Effect of plagiochin E, an antifungal macrocyclic bis(bibenzyl), on cell wall chitin synthesis in Candida albicans1
Introduction
The incidence of fungal infections and associated mortality rates has dramatically increased during the last few decades[1–3]. Systemic mycoses and some forms of dermatomycosis are the cause of mortality in many patients who underwent anticancer chemotherapy, radiation therapy, parenteral nutrition, or organ transplants[4]. This has resulted in the increased use of broad-spectrum antifungal agents and the initiation of protocols for antifungal prophylaxis in patients at risk. The long-term use of antifungal agents, such as fluconazole, has led to drug resistance in some cases, possibly due to alteration of the drug target, changes at the cellular level of the demethylase enzyme, or changes in drug permeability[5–7]. Antifungal drug resistance has become an important problem in a variety of infectious diseases today. For instance, 33% of late-stage AIDS patients had drug-resistant strains of Candida albicans in their oral cavities[8]. Antifungal drug resistance has exacerbated the need for the next generation of new safe antifungal agents.
The macrocyclic bis(bibenzyls) isolated from liverworts[9–11] has demonstrated a wide range of biological activities, including antifungal, antibacterial, cytotoxic (antitumoral and antileukemia), and 5-1ipoxygenase- and calmodulin-inhibitory activities[12–14]. In our ongoing program for the discovery of new antifungal ingredients from liverworts, a macrocyclic bis(bibenzyl), plagiochin E (PLE; Figure 1), was isolated from Marchantia polymorpha L (Marchantiaceae) by bioassay-guided separation and was found to have antifungal activity against Candida albicans[15]. PLE had identical antifungal activity against both fluconazole-susceptible and fluconazole-resistant isolates of Candida albicans assayed by broth microdilution[16], which suggested that the antifungal mechanism of PLE is possibly distinct from that of fluconazole, one of the inhibitors of ergosterol biosynthesis[17,18].
As we know, the fungal cell wall plays an important role in the growth and viability of fungi; the 3 major structural elements, glucan, chitin, and mannan[19,20], are generally considered therapeutic targets[21,22]. Chitin, a long linear homopolymer of β-1,4-linked N-acetylglucosamine (GlcNAc), is synthesized by the incorporation of GlcNAc units from the precursor uridine 5'-diphospho-N-acetylglucosamine (UDP-GlcNAc) in a reaction catalyzed by chitin synthetase (Chs)[23,24]. Three different Chs (Chs1, Chs2, and Chs3) and their associated genes (CHS1, CHS2, and CHS3) have been identified in Candida albicans[25]. Chitin is indispensable for the construction of the cell wall, and therefore, for fungal survival. The inhibition of chitin polymerization may affect cell wall maturation, septum formation, and bud ring formation, damaging cell division and cell growth in the process. Several compounds that affect chitin synthesis have been identified, such as nikkomycin Z and polyoxin D, which are well-known competitive Chs inhibitors, presumably due to structural similarity with the Chs substrate[26–28].
To understand the biochemical basis of the antifungal activity of PLE, the changes of ultrastructure in Candida albicans was observed under transmission electron microscopy (TEM) after incubation with PLE for 24 h. The results showed that the cell wall of Candida albicans was seriously damaged and cell division was inhibited, which suggested that the antifungal effect of PLE was associated with its effect on the fungal cell wall. Therefore, a further study on the effect of PLE on chitin synthesis in Candida albicans was carried out at the cellular and molecular levels for identifying a promising cellular target of PLE.
Materials and methods
Chemicals PLE was isolated from liverwort Marchantia polymorpha L (Marchantiaceae) in our laboratory, and its structure had been identified by nuclear magnetic resonance (NMR) and mass spectrometry (MS). A stock solution of PLE at a concentration of 20 480 mg/L was prepared in DMSO and frozen at –20 °C. In each assay, DMSO comprised <1% of the total test volume. N-acetyl glucosamine, dansyl chloride, osmium tetroxide, EPON-812, ethidium bromide, bovine serum albumin, lyticase, and chitinase were purchased from Sigma (St Louis, MO, USA). Trypsin, soybean trypsin inhibitor, and MOPS (morpholinepropanesulfonic acid) were purchased from Amresco (Solon, OH, USA). Tryptone, yeast extract, glucose, and agar were purchased from Oxo (London, UK). RPMI-1640 and agarose were purchased from Gibco (Grand Island, NY, USA). The advantage RT-for-PCR kit and SYBR green real-time PCR master mix were purchased from TOYOBO (Osaka, Japan).
