Preparation of the phycoerythrin subunit liposome in a photodynamic experiment on liver cancer cells1
Introduction
Photodynamic therapy (PDT), the activation of a tumor-localized photosensitizer by light, is generally applied as a single modality for the treatment of a variety of solid tumors, and has attained regulatory approval[1]. Its dominant mechanism of action is the local generation of cytotoxic singlet oxygen, which causes the destruction of tumor cells and the damage of the tumor microvasculature[2]. Photofrin, a complex mixture of porphyrin oligomers, is one of the most efficient photosensitizers approved for cancer PDT[3]. However, Photofrin can cause prolonged skin photosensitization, where patients are required to avoid direct exposure to sunlight for a period of 4–6 weeks.
Phycoerythrin is a pigment protein that captures light, and is apparent in blue, red, and latent alga, and a few shell alga[4]. Phycoerythrin R-PE is a multipolymer (α β)6γ, which is comprised of α β γ subunits. The molecular weights of the subunits are 18 200 (α), 19 050 (β), and 33 800 (γ). The absorption peak is located at 550 nm, (498 and 550 nm), and 498 nm[3]. Among them, the β subunit has better PDT effects, a higher R-PE content, and weak phototoxicity, making it an attractive option for improving the selectivity of photosensitizers because of its good fluorescence characteristics (2 PEB, 1 PUB)[5].
The R-PE β subunit is hydrophobic and tends to aggregate in the aqueous environment, which limits its delivery and photosensitizing efficiency. The liposomal delivery of photosensitizers will often overcome or decrease these problems. In addition, liposomal formulations of photosensitizing agents may help to achieve better selectivity for tumor tissues compared with normal tissues. Over the past years, liposomal photosensitizers have emerged as therapeutic agents in many experimental studies, and have obtained approval for clinical application. The delivery of photosensitizing agents in liposomes may serve many functions, including solubilizing hydrophobic photosensitizers, maintaining drugs in a monomeric state for systemic (intravenous) administration and improving photochemical properties, enhancing the delivery of the photosensitizing agents in absolute terms by increased circulation times (in some cases, improving uptake), and increasing tumor selectivity[6–8].
The objective of this investigation was to establish a preparation method for the phycoerythrin β-subunit (PE-sub) liposome, improve its cellular accumulation and provide evidence for PDT effect of the PE-sub liposome on liver normal cells and cancer cells in vitro, and conduct a morphological analysis on PDT-treated cells.
Materials and methods
Polysiphona urcculate was supplied by the Institute of Oceanology, Chinese Academy of Science, Qingdao, China. The normal liver cell line HL7702 and liver cancer cell line HepG2 were obtained from the Institute of Cell Biology, Shanghai, China. Phosphatidylcholine was purchased from Sigma (St Louis, MO, USA), and cholesterin was purchased from Amresco (Solon, OH, USA).
HPLC was from Aglient (Santa Clara, CA, USA). The chromatogram column was a Dikma Diamonsil C18 (Beijing, China); the transmission electronic microscope was a Jem-100sx from Japan electron (Japan) the fluorescence microscope was from Olympus (Tokyo, Japan), the Zetasizer 3000 HSa instrument was from Malvern Instrument (Worcestershire, UK), and the UV/visible spectrophotometer was from Amersham Pharmacia Biotechnology (Uppsala, Sweden).
Separation of the PE-sub[5,9] The fresh sea red alga, Polysiphona urcculata, was rinsed in cold tap water, picked to remove contaminating algae and non-plant material, and filtered through cheesecloth. The extract was digested for 10 d by natural protease at 4 ºC in a moist atmosphere. The digested extract was centrifuged at 10 000×g for 5 min, and the supernatant was brought to 60% saturation by the addition of solid (NH4)2SO4 at 4 ºC. The (NH4)2SO4 precipitate was collected by centrifugation at 10 000×g for 10 min and dialyzed overnight at 4 ºC against 10 mmol/L NaPi buffer (pH 3.0). The protein suspension was chromatographed at 10 mL/h on a 3×30 cm CM-Sephedex C50 column in 20 mmol/L NaPi and 1 mmol/L NaCl buffer. The column was immediately developed with a linear gradient of pH 3–7.0 in the same buffer. The leading fraction which contained the γ subunit, and the later fractions containing the α and β subunits, were pooled separately. The α/β-subunit mixture was resolved into a pure subunit by chromatography on an IRC-50 column. The subunits were desalted by chromatography on a Sephedex G25 column and stored at –20 ºC. The α, β, γ subunits were characterized according to spectroscopic properties, molecular weight and behavior on SDS–PAGE.
