generation. Previous studies have shown that ROS attack the polyunsaturated fatty acid component in the membranes of polymorphonuclear leukocytes, leading to altered membrane compositions[34]. These alterations in attachment activity may interfere with the transendothelial migration of leukocytes, further affecting the immune and acute inflammatory responses during infection. Our results suggest that antioxidants such as NAC and curcumin actively scavenge MG-induced ROS, leading to restored attachment of the mononuclear cells to collagen I (Figure 5), and possibly leading to antioxidant-mediated decreases in the toxic effects of MG.
Recently, curcumin was reported to inhibit hyperglycemia-induced oxidative stress and diabetic cataract formation in diabetic rats[35]. Together with our current findings, this suggests that curcumin inhibits not only MG-induced ROS injury but also hyperglycemia-mediated oxidative stress and cataract formation in diabetic patients. Another study showed that curcumin irreversibly inhibits thioredoxin reductase activity by covalently modifying its active site, converting it into an NADPH oxidase that produces ROS[36]. This shift in activity means that thioredoxin reductase is converted from a component of an antioxidant system into a prooxidant. Further studies are needed to clarify these conflicting results that curcumin acts as both a ROS scavenger and producer.
In conclusion, we have shown that the natural product, curcumin, dose-dependently blocks MG glycation-induced ROS generation and apoptosis in mononuclear cells. Our results further indicate that the MG-induced blockade of mononuclear cell attachment to collagen may be abrogated by curcumin pretreatment. Because curcumin dose-dependently inhibits MG/lysine glycation-induced DNA strand breakage, ROS generation and apoptosis in mononuclear cells, we believe that it can be used to counteract damage of the immune system by MG. Future work will be required to determine the mechanisms of curcumin action and the effects of MG and curcumin on mononuclear cell immunity. However, as the passive absorption rate of curcumin in the intestine lumen is up to 80%[37], our findings suggest that it may be possible to use dietary curcumin for prevention of MG-mediated cell injury in diabetic patients.
References
- Brownlee M, Cerami A, Vlassara H. Advanced glycosylation end products in tissue and biochemical basis of diabetic complications. N Engl J Med 1998;318:1315-21.
- Bourajjaj M, Stehouwer CD, van Hinsbergh VW, Schalkwijk CG. Role of methylglyoxal adducts in the development of vascular complications in diabetes mellitus. Biochem Soc Trans 2003;31:1400-2.
- Vander Jagt DL, Robinson B, Taylor KK, Hunsaker LA. Reduction of trioses by NADPH-dependent aldo-keto reductases. Aldose reductase, methylglyoxal, and diabetic complications. J Biol Chem 1992;267:4364-9.
- Yim HS, Kang SO, Hah YC, Chock PB, Yim MB. Free radicals generated during the glycation reaction of amino acids by methylglyoxal. A model study of protein-cross-linked free radicals. J Biol Chem 1995;270:28228-33.
- Messier C, Gagnon M. Glucose regulation and cognitive functions: relation to Alzheimer’s disease and diabetes. Behav Brain Res 1996;75:1-11.
- Kyriaki G. Brain insulin: regulation, mechanisms of action and functions. Cell Mol Neurobiol 2003;23:1-25.
- Albelda SM, Buck CA. Integrins and other cell adhesion molecules. Fed Am Soc Exp Biol J 1990;4:2868-80.
- Springer TA. Traffic signals for lymphocyte recirculation and leukocyte emigration: the multistep paradigm. Cell 1994;76:301-14.
- Chan WH. Effect of resveratrol on high glucose-induced stress in human leukemia K562 cells. J Cell Biochem 2005;94:1267-79.
- Kuttan R, Bhanumathy P, Nirmala K, George MC. Potential anticancer activity of turmeric (Curcuma longa). Cancer Lett 1985;29:197-202.
- Barthelemy S, Vergnes L, Moynier M, Guyot D, Labidalle S, Bahraoui E. Curcumin and curcumin derivatives inhibit Tat-mediated transactivation of type 1 human immunodeficiency virus long terminal repeat. Res Virol 1998;149:43-52.
- Ramirez-Tortosa MC, Mesa MD, Aguilera MC, Quiles JL, Baro L, Ramirez-Tortosa CL, et al. Oral administration of a turmeric extract inhibits LDL oxidation and has hypocholesterolemic effects in rabbits with experimental atherosclerosis. Atherosclerosis 1999;147:371-8.
- Ramsewak RS, DeWitt DL, Nair MG. Cytotoxicity, antioxidant and anti-inflammatory activities of curcumins I-III from Curcuma longa. Phytomedicine 2000;7:303-8.
- Jiang MC, Yang-Yen HF, Yen JJ, Lin JK. Curcumin induces apoptosis in immortalized NIH 3T3 and malignant cancer cell lines. Nutr Cancer 1996;26:111-20.