Microorganism and media The isolate of Candida albicans [CA2, minimal inhibitory concentration (MIC)=16 mg/L] used in this study was kindly donated by Shandong Provincial Qianfoshan Hospital (Ji-nan, China), and stored at –80 °C in medium containing 20% glycerol. Microbiological media were either YPD medium (2% tryptone, 1% yeast extract, and 2% glucose) or YPD solid medium with 2% agar or RPMI-1640 buffered with 0.165 mol/L MOPS to pH 7.0. Prior to testing, the isolate was passaged on YPD solid medium to ensure purity and viability.
Effect of PLE on ultrastructure of Candida albicans TEM was performed to observe the effect of PLE on the cell ultrastructure of Candida albicans. The cells were collected after being treated with 16 mg/L PLE at 35 ºC for 24 h, washed twice with phosphate-buffered solution (PBS), and centrifuged for 5 min at 500×g. The pellet was fixed in 2.5% glutaraldehyde at 4 ºC for 24 h and then placed in 1% osmium tetroxide in 0.1 mol/L sodium cacodylate (pH 7.4) for 1 h. The cells were desiccated in graded series of acetone and embedded with EPON-812. Ultra-thin sections were prepared and observed under TEM (JEM-200EX; JEOL, Tokyo, Japan) with 5000 magnification after double staining with uranium and plumbum. At the same time, the untreated cells were used as the control.
Effect of PLE on Chs activity in vitro The substrate 6-O-dansyl-N-acetylglucosamine (DNAG) was synthesized in 3 steps from N-acetyl glucosamine according to a previously-described procedure[29,30].
Chs was extracted according to a previously-described procedure with some modifications[31]. Logarithmic phase cells grown in 100 mL YPD medium were collected, washed twice with sterile water, and homogenated. Broken material was collected and cell debris was removed by centrifugation at 5000×g for 5 min at 4 ºC. The supernatant was collected and stored at –80 ºC. The protein concentration was measured by the Lowry’s method, using bovine serum albumin as the standard.
Chs activity assay was performed, as previously described, with slight modifications[32]. For Chs1 activity, the standard assay mixture containing 4.3 mmol/L magnesium sulfate, 32 mmol/L Tris-HCl at pH 6.5, 5 µL trypsin (2 g/L), 1.1 mmol/L DNAG, and 17 µL membrane suspension in a total volume of 46 µL was used. For Chs2, the standard assay mixture containing 3.2 mmol/L cobaltous nitrate and 32 mmol/L Tris-HCl (pH 8.0) was used. For Chs3, it containing 3.2 mmol/L cobaltous nitrate, 10 mmol/L nickelous nitrate, and 32 mmol/L Tris-HCl (pH 8.0), but did not need trypsin activation. Mixtures were incubated for 15 min at 30 ºC. Proteolysis was stopped by adding 5 µL soybean trypsin inhibitor (3 g/L) on ice. Different concentrations of PLE were added. Each reaction was incubated for 2 h at 30 ºC and stopped by the addition of 1 mL of 10% trichloroacetic acid. The pellet was then treated for 10 min with hot 5% KOH. The incorporated fluorescence was determined by diluting the hot alkali-insoluble material in water and measuring the light emitted at 501 nm with an excitation wavelength of 321 nm in a fluorospectrophotometer. All reactions were carried out in triplicate.
Effect of PLE on chitin synthesis in situ Fluorescent microscopy was used to assay the effect of PLE on chitin biosynthesis of Candida albicans in situ[29,30]. Logarithmic phase cells grown in 100 mL YPD medium were collected, washed once with 50 mmol/L Tris-HCl (pH 7.4), and resuspended in 20 mL of 1 mol/L sorbitol, 100 mmol/L Tris-HCl (pH 7.4), 5 mmol/L EDTA, and 15 mmol/L β-mercaptoethanol at 35 ºC for 30 min. In total, 50 000 U lyticase and 5000 U chitinase were added and the suspension was incubated at 35 ºC for 1 h. The spheroplasts were collected by centrifugation for 5 min at 200×g and resuspended in regeneration medium RPMI-1640 supplemented with 1 mol/L sorbitol and 1 g/L DNAG to 1×106 to 5×106 CFU/mL. PLE was added from stock solution to a final concentration of 16 mg/L. After incubation at 35 ºC for 24 h, the cells were collected by centrifugation for 5 min at 200×g, washed twice, resuspended in PBS buffer, then observed with an Olympus fluorescent microscope (Olympus 1×81; Olympus, Tokyo, Japan) equipped with a 60× oil immersion lens.