Preparation for the PE-sub liposome The PE-sub-encapsulated liposome was prepared by the film dispersion method. Briefly, this particle was synthesized by mixing an aqueous solution containing phosphatidylcholine and cholesterin. First, we dissolved phosphatidylcholine and cholesterol in ethanol and decompressed evaporation at 35 ºC to remove the ethanol, and enable a symmetrical film to form. We then added the PE-sub to phosphate-buffered solution (PBS; pH 6.8) and sonicated the mixture for 5–20 min. The suspended clear solution was centrifuged slowly; the pellet was discarded and filtered through 0.45 µm filters. Finally, 1% mannitol was added, frozen to a dry powder, and stored at 4 ºC.
Four factors were selected: (1) the PBS buffer volume; (2) the ratio of the PE-sub to the liposome particles; (3) the ratio of phosphatidylcholine to cholesterin; and (4) the sonication time. Orthogonal table L9 (34) was used for the combination experiment.
Morphological observation on the PE-sub liposome The PBS solution of the PE-sub liposome was placed onto a glass slide and monitored under a fluorescence microscope. The excitation wavelength was 500–525 nm. The PE-sub liposome was then stained with 1% phosphotungstic acid and analyzed with a transmission electronic microscope.
Particle size and granular distribution The PE-sub liposomes were diluted with PBS buffer until a suitable concentration was obtained. Its particle size and granular distribution were determined by using the Zetasizer instrument.
HPLC analysis of encapsulation rate[10–12]
Chromatogram condition Chromatogram condition included chromatogram column: C18 column (46 mm×10 mm, 5 μm): C18 reversed phase chromatogram column (46 mm×250 mm, 5 μm); mobile phase: 0.15 mol/L PBS buffer (pH6.8): Acetonitrile (40:60); velocity of flow: 1.0 mL/min; column temperature: 30 ºC; injection volume: 20 μL; detection wavelength: 280 nm.
Sample preparation The control sample was diluted by PBS buffer at concentrations of 0.05, 0.08, 0.1, 0.2, and 0.5 g/L, The standard curve equation was made by linear regression, according to peak area to concentration. The PE-Sub liposome was separated by CM Sephedex-C50; PBS was used as eluting buffer; velocity of flow reached 1 mL/min at room temperature. PE-sub and PE-sub liposome were collected separately.
Encapsulation rate calculation The peak area of the test sample was measured by HPLC and calculated by the following equation:
Encapsulation rate: %=(total amount of the PE-sub–amount of the dissolved PE-sub)/total amount of the PE-sub×100% (1)
Precision experiment The peak area of the control sample solution was measured by HPLC and repeated 5 times.
Repetition experiment We took five test samples and measured their peak area by HPLC.
Stability experiment The control sample solution was taken to measure peak area of the samples at room temperature at 2, 4, 6, 8, 10, and 12 h.
Sample reclaiming rate experiment The PE-sub control sample was diluted at concentrations of 0.01, 0.015, 0.02, 0.025, and 0.03 g/L and injected to measure its peak area by HPLC to calculate its reclaiming rate.
Cell uptake study For monitoring cellular uptake and the subcellular localization of the liposome particles, human liver cell line HL7702 and liver cancer cell line HepG2 were routinely grown in Dulbecco’s modified Eagle’s medium (DMEM) supplemented with 10% fetal bovine serum and 1% penicillin/streptomycin and kept in an atmosphere of 95% air and 5% CO2 in a 37 ºC humidified incubator. In total, 1×105 /mL cells were inoculated in 6-well culture flash with coverslips and grown overnight. The coverslips with the cells were gently washed with serum-free DMEM, and then the PE-sub and PE-sub liposome PBS solution were added to the cells at a final concentration of 100 μg/mL. After washing twice, the cells were monitored under an Olympus fluorescent microscope after 1–6 h.