- Jee SH, Shen SC, Tseng CR, Chiu HC, Kuo ML. Curcumin induces a p53-dependent apoptosis in human basal cell carcinoma cells. J Invest Dermatol 1998;111:656-61.
- Mahmoud NN, Carothers AM, Grunberger D, Bilinski RT, Churchill MR, Martucci C, et al. Plant phenolics decrease intestinal tumors in an animal model of familial adenomatous polyposis. Carcinogenesis 2000;21:921-7.
- Khar A, Ali M, Pardhasaradhi BVV, Begum Z, Anjum R. Antitumor activity of curcumin is mediated through the induction of apoptosis in AK-5 tumor cells. FEBS Lett 1999;445:165-8.
- Geerlings SE, Hoepelman AI. Immune dysfunction in patients with diabetes mellitus (DM). FEMS Immunol Med Microbiol 1999;26:259-65.
- Kang JH. Oxidative damage of DNA induced by methylglyoxal in vitro. Toxicol Lett 2003;145:181-7.
- Beauchamp C, Fridovich I. Superoxide dismutase: improved assays and an assay applicable to acrylamide gels. Anal Biochem 1971;44:276-87.
- Halliwell B, Gutteridge JM. Formation of thiobarbituric-acid-reactive substance from deoxyribose in the presence of iron salts: the role of superoxide and hydroxyl radicals. FEBS Lett 1981;128:347-52.
- Menon RP, Pillai S, Sudhakaran PR. Binding of collagen causes intracellular mobilization of calcium in human mononuclear cells. Biochem Mol Biol Int 1993;31:833-40.
- LeBel CP, Ischiropoulos H, Bondy SC. Evaluation of the probe 2',7'-dichlorofluorescin as an indicator of reactive oxygen species formation and oxidative stress. Chem Res Toxicol 1992;5:227-31.
- Sakurai T, Tsuchiya S. Superoxide production from nonenzymatically glycated protein. FEBS Lett 1998;236:406-10.
- Chan WH, Wu CC, Yu JS. Curcumin inhibits UV irradiation-induced oxidative stress and apoptotic biochemical changes in human epidermoid carcinoma A431 cells. J Cell Biochem 2003;90:327-38.
- Kalopos MD. Methylglyoxal toxicity in mammals. Toxicol Lett 1994;73:3-24.
- Atkins TW, Thornally PJ. Erythrocyte glyoxalase activity in genetically obese (ob/ob) and streptozotocin diabetic mice. Diabetes Res 1989;11:125-9.
- Lee C, Yim MB, Chock PB, Yim HS, Kang SO. Oxidation-reduction properties of methylglyoxal-modified protein in relation to free radical generation. J Biol Chem 1998;273:25272-8.
- Oya T, Hattori N, Mizuno Y, Miyata S, Maeda S, Osawa T, et al. Methylglyoxal modification of protein. Chemical and immunochemical characterization of methylglyoxal-arginine adducts. J Biol Chem 1999;274:18492-502.
- Ames BN. Dietary carcinogens and anticarcinogens. Oxygen radicals and degenerative diseases. Science 1983;221:1256-64.
- Kasai H, Kumeno K, Yamaizumi Z, Nishimura S, Nagao M, Fujita Y, et al. Mutagenecity of methylglyoxal in coffee. Gann 1982;73:681-3.
- McLellan AC, Thornalley PJ, Benn J, Sonksen PH. Glyoxalase system in clinical diabetes mellitus and correlation with diabetic complications. Clin Sci 1994;87:21-9.
- Chaplen FW, Fahl WE, Cameron DC. Evidence of high levels of methylglyoxal in cultured Chinese hamster ovary cells. Proc Natl Acad Sci USA 1998;95:5533-8.
- Chari SN, Muddeshwar MG. Leukocyte lipid profile in chronic alcoholics. Indian J Physiol Pharmacol 1991;35:195-8.
- Suryanarayana P, Saraswat M, Mrudula T, Krishna TP, Krishnas-wamy K, Reddy GB. Curcumin and turmeric delay stre-ptozotocin-induced diabetic cataract in rats. Invest Ophthalmol Vis Sci 2005;46:2092-9.
- Fang J, Lu J, Holmgren A. Thioredoxin reductase is irreversibly modified by curcumin: a novel molecular mechanism for its anticancer activity. J Biol Chem 2005;280:25284-90.
- Jaruga E, Bielak-Zmijewska A, Sikora E, Skierski J, Radziszewska E, Piwocka K, et al. Glutathione-independent mechanism of apoptosis inhibition by curcumin in rat thymocytes. Biochem Pharmacol 1998;56:961-5.