Comparison of antioxidant activities between salvianolic acid B and Ginkgo biloba extract (EGb 761)1
Introduction
Oxidative stress is believed to be a primary factor both in neurodegenerative diseases and in the normal process of aging[1,2]. Reactive oxygen species (ROS), such as superoxide anion (O2¯·) and hydroxyl radical (·OH), are ascertained to exert some detrimental effects, including lipid peroxidation of cell membranes, alteration of lipid-protein interactions, enzyme inactivation[3], and DNA breakage[4]. High oxygen consumption, relatively low antioxidant levels and low regenerative capacity result in brain tissue being susceptible to oxidative damage. Thus, antioxidants and free radical scavengers might have therapeutic applications in treating ROS-initiated neurodegenerative diseases such as Parkinson’s (PD) and Alzheimer’s diseases (AD).
Recently, natural antioxidants have received considerable interest owing to their potential health benefits[5]. Salvia miltiorrhiza, commonly known as Danshen, is a well-known Chinese traditional herbal medicine, which has been widely used in oriental medicine for the treatment of heart and brain diseases, hepatitis, hemorrhage, and menstrual abnormalities. Total salvianolic acids are water-soluble components extracted from salvia miltiorrhiza. They contain approximately 1% salvianolic acid A (SalA), 57% salvianolic acid B (SalB), 35% rosmarinic acid and other polyphenolic acids[6]. Clearly, SalB (Figure 1) is the greatest constituent of total salvianolic acids. Our previous study showed that SalB possesses strong antioxidant activities[7]. It was also demonstrated that SalB could ameliorate learning and memory dysfunctions induced by transient cerebral ischemia in mice[8], alleviate ischemic damage in rats[9], and prevent cytotoxicity induced by amyloid β protein (Aβ)[10]. Although the mechanism of action of SalB is not fully understood, some investigators believe that the antioxidant activity of SalB is involved in its pharmacological actions[8,9].
Ginkgo biloba is another Chinese plants widely used for its medicinal properties for several thousand years. The standardized extract of ginkgo biloba leaves, named EGb 761, contains specific percentages of ginkgo-flavone glycosides (24%) and terpenoids (6%)[11]. During the past decade, many experiments have demonstrated that EGb 761 exhibits a range of biochemical and pharmacological effects[12]. It possesses antioxidant and neuroprotective properties, improves blood rheology and tissue metabolism, opposes the detrimental effect of ischemia, attenuates apoptosis in neuronal cultures and inhibits the formation of Aβ aggregates. Recently, EGb 761 has been marketed as a therapeutic dietary supplement to counteract a variety of neurological disorders, including AD. Most of the effects of EGb 761 have been attributed to its antioxidant properties, probably because of the presence of flavonoid or terpenoid constituents in the extract[13,14].
Although salvia miltiorrhiza has been used in traditional medicine for over 1000 years, its antioxidant activity still lacks convincing evidence. Moreover, comparative evaluation of the antioxidant activities of SalB and EGb 761 has not as yet been conducted. The goal of the present study was to investigate antioxidant and free radical scavenger activity of SalB, and compare the effects of SalB with those of the well-known natural antioxidant EGb 761.
Materials and methods
Drugs and reagents SalB (purity >99%) was purchased from the National Pharmaceutical and Biological Product Inspection Institute Of China (Beijing, China), and EGb 761 was obtained as dry powders from IPSEN (Paris, France). 5,5-dimethyl-1-pyrroline-N-oxide (DMPO), 2,5-dihydroxybenzoic acid (2,5-DHBA), L-ascorbic acid, NADH, NADPH, phenazine methosulate (PMS), and 3-(4,5-dimethylthiazol-2-yl)-2,5-diphenyl tetrazolium bromide (MTT) was obtained from Sigma Chemical (St Louis, MO, USA). High performance liquid chromatography (HPLC) grade acetonitrile were obtained from Merck (Darmstadt, Germany). Other reagents made in China were of analytical grade. SalB and EGb 761 were freshly dissolved in sterilized double deionized water and filtered through a 0.22-µm filter (Millipore, CA, USA) before each experiment.
In our pilot studies, increasing concentrations of SalB (0.00072, 0.0072, 0.072, 0.72, and 7.2 mg/L) and EGb 761 (1, 5, 10, 50, and 100 mg/L) were studied in scavenging ROS and inhibiting lipid peroxidation in order to select the effective doses for further use in experimental studies.