The PE-sub cell number (N) and the total cell number (S) in 10 visual units per coverslip were counted. Data presented represent the percentage of living cells (±SD) from 3 separate experiments and calculated based on the following equation:
Transfection rate=([N1+N2+N3+N4]/[S1+S2+S3+S4]) ×100% (2)
PDT In total, 5×105 /mL cells were inoculated in serum-free DMEM and treated with different concentration of the PE-sub with or without liposomes for 4 h before laser irradiation. The PDT excitation wavelength was 496 nm laser light, and the cells were cultured in DMEM containing 10% serum for 18 h. The survival rate of the photodynamic therapy-treated HL7702 and HepG2 cell lines was analyzed by 3-(4,5-dimethylthiazol-2-yl)-2,5-diphenyltetrazolium bromide assay.
Cell apoptotic analysis The photodynamic therapy-treated cells were harvested at 18 h, washed with cold PBS, and resuspended in 70% cold ethanol overnight at 4 ºC. The cells were centrifuged at 1000×g for 5 min. The pellet was washed twice in cold 0.1% Triton X-100 PBS incubated with 200 μg/mL RNase A and 20 μg/mL propidium iodide at 25 ºC for 30 min and analyzed by flow cytometry. The extent of apoptosis was quantified by using WinMDI version 2.9 (The Scripps Research Institute, La Jolla, CA, USA). For the morphological observation of the photodynamic therapy-treated cells, a drop of 0.01% acridine orange (10 μL/mL in PBS) was added to the coverslips and immediately examined under a fluorescence microscope. Fluorescence was detected at 500–525 nm. DNA in the cells was stained bright green, and RNA was stained orange.
Statistical analysis The data are the mean values of at least 3 experiments and are expressed as mean±SD. The Student’s t-test was used to compare data. P<0.05 was considered to be statistically significant.
Results
Identification of the PE-sub The PE-sub was separated and characterized on SDS–PAGE and native-PAGE. Compared with the low molecular weight marker on SDS–PAGE electrophoresis, the molecular weight of the β subunit was 19 kDa, and the absorption spectra was located at 498–550 nm (Figure 1), identifying it as the β subunit[5]. The purity of the protein was examined by absorption spectra (Amax/A280>4.0) and electrophoresis[13].
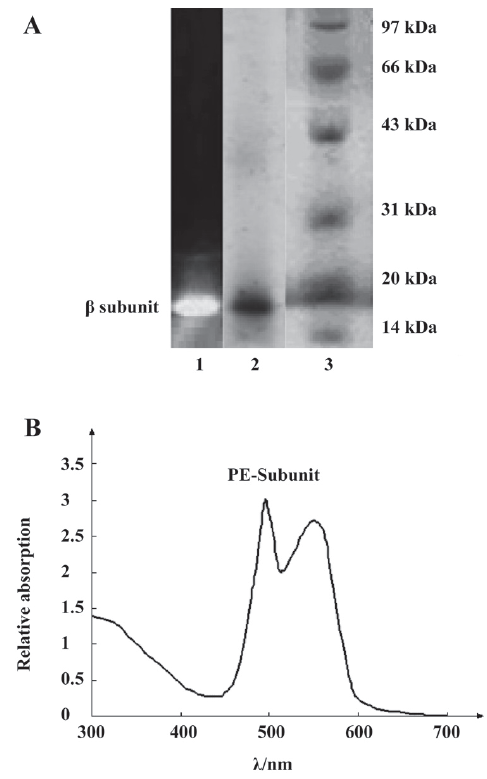
Optimum preparation condition of PE-sub liposome As shown in Table 1, the orthogonal assay L9(34) was used to determine the best combination condition for the encapsulation rate. We tested 4 influential factors and found that the remarkable factor was A (Table 2). The Sj value showed that the order of the influential factors was PBS buffer volume (A)>ratio of PE-sub (B)>ratio of phosphatidylcholine to cholesterin (C)>sonication time (D). Considering the encapsulation rate, the best combination for liposome preparation was the combination of the maximum value of Ij, IIj, and IIIj. Therefore, the optimum condition was A2B3C2D2 (Table 1). The buffer volume was 20 mL; ratio of PE-sub to lipid particles was set as 1:30; ratio of phosphatidylcholine to cholesterol was set as 1:2; sonication time was set as 10 min.