Effect of PLE on CHS expression Real-time RT-PCR was performed to assay the effect of PLE on CHS expression. RNA was isolated by the hot phenol method. Candida albicans was initially grown for 24 h at 35 °C in liquid YPD medium. The cultures were then diluted to 1×105 CFU/mL in 40 mL fresh YPD medium and allowed to grow for an additional 8 h at 35 °C. The cells were collected by centrifugation at 1500×g for 3 min, washed once with distilled water, and then centrifuged at 5000×g for 10 s at 4 °C. The pellet was resuspended in 400 µL N-[tris(hydroxymethyl)methyl]-2-aminoethanesulfonic acid (TES) buffer and 400 µL acid phenol was then added. The pellet was vortexed for 10 s, incubated for 30–60 min at 65 °C, and then incubated for 5 min on ice. After centrifugation at 5000×g for 5 min at 4 °C, the upper aqueous phase was transferred to a new microcentrifuge tube. Then 400 µL of chloroform was added. The mixture was vortexed for 10 s, centrifuged at 5000×g for 5 min at 4 °C, and the upper aqueous phase was transferred to a new microcentrifuge tube. Isopropanol was added to precipitate RNA. Samples were incubated at 4 °C for at least 2 h, and centrifuged at 2000×g for 8 min. The pellet was washed with 75% ethanol and resuspended in DEPC-treated water in order to protect the RNA from degradation by RNases. RNA was quantitated by diluting 1 µL into 100 µL with water, and the A260 and A280 were determined. Single-stranded cDNA was synthesized with oligo(dT) primers from 5 µg total RNA using the Advantage RT-for-PCR kit accordingly to the manufacturer's instruction. The accumulation of PCR products was measured in real-time PCR by using the SYBR green real-time PCR master mix. The sequences of the primers are listed in Table 1. The reaction was performed in a MiniOpticon real-time PCR system (Bio-Rad, Hercules, CA, USA). The basic protocol for real-time PCR was an initial incubation at 50 °C for 2 min, 95 °C for 10 min, followed by 44 cycles of 95 °C for 15 s, and 60 °C for 1 min. After that, a melting curve was constructed for verification of the specificity of PCR products by increasing the temperature from 60 to 94 °C in sequential steps of 0.5 °C for 10 s. All samples were run in triplicate, and 18S rRNA was used as the housekeeping gene for arbitrary unit calculation for every tested gene.
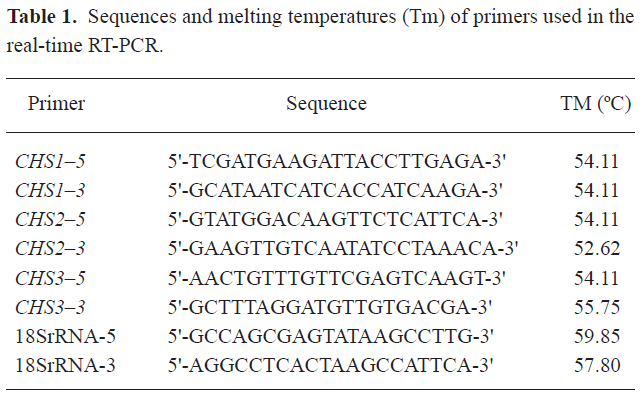
Full table
Statistical analysis Values are presented as mean±SD. Statistical significance was determined by Student’s t-test, and P<0.05 was considered statistically significant.
Results
Effect of PLE on cell ultrastructure of Candida albicans analyzed by TEM The Candida albicans images under TEM after incubation with PLE for 24 h are shown in Figure 2. In the cells treated with PLE, the most obvious morphological change appeared in the cell wall, which was much thicker and had a significantly lower electron density than that of the untreated cells whose cell wall was intact. Many cells were also aggregated after treatment with PLE, in which the mother and daughter cells were connected by immature septa.