Superoxide anion assay Two sources were used for the production of the superoxide free radical. The xanthine/xanthine oxidase system[15]: superoxide anion was detected by spectrophotometry with a commercial kit (Nanjing Jiancheng Bioengineering Institute, Nanjing, China) according to the manufacturer’s instructions. O2¯· was generated enzymatically using the xanthine/xanthine oxidase system in the absence and presence of different concentrations of SalB or EGb 761. Then the yield of O2¯· could reduce Nitro Blue Tetrazolium (NBT), becoming a colored substance. After reaction, each sample was detected and the absorbance was read at a wavelength of 550 nm using a SpectraMax microplate reader (Molecular Devices, Sunnyvale, CA, USA). Prior to performing the study, we examined whether these two plant extracts inhibited xanthine oxidase activity by monitoring uric acid formation spectrophotometrically at 290 nm[16]. The PMS/NADH system: superoxide radical was generated in a non-enzymic system described by Nishimiki et al[17] with slight modifications. The reaction mixture contained 15 µmol/L PMS, 78 µmol/L NADH, 30 µmol/L NBT, and various concentrations of extracts in phosphate buffer (0.1 mol/L, pH 7.4). After being incubated at ambient temperature for 5 min, the color was read at 560 nm against blank.
Hydroxyl free radical assay The following two methods were used to assess the scavenging effect on hydroxyl radicals. High performance liquid chromatography with electrochemical detection (HPLC-ECD): a Fenton-type reaction (Fe2+-EDTA+H2O2 →Fe3+-EDTA+·OH+OH –) was used to test the ability of SalB and EGb 761 to scavenge ·OH, according to a previous study[18] with slight modifications. The reaction mixture contained 5 mmol/L DMPO, 500 µmol/L FeSO4, 500 µmol/L EDTA, and 2 mmol/L H2O2, in the absence and presence of the extracts in a final volume of 0.5 mL. After incubation at 37 oC for 5 min, 50 µL 2,5-DHBA solution was added at a final concentration of 0.5 µmol/L. Then 20 µL of the mixture was injected into HPLC and analyzed by using an electrochemical detector. The ·OH was measured by oxy-radical trapping of DMPO to form DMPO-OH adducts in the system. HPLC was carried out on a Spherisorb ODS-2 reversed-phase column (250 mm×4.6 mm, 5 µm), with a mobile phase of citric acid/sodium acetate (30 mmol/L citric acid-50 mmol/L sodium acetate-3% acetonitrile, pH 5.1), at a flow rate of 1.2 mL/min and detection potential of 0.6 V with Ag/AgCl as a reference electrode. The quantitation of the DMPO-OH adducts was expressed as the ratio of its peak area to that of 2,5-DHBA used as an internal standard. Spectrophotometry: hydroxyl radicals were also measured by spectrophotometry using a commercially available detection kit (Nanjing Jiancheng Bioengineering Institute) according to the manufacturer’s instructions. The kit uses a chromatogenic substrate, which reacts with the product of ·OH, yielding a stable color substance with maximum absorbance at 550 nm.
Assay of lipid peroxidation in microsomes of rat brain, liver and kidney Male Wistar rats (Grade II, Certificate N
The level of malondialdehyde (MDA), a secondary product of lipid peroxidation, is often used as an index of lipid peroxidation by reacting the lipid peroxides with thiobarbituric acid (TBA). In the present study, lipid peroxidation in microsomes prepared from brain, liver and kidney were induced by ascorbate-NADPH[20] and determined as TBA-reacting products as described in a previous study[21]. The reaction mixture, containing 1 mg of microsomal protein in 0.1 mol/L phosphate buffer (pH 7.4), 200 µmol/L NADPH, 50 µmol/L L-ascorbic acid, and various concentrations of the tested extracts, was incubated at 37 oC for 30 min (final volume=1 mL). The reaction was terminated by the addition of 2 mL of 0.53% TBA (w/v) and 20% trichloroacetic acid (TCA). This mixture was heated at 100 oC for 30 min, cooled in ice and centrifuged at room temperature for 10 min at 3000×g. The absorbance of each supernatant at 532 nm was then measured and 1,1,3,3-tetraethoxypropane (TEP) was used as a standard. The results were expressed as percent of control.