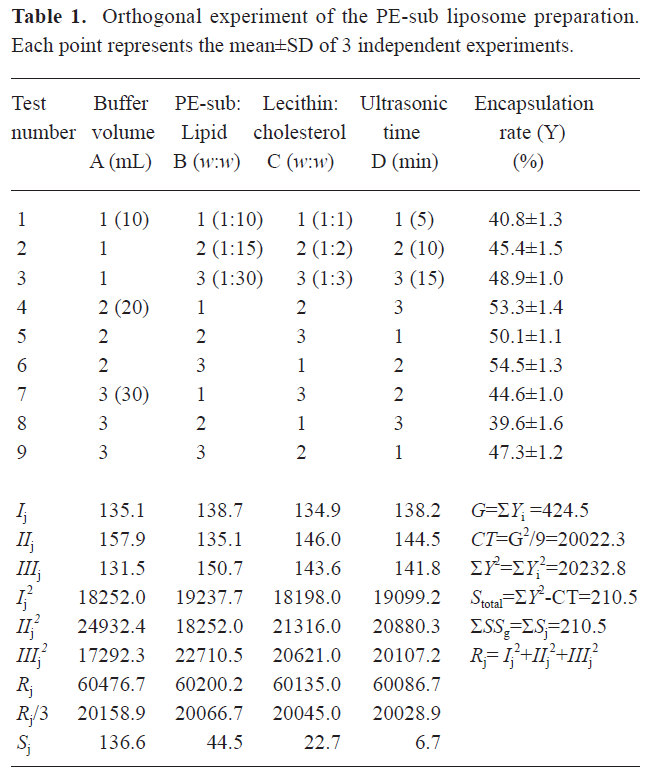
Full table
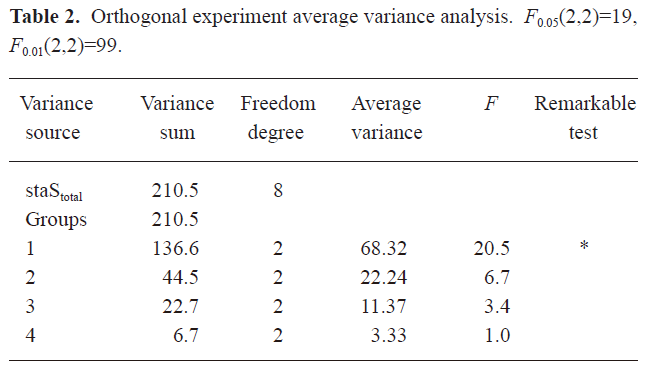
Full table
Determination of the PE-sub liposome encapsulation rate The PE-sub liposome was dissolved in PBS buffer at concentration of 0.05, 0.075, 0.10, 0.25, and 0.50 g/L. According to absorption spectra area to concentration with the linearity regress analysis, we obtained a standard curve equation: A=1537.6ρ–12.163 (r=0 9991, n=5). HPLC of the PE-sub and PE-sub liposome were shown in Figure 2. The encapsulation rate of the PE-sub liposome is shown in Table 1.
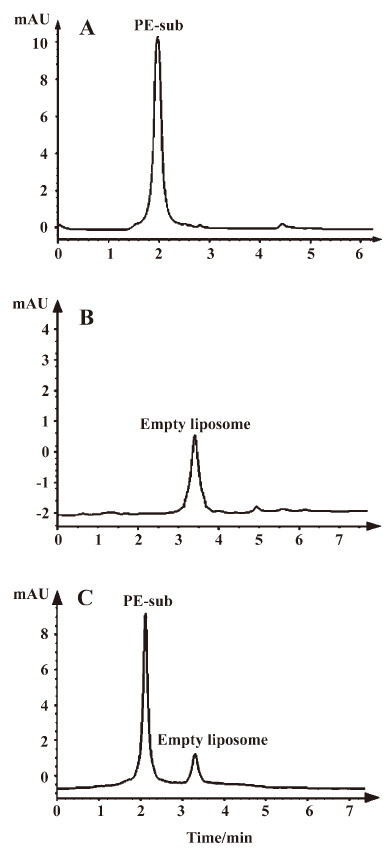
As shown in Figure 2, the PE-sub and empty liposome were well–separated. The empty liposome had no influence on the encapsulation rate.