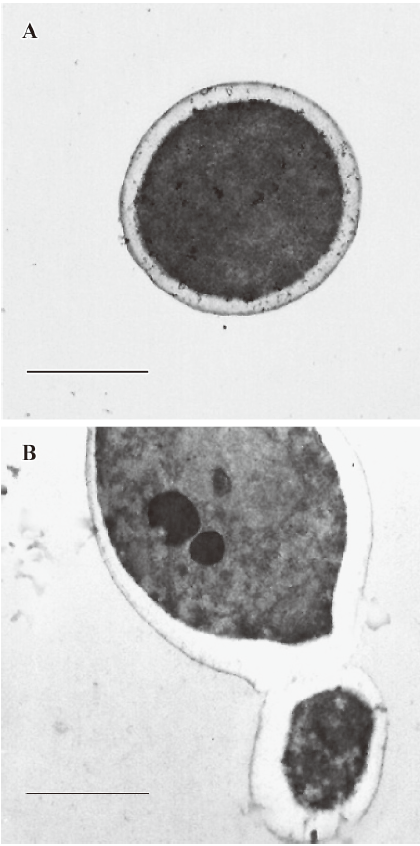
Effect of PLE on Chs activity in vitro Chs activity is often assayed by radioactivity using UDP-[14C] GlcNAc. In this study, a fluorescent method was selected for assessing Chs activity using DNAG as the substrate. Three different Chs can be catalyzed by different divalent metal ions at different pH optimums as described by Chio et al who have formulated conditions for the specific determination of each synthetase in the presence of the others[32].
In this assay Chs activity was measured in the Candida albicans at the logarithmic growth phase. The results revealed that residual activity of the 3 enzymes (percentage of incorporation compared to the incorporation in the absence of PLE) decreased obviously in relation to the doses of PLE, as shown in Figure 3. When the concentration of PLE was 16 mg/L, the residual activities of Chs1, Chs2, and Chs3 were 77.41%±2.39%, 68.35%±1.96%, and 50.71%±1.51%, respectively.
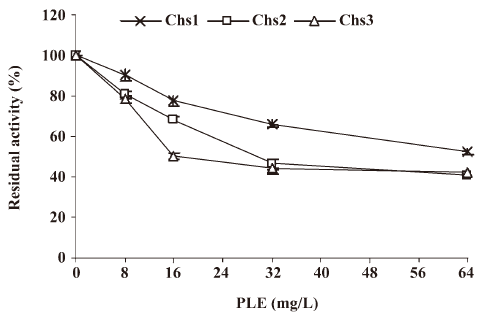
Effect of PLE on chitin synthesis in situ The fungal cell wall is a complex matrix of polysaccharides (glucans, mannans, and chitins), proteins, and minor amounts of lipids. The removal of this essential structure for cell integrity and viability by controlled enzymatic digestion in an isoosmotic medium brings about spheroplast formation. When spheroplasts are incubated in an osmotically-stabilized liquid nutrient medium, cell wall precursors are secreted into the culture medium to de novo synthesize the cell wall[33].
To assay the effect of PLE on the chitin synthesis of Candida albicans in situ, spheroplast regeneration in the presence of DNAG was followed. First, the cells were converted to spheroplasts by enzymatic digestion of chitinase and lyticase, as described earlier, and then suspended in the regeneration medium supplemented with 1 mol/L sorbitol as the osmotic stabilizer in the presence of DNAG. The cells were regenerated for 24 h. Spheroplasts regenerated in the presence of DNAG were fluorescent, but samples treated with PLE at concentration of 16 mg/L were less fluorescent and aggregated, as shown in Figure 4. The results indicated that the incorporation of DNAG into chitin in cells was moderately inhibited by PLE. Because it is a selective incorporation of DNAG into the chitin biopolymer rather than a non-specific absorption in the cell wall matrix[30], the results suggested that PLE could moderately inhibit the synthesis of chitin in situ.