Hydrogen peroxide (H2O2)-induced oxidative damage in SH-SY5Y cells Human neuroblastoma SH-SY5Y cells were obtained from Institute of Basic Medical Sciences, Chinese Academy of Medical Sciences. The cells were cultured in DMEM with 10% FBS, 100 U/mL penicillin, 100 mg/L strepto-mycin, and maintained at 37 oC in a humidified atmosphere of 95% air and 5% CO2. All experiments were carried out 48 h after cells were seeded at a density of 1.0×105 cells/cm2. H2O2 was added into the cultures from freshly prepared stock solutions with a final concentration of 150 µmol/L. The extracts were added to cells 15 min before the treatment with H2O2. Assay for cell viability, lipid peroxidation and enzyme activity were performed 24 h after incubation with H2O2.
MTT is an indicator of the mitochondrial activity of living cells and is generally used as an index of cell survival[22]. After different treatments, 10 µL of the 5 g/L MTT stock solution was added in 96-well culture plates and maintained for 4 h. Subsequently, the medium was aspirated and 100 µL DMSO was added. The absorbance was measured at 570 nm. The SH-SY5Y cells were washed two times with PBS for harvesting, then scraped from the plates into ice cold PBS (0.1 mol/L, containing 0.05 mmol/L EDTA) and homogenized. The homogenate was centrifuged at 4 oC at 10 000×g for 30 min. The resulting supernatant was stored at -20 oC until MDA assay. The content of MDA was measured as described above. LDH, an indicator of cell injury, was detected with an assay kit (Beijing BHKT Clinical Reagent, Beijing, China). Culture medium (50 µL) was gently aspirated and saved for LDH determination. LDH could catalyze the synthesis of pyruvic acid from lactic acid, and pyruvic acid then reacted with 2,4-dinitrophenyl hydrazine to form a derivative of phenylhydrazone. The latter showed a brownish-red color in basic solution. After reaction, each sample was detected and the absorbance was read at a wavelength of 440 nm.
Statistical analysis All results are expressed as means± SD. Comparisons between groups were made using an analysis of variance (ANOVA), followed by a least significant difference multiple range test. The differences were considered significant when P<0.05.
Results
Selecting the effective doses of SalB and EGb 761 In our pilot studies, graded concentrations of SalB (0.00072, 0.0072, 0.072, 0.72, and 7.2 mg/L) and EGb 761 (1, 5, 10, 50, and 100 mg/L) were studied on ROS formation and lipid peroxidation. It was not observed that the lower doses of SalB (0.00072 and 0.0072 mg/L) and EGb 761 (1 and 5 mg/L) produced any significant effect compared to the control, although slight reductions appeared (data not shown). Therefore, in the following experiments the higher doses of SalB (0.072, 0.72, and 7.2 mg/L) and EGb 761 (10, 50, and 100 mg/L) were used to compare the antioxidant activities between them. The concentrations 0.072, 0.72, and 7.2 mg/L of SalB used in the following were equivalent to 0.1 µmol/L, 1 µmol/L, and 10 µmol/L in molarity, respectively.
Effects of SalB and EGb 761 on superoxide anion radical The ability of extracts to scavenge the superoxide anion radical was monitored in two different systems. In Figure 2, the effects of these extracts on superoxide anion radical generated in the xanthine/xanthine oxidase system was presented. Before testing the inhibitory effects of SalB and EGb 761 towards superoxide generation, we examined whether these extracts interact with xanthine oxidase. It was observed that SalB at the concentrations tested here did not exhibit a significant influence on xanthine oxidase, so the reduction in the generation of superoxide anion was the direct effect of SalB on superoxide anion. However, EGb 761 at concentrations above 50 mg/L significantly inhibited xanthine oxidase activity (data not shown), indicating the actual superoxide scavenging action of EGb 761 was lower than that shown in Figure 2. As shown in Figure 2, both of these extracts inhibited O2¯· generation in a dose-dependent manner. At the concentration of 7.2 mg/L, SalB caused 36.6% inhibition of O2¯· generation, and EGb 761 at the concentration of 10 mg/L could achieve a similar inhibitory effect. Although the inhibitory effect strengthened with an increase of the concentration of EGb 761, the result was partly associated with its interaction with xanthine oxidase.