The RSD of the precision experiment was 1.07%± 0.03% (n=5), for the repetition experiment it was 1.16%±0.04% (n=5), and for the stability experiment it 1.89%±0.05% (n=5), calculated by the absorption spectra area. This liposome preparation method demonstrated good precision, repetition, and stable characteristics. The average reclaiming rate of the sample reached 99.75%±3.1% with 1.18%±0.02% RSD (n=5).
Morphological observation and particle size analysis Because of the emission peak of the PE-sub located at 650 nm, the orange red fluorescence was observed in the PE-sub liposome under a fluorescence microscope. This demonstrated that the PE-sub was encapsulated into the liposome and still retained fluorescence activity (Figure 3).
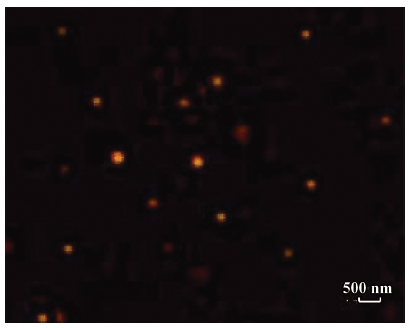
The size distribution of the PE-sub particle was determined using transmission electron microscopy. The overall size distribution was measured using a laser scanning light scattering sizing system. Transmission electron microscopy (TEM) studies on particles revealed a relatively uniform size distribution (Figure 4), which was quantified using laser sizing showing an average particle diameter of 136 nm (Figure 5). Its particle size mainly ranged from 80 to 200 nm.
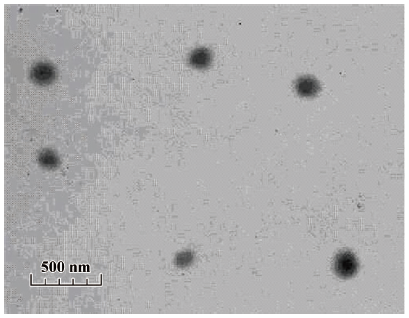
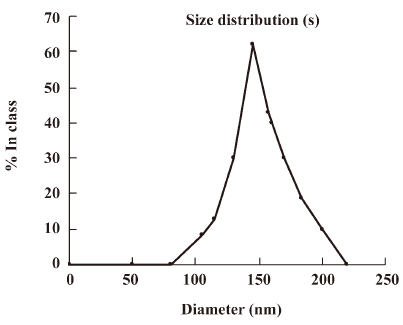
PE-sub liposome enhancing cellular uptake To monitor cellular uptake and subcellular distribution, the PE-sub, with or without the liposome, was incubated with HL7702 and HepG2 cells for 4 h. Because of its good fluorescence characteristics, the subcellular localization of these particles was monitored under a fluorescence microscope after 1–6 h. The data in Figure 6 revealed that these particles bound to the cell surface and were also internalized into the cytoplasm, but not in the nucleus.
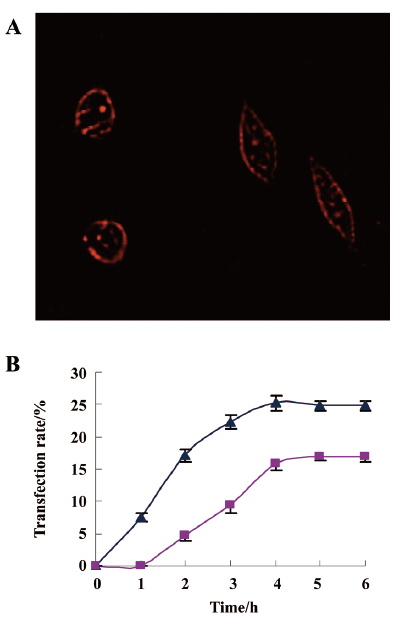
At a concentration of 100 μg/mL, the PE-sub liposome transfection rate in the HepG2 cells reached 17.2%±1.06% at 2 h, 25.3%±1.15% at 4 h, and remained stable at 5–6 h. The PE-sub liposome was transfected into the HL7702 cells slowly in the first 2 h, reached 15.8%±1.04% at 4 h, and remained stable at 5–6 h (Figure 6A). This result demonstrated the higher transfection rate of the PE-sub-liposome in the cells at 4 h. Increasing the incubation time of the PE-sub with or without the liposome did not increase PE-sub fluorescence intensity after 6 h. Whether naked or liposome-bound, the cell accumulation of the PE-sub liposome in the HepG2 cell line increased at a faster rate than it did at the same dosage in the HL7702 cell line. Therefore, we chose 4 h as the PDT treatment time in the following experiment.