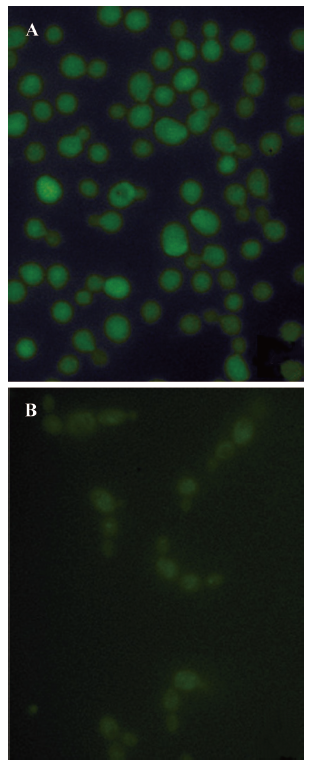
Effect of PLE on the CHS expression Real-time RT-PCR was performed to determine the effect of PLE on the relative expression levels of 3 different CHS genes, which were shown as ratios to the mean levels of the control. The results showed that the relative expression level of CHS1 decreased significantly to 0.2137±0.0207 after treatment with 16 mg/L PLE at 35 °C for 8 h, while the relative expressions of CHS2 and CHS3 increased slightly to 1.0325±0.2708 and 1.0257±0.06570, respectively Figure 5.
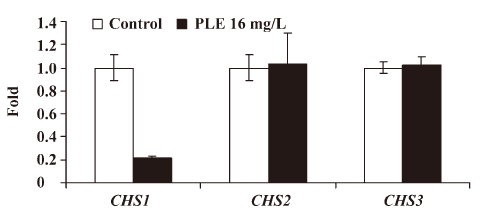
Discussion
Fungal cells are coated with a carbohydrate-containing wall, which is necessary for cell integrity[34,35]. As a consequence, the division of fungal cells is different from that of mammalian cells, whose cytokinesis is affected by the contraction of an actomyosin ring that invaginates the plasma membrane until it pinches off, splitting the dividing cell into 2 daughter cells[36]. This implies the formation of the septum between the mother and daughter cells to permit their separation without lysis. The yeast septum is made in 3 different stages, and Chs plays a central role in this process. First, a chitin ring is formed at the basis of an emerging bud. Second, the membrane invaginates, and chitin is secreted from it to form the primary septum. Next, secondary septa are built up from both the mother and daughter cell sides. Finally, the 2 cells are separated by the action of a chitinase that partially hydrolyzes the primary septum[37–39].
Three Chs isoforms have been identified in Saccharomyces cerevisiae and their functions are known[40,41]: ScChs1 repairs damaged chitins during cell separation, ScChs2 is involved in the formation of the primary septum disk, and ScChs3 is responsible for the synthesis of the chitin ring at the base of an emerging bud and cell wall chitin synthesis. ScCHS2 mutants are unable to construct a primary septum. Although they usually succeed in completing cell division, their septa are thick, amorphous, and often show lacunae. Chitinase cannot act on these septa, and as a result, cell separation is defective and the cells form large clumps[42]. ScCHS3 mutants do not lay down the chitin ring at the base of emerging buds, but they have a tri-layered septum. They also lack the chitin dispersed in the cell wall and involved in cross-links[43]. Three isoforms of Chs in Candida albicans have also been identified. Sequence homology in amino acids matched Chs1 with ScChs2, Chs2 with ScChs1, and Chs3 with ScChs3, and their functions[44–46]. Polyoxins are a competitive inhibitor of Chs of Candida albicans. It has been reported that after treatment with polyoxin D, some pairs of abnormal cells consisted of 2 lysed cells from which the cytoplasm had been extruded at the cell junction or 2 highly-refractile cells were joined by a thin bridge[47].
In our experiments, we observed the ultrastructural changes of Candida albicans after treatment with PLE under TEM. The first change was that the cell wall became thick and had a low electron density, which suggested that the PLE changed the structure of the cell wall in Candida albicans. The second change was that many cells aggregated with immature septa, which suggested the septum formation and cell division were inhibited by PLE. As we know, Chs plays a central role in cell division of budding yeast, so the effect of PLE on chitin synthesis was assayed. The results of the enzymatic activities showed that PLE had a moderate inhibitory effect against all 3 different Chs in vitro, which is in accordance with the predicted results. The effect of PLE on chitin synthesis in situ was also determined by assaying the incorporation of DNAG into chitin during the spheroplast regeneration of Candida albicans in presence of PLE, and the results showed that PLE inhibited chitin synthesis in situ, which verified the above results. Chitin is a major structural element of the fungal cell wall, thus, the inhibition of chitin synthesis by PLE would decrease the proportion of chitins in the cell wall, which leads to a damaged cell wall with lower electron density, as observed under TEM. The partial inhibition of Chs by PLE would affect the formation of primary septa between mother and daughter cells and inhibit cell division, which induces many aggregated cells observed under TEM and fluorescent microscopy.