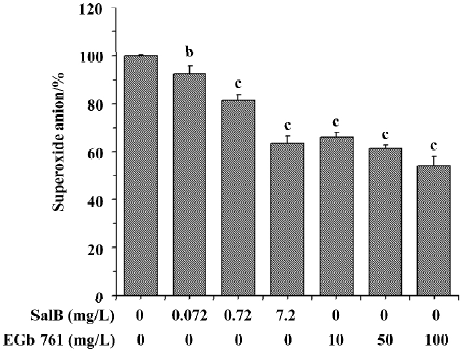
The ability of extracts to scavenge the superoxide anion radical was also assayed in the PMS/NADH system (Figure 3). It is obvious that SalB exhibited dose-related inhibitory activity towards O2¯· generation, and that the highest concentration (7.2 mg/L) tested here caused about 40% inhibition of O2¯· generation. EGb 761 inhibited the O2¯· generation by 40% at concentrations above 10 mg/L, and the inhibitory activity remained constant at higher EGb 761 concentrations, indicating that the maximal effect of EGb 761 is obtained at approximately 10 mg/L. These results obtained from the two systems indicate that both SalB and EGb 761 are capable of inhibiting O2¯· formation, and the inhibitory activity of SalB is more potent.
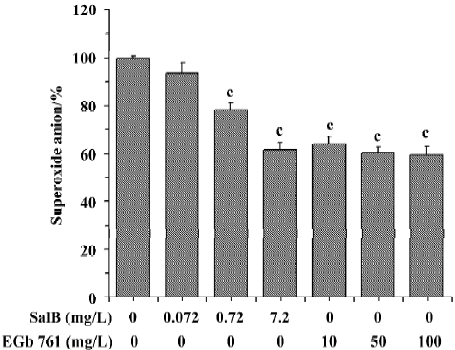
Effects of SalB and EGb 761 on hydroxyl free radical The ability of extracts to scavenge the hydroxyl free radical was evaluated by two different methods. Figure 4 shows a typical HPLC chromatograph of DMPO-OH adduct in Fe2+-EDTA-H2O2 system. In Figure 5, the effects of these extracts on hydroxyl radical monitored by HPLC-ECD was presented. All the concentrations of the two extracts significantly inhibited the formation of DMPO-OH and exhibited dose-related inhibitory activity. SalB exerted a maximal inhibitory effect of 40% at a level of 7.2 mg/L, and EGb 761 could not achieve the same inhibitory effect even at the highest concentration (100 mg/L) tested here.
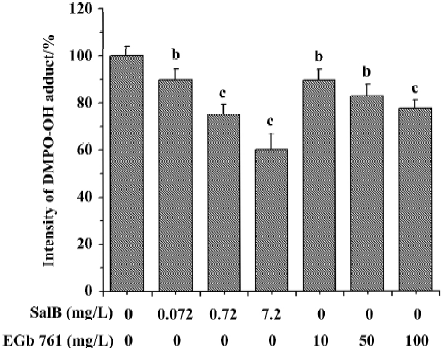
The ability of extracts to scavenge the hydroxyl radical was also assayed by spectrophotometry using a commercial detection kit. All the concentrations of the two extracts significantly inhibited ·OH generation and the inhibition profile was consistent with the results obtained by HPLC-ECD. These results obtained from the two methods indicate that both SalB and EGb 761 are capable of inhibiting ·OH forma-tion, and the inhibitory activity of SalB is much more potent (Figure 6).
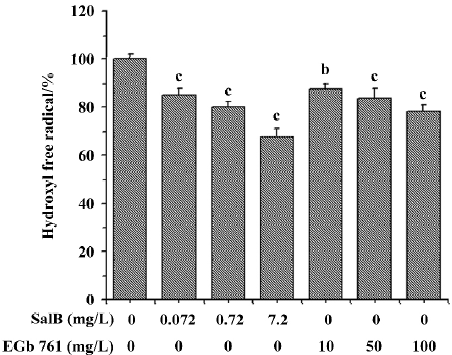
Effects of SalB and EGb 761 on lipid peroxidation in microsomes The effects of these two extracts on microsomal MDA formation are shown in Table 1. SalB and EGb 761 at all the concentrations tested here significantly decreased the formation of MDA and had a dose-dependent inhibitory activity. Consistent with the ROS scavenging activity presented above, SalB was more efficient than EGb 761 in inhibiting lipid peroxidation in microsomes.