PDT effect comparison between the PE-sub and PE-sub liposome In order to study the PE-sub liposome as a photosensitizer on cancer cell and its cell toxicity on normal cells, we treated cells with different concentrations of the PE-sub liposome for 4 h, irradiated with 22 J/cm2 of light. The PE-sub served as the control. When the particles were incubated with the cells during rinsing with PBS, and irradiated with laser, no cytotoxicity was observed; in fact, most cells were viable. These results revealed that 22 J/cm2 laser irradiation produced no cytotoxicity. Furthermore, when the particles were incubated for 4 h without irradiation with laser light, no detectable cytotoxicity was observed, suggesting that the liposome alone was not cytotoxic to the cells.
As shown in Tables 3,4, at a concentration of 100 μg/mL, the combination of 4 h incubation with the PE-sub liposome and laser light irradiation resulted in cytotoxicity, where 47.8%±2.29% of the HepG2 cells survived, 20%±1.12%(n=10, P<0.01) statistically lower than the PE-sub and 13%±0.76% than PE-Sub-lip; the half lethal dose of the PE-sub liposome was approximately 75 μg/mL. At the same concentration, the survival rate of the HL7702 cells reached 79%±3.23% after PDT treatment. Cell death induced by particles embedded with PE-sub was dose-dependent when monitored at 25–200 µg/L. Therefore, the PE-sub liposome showed lower cell toxicity on normal cells and good tumor-targeting characteristics.
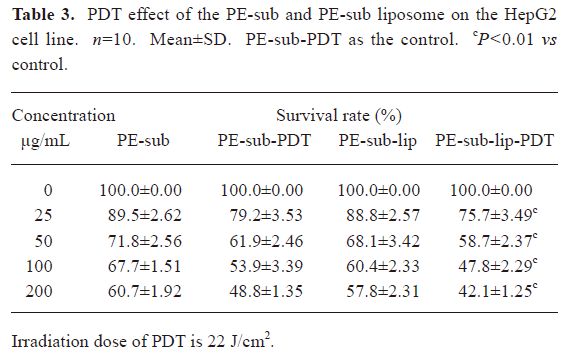
Full table
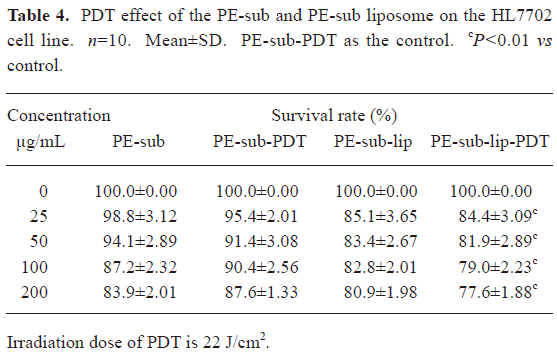
Full table
Apoptotic analysis of PDT-treated cells To investigate the PDT effect on the cell cycle, PDT-treated HepG2 and HL7702 cells were harvested for flow cytometric analysis and morphological observation. The flow cytometric analysis revealed an average 55% cell apoptotic rate for HepG2 cells, and 30% for HL7702 cells at a concentration of 100 μg/mL. The PE-sub liposome was irradiated with 22 J/cm2 laser light and the cells were arrested mainly in the G0/G1 phase. For the morphological observation of the PDT-treated cells, the cells were stained with acridine orange and monitored under a fluorescence microscope, As shown in Figure 7, before PDT treatment, the nucleus (bright green) was an ellipse. The RNA fraction (orange) in the cytoplasm was located near the nucleus, cells had tight connection in HepG2 (Figure 7A) and HL7702 cells (Figure 7C). After PDT treatment, the nucleus separated with typical condensation of heterochromatin. The cytoplasmic RNA fraction also dissociated into pieces, and the cell membrane swelled and lost cell connection. Both HepG2 (Figure 7B) and HL7702 (Figure 7D) cells showed typical apoptotic characteristics, with the only difference being the apoptotic rate, as determined by the flow cytometric analysis.