The different roles of Chs require that each be regulated independently, so that it will execute its function at the required time and location in the cell. The present work indicated that Chs were regulated at both the transcriptional and post-translational stages. ScChs2 is largely regulated by a process of synthesis and degradation. The shut-off transcription of ScCHS2 resulted in the rapid disappearance of ScChs2. However, the regulation of ScChs1 and ScChs3 occurs at the post-translational level, which appears to be quite stable in the absence of transcription[48,49]. The effect of PLE on chitin synthesis in situ would relate to the decreased expression of CHS. The results of the real-time RT-PCR in our experiment showed that compared with the control, the expression of CHS1 in Candida albicans was significantly downregulated after treatment with PLE, and the expressions of CHS2 and CHS3 were slightly upregulated. Because Chs1, whose sequence homology in amino acids matched that of ScChs2, is regulated at the transcriptional and post-translational stages, the downregulation of the expression of CHS1 by PLE would decrease the level of Chs1 and inhibit its activity. The regulation of Chs2 and Chs3 occurs at the post-translational level, and their activities have little correlation with the levels of transcription. Thus, the upregulation of the expressions of CHS2 and CHS3 by PLE would not affect the activities of Chs2 and Chs3. The inhibitory effects of PLE on Chs2 and Chs3 would be at the post-translational level or by the inhibition on the enzyme-active center of PLE. Overall, the effect of PLE on chitin synthesis would be caused by the decreased expression of CHS or the inhibition on the enzyme-active center.
The fungal cell wall plays a very important role in maintaining the structural integrity of fungal cells. Once the continuity of the cell wall is damaged, cellular morphology can not be maintained any longer, and the cells are rendered osmotically fragile[50]. A very small lesion in the cell wall can cause swelling in the area of the lesion, rupture of the cell membrane, and growth inhibition. The inhibition of chitin biosynthesis by PLE would affect the continuity of the fungal cell wall and damage cell growth. The results reported in the present study allow us to infer that PLE acts by inhibiting the biosynthesis of chitin as one of its possible modes of action. Because mammalian cells do not have a cell wall, these kinds of antifungal agents should be safe for humans[51]. Thus, PLE would be a potential candidate for the development of safer antifungal drugs.
Acknowledgements
We thank Shu-juan SUN of Shandong Provincial Qianfoshan Hospital of China for donating the yeast isolates, and Bo-qin LI and Lei WANG for excellent technical assistance.
References
- Fridkin SK, Jarvis WR. Epidemiology of nosocomial fungal infections. Clin Microbiol Rev 1996;9:499-511.
- Maschmeyer G. The changing epidemiology of invasive fungal infections: new threats. Int J Antimicrob Agents 2006;27:3-6.
- Mcneil MM, Nash SL, Hajjeh RA, Phelan MA, Conn LA, Plikaytis BD, et al. Trends in mortality due to invasive mycotic diseases in the United States, 1980–1997. Clin Infect Dis 2001;33:641-7.
- Urbina JM, Cortés JGG, Palma A, Lόpez SN, Zacchino SA, Enriz RD, et al. Inhibitors of the fungal cell wall. Synthesis of 4-aryl-4-N-arylamine-1-butenes and related compounds with inhibitory activities on β (1-3) glucan and chitin synthases. Bioorg Med Chem 2000;8:691-8.
- Hitchcock CA. Resistance of Candida albicans to azole antifungal agents. Biochem Soc Trans 1993;21:1039-47.
- Parkinson T, Falconer DJ, Hitchcock CA. Fluconazole resistance due to energy-dependent drug efflux in Candida glabrata. Antimicrob Agents Chemother 1995;39:1696-99.
- Prasad R, Wergifosse P, Goffeau A, Balzi E. Molecular cloning and characterization of a novel gene of Candida albicans, CDR1, conferring multiple resistance to drugs and antifungals. Curr Genetic 1995;27:320-9.