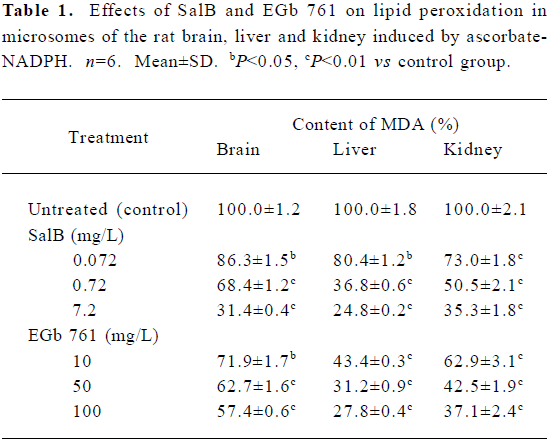
Full table
Effects of SalB and EGb 761 on viability of SH-SY5Y cells induced by H2O2 The effects of SalB and EGb 761 on the cytotoxicity induced by H2O2 in SH-SY5Y cells were evaluated in this study (Figure 7). Treatment with 150 µmol/L H2O2 for 24 h resulted in a significant inhibition of MTT reduction (the cell viability decreased to 65.2% with respect to untreated cells). However, pre-treatment of cells with SalB (0.072–7.2 mg/L) or EGb 761 (10–100 mg/L) concentration-dependently protected cells against the toxicity induced by H2O2. The lowest concentration of SalB or EGb 761 tested here did not enhance significantly viability, although a slight increase was found. In addition, the concentration of SalB was far lower than that of EGb 761 when the similar protective effect was obtained.
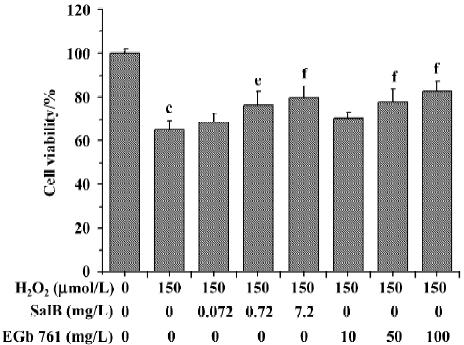
Effects of SalB and EGb 761 on the content of MDA and the activity of LDH in SH-SY5Y cells induced by H2O2 The treatment of SH-SY5Y cells with 150 µmol/L H2O2 caused an increase in the intracellular MDA level by 137.2%. However, preincubation of cells with SalB or EGb 761 markedly attenuated the increase in the MDA level in a dose-dependent manner, and SalB at a lower concentration could achieve a similar inhibitory effect (Figure 8).
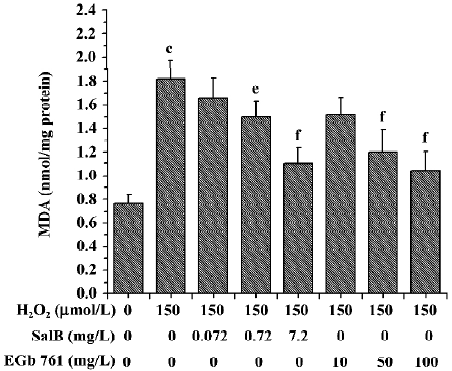
The effects of these two extracts on LDH activity induced by H2O2 are shown in Figure 9. There was a marked increase of LDH leakage after the addition of H2O2 to the culture media, and this response was attenuated by EGb 761 in a dose-dependent manner. Although SalB markedly attenuated the increase in the LDH activity only at the highest concentration, this effective concentration of SalB was far lower than that of EGb 761 when a similar inhibitory effect was obtained.