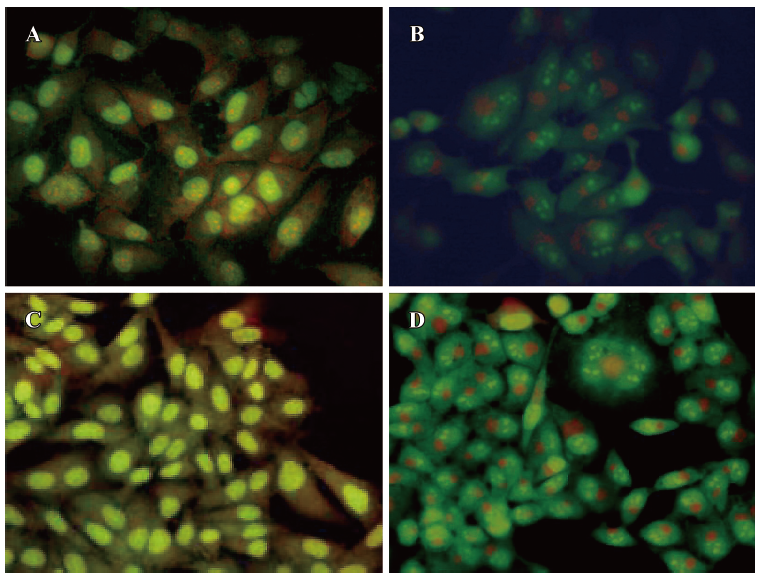
Discussion
In the process of liposome preparation, the film dispersion method, an ideal method for preparing protein liposomes, was used in this present investigation. We found that the PE-sub liposome could be readily achieved, yielding particles with a mean diameter of 136 nm (range: 80–200 nm). Temperature is an important influence factor on the liposome encapsulation rate, so when the temperature increases, the fluidity of the liposome membrane will also increase, enveloped R-PE will leak out from its liposome. The temperature should be strictly controlled below 40 ºC in order to maintain the biological activity of the PE-sub. Because of the fluorescence activity of the PE-sub, the uniformed nanoparticles of the PE-sub liposome could be seen by fluorescence microscope and TEM.
The size of nanoparticles is considered to be the one of main factors determining the efficiency of nanostructural antitumoral drugs. If a photosensitizer contains liposomes of different sizes, its accumulation is determined mostly by the content of fractions of 70–150 nm in diameter. An investigation into the photodynamic efficiency of Tiosens has shown that the use of dispersions with liposomes below 150 nm causes 80%–90% tumor growth inhibition, while the use of dispersions that are larger in size gives less-pronounced effects[14]. In the present study, the average particle size of the PE-sub was below 150 nm.
The enhancement of cellular uptake with the liposome in tissue culture has been shown to be dependent on incubation time. The PE-sub liposome transfection rate in the HepG2 cell line reached 17.03% at 2 h, 25.33% at 4 h, and remained stable at 5–6 h. The transfection rate was approximately 8%–12% higher than naked PE-sub (data not shown). Furthermore, cell accumulation of the PE-sub liposome in the HepG2 cell line increased at a faster rate than in the HL7702 cell line at the same dosage, whether naked or liposome bound. A large percentage of the PE-sub was located in the cell membrane and cytoplasm. Normal liver cells were less affected by liposome carrier than HepG2 cells, indicating that liposome carrier was transfected faster in proliferating cells like cancer cell[6,15]
In vitro characterization studies revealed that exposure of PE-sub encapsulated nanoparticles to 496 nm laser light produced significant cytotoxicity on liver cancer cells in a concentration-dependent manner compared to the normal liver cells. Due to different transfection rate between PE-sub liposome and naked PE-sub, the PDT effect of PE-sub liposome was also improved on HepG2 cells; use of liposome carrier, the half lethal dose of PDT was 75 μg/mL on HepG2. Whereas at the same concentration, the survival rate of HL7702 reached 79% after PDT treatment. One important finding of the present study was the lower cell toxicity on normal cell and good tumor-targeting characteristics of PE-sub liposome.