- White TC, Marr KA, Bowden RA. Clinical, cellular, and molecular factors that contribute to antifungal drug resistance. Clin Microbiol Rev 1998;11:382-402.
- Bardón A, Kamiya N, Toyota M, Takaoka S, Asakawa Y. Sesquiterpenoids, hopanoids and bis(bibenzyls) from the argentine liverwort Plagiochasma rupestre. Phytochem 1999;52:1323-9.
- Hashimoto T, Irita H, Takaoka S, Tanaka M, Asakawa Y. New chlorinated cyclic bis(bibenzyls) from the liverworts Herbertus sakuraii and Mastigophora diclados. Tetrahedron 2000;56:3153-9.
- Hashimoto T, Kanayama S, Fukuyama Y, Takaoka S, Tori M, Asakawa Y. Two novel macrocyclic bis(bibenzyls), isoplagiochins A and B from the liverwort Plagiochila fruticosa. Tetrahedron Lett 1994;35:911-2.
- Asakawa Y, Toyota M, Tori M, Hashimoto T. Chemical structures of macrocyclic bis(bibenzyls) isolated from liverworts (Hepaticae). Spectroscopy 2000;14:149-75.
- Qu JB, Xie CF, Guo HF, Yu WT, Lou HX. Antifungal dibenzofuran bis(bibenzyl)s from the liverwort Asterella angusta. Phytochem 2007;68:1767-74.
- Keseru GM, Nόgrádi M. The biological activity of cyclic bis(bibenzyls): a rational approach. Bioorg Med Chem 1995;3:1511-7.
- Niu C, Qu JB, Lou HX. Antifungal bis[bibenzyls] from the Chinese liverwort Marchantia polymorpha L. Chem Biodivers 2006;3:34-40.
- Leng P, Guo XL, Yang Y, Lou HX. Primary study on antifungal activities and reversal of fluconazole resistance of PLE. Chin Pharm J 2007;42:349-52.
- Carrillo-Muñoz AJ, Giusiano G, Ezkurra PA, Quindós G. Antifungal agents: mode of action in yeast cells. Rev Esp Quimioterap 2006;19:130-9.
- Ghannoum MA, Rice LB. Antifungal agents: mode of action, mechanisms of resistance, and correlation of these mechanisms with bacterial resistance. Clin Microbiol Rev 1999;12:501-17.
- Bowman SM, Free SJ. The structure and synthesis of the fungal cell wall. BioEssays 2006;28:799-808.
- Latgé JP. The cell wall: a carbohydrate armour for the fungal cell. Mol Microbiol 2007;66:279-90.
- Georgopapadakou NH, Tkacz JS. The fungal cell wall as a drug target. Trends Microbiol 1995;3:98-104.
- Perfect JR. Molecular targets for new antifungal drugs. Can J Bot 1995;73 Suppl 1:1187-91.
- Lipke PN, Ovalle R. Cell wall architecture in yeast: new structure and new challenges. J Bacteriol 1998;180:3735-40.
- Osumi M. The ultrastructure of yeast: cell wall structure and formation. Micron 1998;29:207-33.
- Mio T, Yabe T, Sudoh M, Satoh Y, Nakajima T, Arisawa M, et al. Role of three chitin synthase genes in the growth of Candida albicans. J Bacteriol 1996;178:2416-9.
- Gaughran JP, Lai MH, Kirsch DR, Silverman SJ. Nikkomycin Z is a specific inhibitor of Saccharomyces cerevisiae chitin synthase isozyme Chs3 in vitro and in vivo. J Bacteriol 1994;176:5857-60.
- Kim MK, Park HS, Kim CH, Park HM, Choi W. Inhibitory effect of nikkomycin Z on chitin synthases in Candida albicans. Yeast 2002;19:341-9.
- Endo A, Kakiki K, Misato T. Mechanism of action of the antifungal agent polyoxin D. J Bacteriol 1970;104:189-96.
- Bahmed K, Quilès F, Wathier M, Bonaly R, Benallaoua S, Pucci B, et al. Use of dansyl N-acetyl glucosamine as substrate for chitin synthetase activities. Proc Biochem 2005;40:2523-9.
- Carrano L, Tavecchia P, Sponga F, Spreafico F. Dansyl N-acetyl glucosamine as a precursor of fluorescent chitin: a method to detect fungal cell wall inhibitors. J Antibiot 1996;50:177-9.