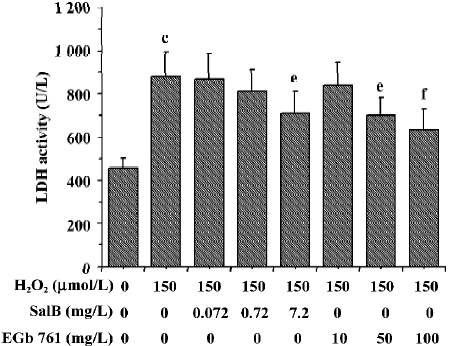
Discussion
Oxidative stress, defined as the imbalance between the formation of reactive oxygen species (ROS) and antioxidative defence mechanisms, has been suggested to be involved in the pathogenesis of most of the neurodegenerative disorders including AD[1]. Antioxidants that react preferentially with ROS to inactivate them have excited great interest because of their potential for therapeutic use. In this study, we investigated and compared the antioxidant activities of SalB and EGb 761 in aqueous solution, rat microsomes and the cellular system. Our results showed that SalB is more efficient than EGb 761 in scavenging ROS, especially hydroxyl radical (·OH). For lipid peroxidation inhibition activity in microsomes, we also obtained similar results. Furthermore, both SalB and EGb 761 protected SH-SY5Y cells against H2O2-induced oxidative damage, while the concentration of SalB was far lower than that of EGb 761 when a similar protective effect was obtained.
Superoxide anion (O2¯·) has been of intense interest owing to its increased dominance in vivo in different disease conditions. Although O2¯· is far less active than ·OH, it can generate ·OH by superoxide dismutase (SOD) in the biological system. EGb 761 has been previously reported to scavenge O2¯· generated by using the water radiolysis method, and at concentrations above 100 mg/L completely scavenge superoxide[14]. But in the present study, our data showed that EGb 761 only inhibited O2¯· formation by approximately 40% at a concentration of 100 mg/L. The great difference between them may be the result of different systems used for the production of O2¯· . Our data also showed that SalB inhibited O2¯· formation by approximately 40% at concentrations as low as 7.2 mg/L, suggesting that SalB is an efficient scavenger for O2¯· . However, EGb 761 inhibited the activity of xanthine oxidase (XOD), an important biological source of O2¯· radical, whereas SalB did not exhibit such inhibitory activity at the concentrations tested here. In addition, EGb 761 appears to have a SOD activity[13]. Whether SalB possesses enzyme activity requires further investigation.
·OH is the most active radical causing lipid oxidation and enormous biological damage. Fenton reaction (Fe2++H2O2 → Fe3+ +·OH+OH–), a well known ·OH generator, was often used to test the ability of antioxidants to scavenge this free radical. When catalysed by iron-EDTA, this reaction occurs more rapidly[23], and more importantly, EDTA is able to weaken the interference effects from some compounds that chelate or bind to iron ions. For these reason, we generated ·OH-radicals from a Fenton system containing EDTA. The radicals formed were scavenged and quantified by oxy-radical trapping of DMPO to form DMPO-OH adducts using the HPLC method. The DMPO-OH adduct peak was separated successfully and identified by HPLC-ECD. To confirm the HPLC data by an alternative method, ·OH was also measured by spectrophotometry. Our results are consistent with the previous reports that EGb 761 could scavenge ·OH-radicals[14], and demonstrate that SalB is more potent than EGb 761 in scavenging this radical, because EGb 761 even at the highest concentration (100 mg/L) could not achieve the same level of inhibition as could SalB at a level of 7.2 mg/L.
Lipid peroxidation is one of the reactions induced by oxidative stress and is especially active in those tissues having membranes rich in polyunsaturated fatty acid. The microsomal fraction has been shown to be particularly sensitive to peroxidation and as such has been used extensively to investigate the antioxidant activity of many naturally occurring and synthetic compounds[24]. EGb 761 has been reported to reduce the free radical-induced lipid peroxidation generated by NADPH-Fe3+ systems in rat microsomes, and to protect human liver microsomes from lipid peroxidation induced by cyclosporin A[25]. In the present study, we investigate the anti-lipid peroxidation effect of SalB and EGb 761 in microsomes of rat brain, liver and kidney induced by ascorbate-NADPH. The results presented here confirmed the inhibitory effect of EGb 761 on lipid peroxidation and showed that SalB was more efficient than EGb 761, which was consistent with their greater ROS scavenging ability. Therefore, the ability of these plant extracts to inhibit lipid peroxidation seems to be related to their ability of scavenging ROS.