PDT of mammalian cells in culture can induce apoptosis, necrosis, or cell death including both apoptosis and necrosis. The effects depended on the cell type, the nature of the photosensitizer, and the severity of the treatment conditions[15]. In this study, 45% apoptotic cells could be seen at a concentration of 100 μg/mL PE-sub liposome, irradiated with 22 J/cm2 laser light.
The nanoparticle formulation of Photofrin provides interesting possibilities for new avenues to significantly improve PDT. The PE-sub liposome showed a better PDT effect compared with the PE-sub in vitro photodynamic experiment. We will make a further study on the slow release characteristics, long-term effects, and protective characteristics of a liposomal photosensitizer for unstable PE-sub in appropriate animal model and its tumor-targeting advantages.
Acknowledgements
The authors wish to thank Dr Hui ZHONG for providing the HL7702 and HepG2 cell lines, Yong-long ZHUANG (Experimental Center of Modern Technology, Anhui University, China) and Xing-xing XIA (School of Life Sciences, Anhui University, China) for invaluable assistance in preparing the TEM and HPLC.
References
- Sibata CH, Colussi VC, Oleinick NL, Kinsella TJ. Photodynamic therapy in oncology. Exp Opin Pharmacother 2001;2:917-27.
- Henderson BW, Gollnick SO. Mechanistic principles of photo-. dynamic therapy. In: Vo-Dinh T, editor. Biomedical photonics handbook. Boca Raton (FL): CRC Press; 2003. p 36.1–36.27
- Medina OP, Zhu Y, Kairemo K. Targeted liposomal drug delivery in cancer. Curr Pharm Des 2004;10:2982-9.
- Li S, Wang SM, Gong XQ, Chen LX. A rod-linker contained R-phycoerythrin complex from the intact phycobilisome of the marine red alga Polysiphonia urceolata. J Photochem Photobiol B 2004;76:1-11.
- Huang B, Wang GC, Zeng CK, Li ZG. The experimental research of R-phycoerythrin subunits on cancer treatment. A new photosensitizer in PDT. Cancer Biother Radiopharm 2002;17:35-42.
- Chen B, Pogue BW, Hasan T. Liposomal delivery of photosensitizing agents. Expert Opin Drug Deliv 2005;2:477-84.
- Wang T, Deng YJ, Geng YH, Gao ZB, Zou JP, Wang ZX. Preparation of submicron unilamellar liposomes by freeze-drying double emulsions. Biochim Biophys Acta Biomembr 2006; 1758: 222–31.
- Snyder JW, Greco WR, Bellnier DA, Vaughan L, Henderson BW. Photodynamic therapy, a means to enhanced drug delivery to tumors. Cancer Res 2003;63:8126-31.
- Niu JF, Wang GC, Tseng CK. Method for large-scale isolation and purification of R-phycoerythrin from red alga Polysiphonia urceolata Grev. Protein Expr Purif 2006;49:23-31.
- Lu W, He LC, Zeng XM. HPLC method for the pharmacokinetics and tissue distribution of taspine solution and taspine liposome after intravenous administrations to mice. J Pharm Biomed Anal 2008;46:170-6.
- Zhang B, Wang DK, Song Y, Zhang ZX, Lu GJ. Preparation of asarone liposome and the measurement of encapsulation efficiency. J Chin Med China 2007;1:67-8.
- Chen ZP, Zhu JB, Chen HX, Xiao YY. A simple HPLC method for the determination of bifendate: application to a pharmacokinetic study of bifendate liposome. J Chromatogr B 2007;857:246-50.
- Glazer AN, Hixson CS. Subunit structure and chromophore composition of Rhodophytan phycoerythrins. Porphyridium cruentum B-phycoerythrin and beta-phycoerythrin. J Biol Chem 1977;252:32-42.
- Meerovich GA, Meerovich IG, Pevgov VG, Lukyanets EA, Oborotova NA, Gurevich DG, . Influence of liposome size on accumulation in tumor and therapeutic efficiency of liposomal near-IR photosensitizer for PDT based on aluminum hydroxide tetra-3-phenylthiophthalocyanine.[Abstract]. Nanotech 2008 Conference. Boston: June 1–5; 2008.
- Abels C. Targeting of the vascular system of solid tumors by photodynamic therapy (PDT). Photochem Photobiol Sci 2004;3:765-71.