- Ishiguro J, Saitou A, Durán A, Ribas JC. cps1+, a Schizosaccharomyces pombe gene homolog of Saccharomyces cerevisiae FKS genes whose mutation confers hypersensitivity to cyclosporine A and papulacandin B. J Bacteriol 1997;179:7653-62.
- Chio WJ, Cabib E. The use of divalent cations and pH for the determination of specific yeast chitin synthetases. Anal Biochem 1994;219:368-72.
- Pitarch A, Nombela C, Gil C. Collection of proteins secreted from yeast protoplasts in active cell wall regeneration. Methods Mol Biol 2008;425:241-63.
- Cassone A. Cell wall of Candida albicans: its functions and its impact on the host. Curr Top Med Mycol 1989;3:248-314.
- Chaffin WL, Pez-Ribot JL, Casanova M, Gozalbo D, Martínez JP. Cell wall and secreted proteins of Candida albicans: identification, function, and expression. Microbiol Mol Biol Rev 1998;62:130-80.
- Glotzer M. Cytokinesis: progress on all fronts. Curr Opin Cell Biol 2003;15:684-90.
- Cabib E. The septation apparatus, a chitin-requiring machine in budding yeast. Arch Biochem Biophys 2004;426:201-7.
- Schmidt M, Bowers B, Varma A, Roh DH, Cabib E. In budding yeast, contraction of the actomyosin ring and formation of the primary septum at cytokinesis depend on each other. J Cell Sci 2002;115:293-302.
- Cabib E, Roh DH, Schmidt M, Crotti LB, Varma A. The yeast cell wall and septum as paradigms of cell growth and morphogenesis. J Biol Chem 2001; 276: 19 679–682.
- Bulawa CE. Genetics and molecular biology of chitin synthesis in fungi. Annu Rev Microbiol 1993;47:505-34.
- Cid VJ, Duran A, del Rey F, Snyder MP, Nombela C, Sanchez M. Molecular basis of cell integrity and morphogenesis in Saccharomyces cerevisiae. Microbiol Rev 1995;59:345-86.
- Shaw JA, Mol PC, Bowers B, Silverman SJ, Valdivieso MH, Dura’n A, et al. The function of chitin synthases 2 and 3 in the Saccharomyces cerevisiae cell cycle. J Cell Biol 1991;114:111-23.
- Kollár R, Petráková E, Ashwell G, Robbins PW, Cabib E. Architecture of the yeast cell wall. The linkage between chitin and beta(1→3)-glucan. J Biol Chem 1995;270:1170-8.
- Munro CA, Schofield DA, Gooday GW, Gow NA. Regulation of chitin synthesis during dimorphic growth of Candida albicans. Microbiology 1998;144:391-401.
- Gow NA, Robbins PW, Lester JW, Brown AJ, Fonzi WA. A hyphal-specific chitin synthase gene (CHS2) is not essential for growth, dimorphism, or virulence of Candida albicans. Proc Natl Acad Sci USA 1994;91:6216-20.
- Munro CA, Winter K, Buchan A, Henry K, Becker JM. Chs1 of Candida albicans is an essential chitin synthase required for synthesis of the septum and for cell integrity. Mol Microbiol 2001;39:1414-26.
- Bowers B, Levin G, Cabib E. Effect of polyoxin D on chitin synthesis and septum formation in Saccharomyces cerevisiae. J Bacteriol 1974;119:564-75.
- Cos T, Ford RA, Trilla JA, Duran A, Cabib E, Roncero C. Molecular analysis of Chs3p participation in chitin synthase III activity. Eur J Biochem 1998;256:419-26.
- Choi WJ, Santos B, Durán A, Cabib E. Are yeast chitin synthases regulated at the transcriptional or the posttranslational level? Mol Cell Biol 1994;14:7685-94.
- Moore CW, Valle RD, Koy JM, Pramanik A, Gordon RE. Lesions and preferential initial localization of [s-methyl-3H] bleomycin A2 on Saccharomyces cerevisiae cell walls and membranes. Antimicrob Agents Chemother 1992;36:2497-505.
- Hector RF. Compounds active against cell walls of medically important fungi. Clin Microbiol Rev 1993;6:1-21.