Human neuroblastoma SH-SY5Y cells were used in this study because this is a widely used cell line for modeling neuronal characteristics. H2O2 is one of the major components of ROS which has been extensively used as an inducer of oxidative stress in an in vitro model[26]. Moreover, as a hydroxyl radical donor, H2O2 has been reported to mediate Aβ toxicity[27]. The exposure of cultured cells to free radicals results in an imbalance in energy metabolism in addition to the deleterious effects of hydroxyl and peroxyl radicals on membrane lipids and proteins. Therefore, cellular function is impaired, which finally causes cell death. Our present studies confirmed that cells treated with H2O2 caused a marked decrease in cell survival and elevation of oxidative stress characterized by accumulation of MDA production and LDH release. Lipid peroxidation may cause the damage of the cell membrane, which alters the biophysical characteristics of the cell membrane such as membrane fluidity and the conformation of protein sulphydryl groups. The release of LDH into the culture medium was regarded as an indicator of cellular damage and cytotoxicity[28]. However, when SH-SY5Y cells were preincubated with SalB or EGb 761, the H2O2-induced increase in the level of MDA and the release of LDH was remarkably attenuated as well as H2O2-induced reduction in cell survival. Our results are consistent with a previous study on EGb 761 obtained in rat cerebellar granule cells[29], and show that SalB is more efficient than EGb 761 in protecting SH-SY5Y cells against H2O2-induced oxidative damage. However, EGb 761 could upregulate the activity of SOD, the key antioxidant enzyme in the brain[30], and thus enhance the antioxidant capacity of the neuronal system. However, the influence of SalB on antioxidant enzyme activities in H2O2-treated SH-SY5Y cells remains unclear.
SalB is a water-soluble single compound isolated from the traditional Chinese drug Salvia miltiorrhiza. Our previous studies suggested that SalB has strong antioxidative activity[7]. Analysis of the molecular structure of SalB also indicated that it possesses a potent capacity for scavenging free radicals. The phenolic hydroxyl group in the structure of the SalB may be related to its antioxidant activity. The EGb 761 extract is a relatively complex mixture that contains flavonoid and terpene components. EGb 761 displays, mainly via its flavonoid constituents, free radical scavenging and antioxidant antions that could account for much of the pharmacological effects of the total extract[13,14]. Interestingly, EGb 761 is becoming one of the most popular dietary supplements in the USA for enhancing memory. In European countries it is a commonly prescribed drug for treatment of age-related disorders, including AD. Kose and Dogan[31] have shown that the antioxidant potency of EGb 761 appears to be greater than that of ascorbic acid, glutathione and uric acid, and comparable with that of lipid-soluble antioxidants such as retinol acetate and α-tocopherol. Our results presented herein demonstrated that SalB is more potent than EGb 761 in scavenging O2¯· and ·OH, inhibiting lipid peroxidation of microsomes, and protecting SH-SY5Y cells against H2O2-induced oxidative damage. Moreover, for drugs to be the effective treatment of neurodegenerative diseases they must be capable of penetrating the blood–brain barrier, and SalB can satisfy the requirement. These data suggest that there is promising potential for the natural antioxidant SalB to be used in treating neurodegenerative diseases that involve free radical and oxidative damage.
References
- Stohs SJ. The role of free radicals in toxicity and disease. J Basic Clin Physiol Pharmacol 1995;6:205-28.
- Finkel T, Holbrook NJ. Oxidants, oxidative stress and the biology of ageing. Nature 2000;408:239-47.
- Kim K, Rhee SG, Stadtman ER. Nonenzymatic cleavage of proteins by reactive oxygen species generated by dithiothreitol and iron. J Biol Chem 1985;260:15394-7.
- Imlay JA, Linn S. DNA damage and oxygen radical toxicity. Science 1988;240:1302-9.
- Block G. The data support a role for antioxidants in reducing cancer risk. Nutr Rev 1992;50:207-13.
- Li J, He LY, Song WZ. Separation and quantitative determination of seven aqueous depsides in Salvia miltiorrhiza by HPTLC scanning. Acta Pharm Sin 1993;28:543-7. Chinese..
- Li LN. Water soluble active components of Salvia miltiorrhiza and related plants. J Chin Pharm Sci 1997;6:57-64. Chinese..
- Du GH, Qiu Y, Zhang JT. Salvianolic acid B protects the memory functions against transient cerebral ischemia in mice. J Asian Nat Prod Res 2000;2:145-52.
- Chen YH, Du GH, Zhang JT. Salvianolic acid B protects brain against injuries caused by ischemia-reperfusion in rats. Acta Pharmacol Sin 2000;21:463-6.
- Tang MK, Zhang JT. Salvianolic acid B inhibits fibril formation and neurotoxicity of amyloid beta-protein in vitro. Acta Pharmacol Sin 2001;22:380-4.
- Kleijnen J, Knipschild P. Ginkgo biloba. Lancet 1992;340:1136-9.
- Smith JV, Luo Y. Studies on molecular mechanisms of Ginkgo biloba extract. Appl Microbiol Biotechnol 2004;64:465-72.
- Pincemail J, Dupuis M, Nasr C, Hans P, Haag-Berrurier M, Anton R, et al. Superoxide anion scavenging effect and superoxide dismutase activity of Ginkgo biloba extract. Experientia 1989;45:708-12.
- Marcocci L, Packer L, Droy-Lefaix MT, Sekaki A, Gardes-Albert M. Antioxidant action of Ginkgo biloba extract EGb 761. Methods Enzymol 1994;234:462-75.
- Zang LY, Stone K, Pryor WA. Detection of free radicals in aqueous extracts of cigarette tar by electron spin resonance. Free Radic Biol Med 1995;19:161-7.
- Zang LY, Cosma G, Gardner H, Vallyathan V. Scavenging of reactive oxygen species by melatonin. Biochim Biophys Acta 1998;1425:469-77.
- Nishikimi M, Appaji N, Yagi K. The occurrence of superoxide anion in the reaction of reduced phenazine methosulfate and molecular oxygen. Biochem Biophys Res Commun 1972;46:849-54.
- Liu Y, Zhang JT. Direct measurement of oxy-radicals using HPLC-ECD. Acta Pharm Sin 1993;28:416-21. Chinese..
- Gnojkowski J, Baer-Dubowska W, Klimek D, Chmiel J. Effect of toluidines on drug metabolizing enzymes in rat liver, kidney and lung. Toxicology 1984;32:335-42.
- Huang YS, Zhang JT. Antioxidative effect of three water-soluble components isolated from Salvia miltiorrhiza in vitro. Acta Pharm Sin 1992;27:96-100. Chinese..
- Ohkawa H, Ohishi N, Yagi K. Assay for lipid peroxides in animal tissues by thiobarbituric acid reaction. Anal Biochem 1979;95:351-8.
- Mattson MP, Barger SW, Begley JG, Mark RJ. Calcium, free radicals, and excitotoxic neuronal death in primary cell culture. Methods Cell Biol 1995;46:187-216.
- McCord JM, Day ED Jr. Superoxide-dependent production of hydroxyl radical catalyzed by iron-EDTA complex. FEBS Lett 1978;86:139-42.
- Wills ED. Lipid peroxide formation in microsomes. General considerations. Biochem J 1969;113:315-24.
- Barth SA, Inselmann G, Engemann R, Heidemann HT. Influences of Ginkgo biloba on cyclosporin A induced lipid peroxida-tion in human liver microsomes in comparison to vitamin E, glutathione and N-acetylcysteine. Biochem Pharmacol 1991;41:1521-6.
- Satoh T, Sakai N, Enokido Y, Uchiyama Y, Hatanaka H. Free radical-independent protection by nerve growth factor and Bcl-2 of PC12 cells from hydrogen peroxide-triggered apoptosis. J Biochem 1996;120:540-6.
- Behl C, Davis JB, Lesley R, Schubert D. Hydrogen peroxide mediates amyloid beta protein toxicity. Cell 1994;77:817-27.
- Bagchi D, Bagchi M, Hassoun EA, Stohs SJ. In vitro and in vivo generation of reactive oxygen species, DNA damage and lactate dehydrogenase leakage by selected pesticides. Toxicology 1995;104:129-40.
- Wei T, Ni Y, Hou J, Chen C, Zhao B, Xin W. Hydrogen peroxide-induced oxidative damage and apoptosis in cerebellar granule cells: protection by Ginkgo biloba extract. Pharmacol Res 2000;41:427-33.
- Seif-El-Nasr M, El-Fattah AA. Lipid peroxide, phospholipids, glutathione levels and superoxide dismutase activity in rat brain after ischaemia: effect of Ginkgo biloba extract. Pharmacol Res 1995;32:273-8.
- Kose K, Dogan P. Lipoperoxidation induced by hydrogen peroxide in human erythrocyte membranes. 2. Comparison of the antioxidant effect of Ginkgo biloba extract (EGb 761) with those of water-soluble and lipid-soluble antioxidants. J Int Med Res 1995;23:9-18.