Blood pressure, baroreflex sensitivity, and end organ damage in hybrid offspring of spontaneously hypertensive rats and Sprague-Dawley rats1
Introduction
Arterial baroreflex (ABR) plays a crucial role in cardiovascular regulation. It was found that ABR function, expressed by baroreflex sensitivity (BRS), was impaired in hypertensive patients and animals[1–4]. This impairment of BRS is attributed mainly to the high blood pressure (BP) level based on the following observations. (i) In renovascular hypertensive rats, BRS decreased significantly 1 d and 7 d after renal artery clipping[5,6]. One day after removal of a left renal artery clip, BRS was inversely related to the BP level. BRS was restored as early as renovascular hypertension was reversed[7]. (ii) In spontaneously hypertensive rats (SHR), many antihypertensive drugs lower BP and restore BRS at the same time[8,9]. On the other hand, it was found that BRS might be lower in normotensive children with hypertensive parents or in young spontaneously hypertensive rats (SHR) before hypertension[10,11]. Therefore, it is not yet clear how important BP level is in influencing BRS. To answer quantitatively this question was the first aim of the present work.
It is well known that BP level is an important determinant for end-organ damage (EOD) in hypertensive patients and hypertensive animals. However, it is certainly not the only determinant for EOD. In our previous work, it was found that BRS was related to the severity of EOD in SHR[12]. The second aim of the present work was to ascertain whether BRS was as important as BP level in determining the severity of EOD.
Hybrid offspring of SHR and normotensive rats are used widely to analyze the relationship between BP level and other parameters. The present work was designed to study the features of BP, BRS, and EOD, and the relationships between these parameters in hybrid offspring of SHR and Sprague-Dawley (SD) rats.
Materials and methods
Animals Sprague-Dawley rats were purchased from the Sino-British SIPPR/BK Lab Animal Ltd (Shanghai, China). SHR were provided by the Animal Center of the Second Military Medical University (Shanghai, China). SHR and SD rats were crossbred, and the F1 hybrids were inbred randomly to produce F2 hybrids. All rats were housed at controlled temperature (23 °C–25 °C) and lighting (8:00–20:00 light: dark cycle) with free access to food and tap water. All animals used in this work received humane care in compliance with institutional animal care guidelines.
Blood pressure measurement At the age of 52 weeks, the F1 and F2 hybrids were tested to determine BP in a conscious state. Systolic BP (SBP), diastolic BP (DBP), and heart period (HP) were recorded continuously using techniques described previously[13,14]. The BP signal was digitized by a microcomputer. SBP, DBP, and HP values from every heartbeat were determined on line. The mean values of these parameters during a period of 24 h were calculated, and served as SBP, DBP, and HP.
Baroreflex sensitivity measurement To determine the function of ABR in conscious rats, the methods widely used are derived from that of Smyth, which was first applied to humans[15]. The principle of this method is to measure the prolongation of HP in response to an elevation of BP. With some modifications, this method has been used in conscious rats[16,17]. A bolus injection of phenylephrine was used to induce an elevation of BP. The dose of phenylephrine was adjusted to raise SBP to between 20 mmHg and 40 mmHg. HP was plotted against SBP for linear regression analysis and the slope of SBP-HP was expressed as BRS (ms/mmHg). As there exists a delay between the stimulus and response (approximately 1 s), the slopes were calculated by computer with 1–10 beats of shift for linear regression analysis, and the slope with the highest correlation coefficient was used as BRS. A correlation analysis with 5 beats of shift, for example, means that values of HP6/SBP1, HP7/SBP2, and HP8/SBP3 were used.
Morphological examination Morphological examinations were carried out after BP recording and BRS studies. The animals were weighed and killed by decapitation. The thoracic and peritoneal cavities were opened immediately. The right kidney, aorta, and heart were excised and rinsed in cold physiological saline. The right kidney was blotted and weighed. The left ventricle was isolated, blotted, and weighed. At the same time, the aorta was cleaned of adhering fat and connective tissue. Just below the branch of the left subclavicular artery, a 30 mm-long segment of thoracic aorta was harvested, blotted, and weighed. Ratios of left ventricular weight to body weight (LVW/BW), right ventricular weight to body weight (RVW/BW), ventricular weight to body weight (VW/BW), left ventricular weight to right ventricular weight (LVW/RVW), and aortic weight to the length of aorta (AW/length) were calculated[18,19]. Histopathological observation was also carried out using our conventional method[20]. Briefly, immediately after gross detection, all samples of left ventricles in 2 mm-thick to 3 mm-thick slices, aortae, and kidney were immersed in formalin solution for more than 1 week, dehydrated in ethanol, cleared in dimethylbenzene and embedded in paraffin. The 5 µm-thick sections were then prepared and stained with hematoxylin and eosin for light microscopic evaluation.
Glomerulosclerosis score For the semiquantitative evaluation of glomerular damage, the glomerulosclerosis score (GSS) was defined as described previously[21]. From the light microscopic specimens, approximately 50 glomeruli from the outer cortex and the same number of glomeruli from the inner cortex of each kidney were graded according to the degree of sclerosis: 0, no mesangial expansion; 1, mild mesangial expansion (less than 30% of a glomerular area); 2, moderate mesangial expansion (30%–60% of a glomerular area); 3, marked mesangial expansion (more than 60% of a glomerular area); and 4, global sclerosis. This was carried out by one observer in a blind fashion using coded slides. A weighted composite sclerosis score was then calculated for each kidney according to the following formula: glomerulosclerosis score=[1×(number of grade 1 glomeruli)+2×(number of grade 2 glomeruli)+3×(number of grade 3 glomeruli)+4×(number of grade 4 glomeruli)]×100/(number of glomeruli observed).
Statistical analysis Data were expressed as mean±SEM. Comparisons between 2 groups were made by Student’s t-test. The relationships between hemodynamic parameters and organ damage parameters were analyzed by classic univariate correlation analysis. Stepwise multiple-regression analysis was used to study the independent effect of hemodynamic parameters on organ damage. F to enter and F to remove were set to P<0.05 and P>0.10, respectively. P<0.05 was considered statistically significant. Statistical analysis was carried out using software SPSS 11.0.0 (SPSS, Chicago, Illinois, USA).
Results
Blood pressure and baroreflex sensitivity in the F1 hybrids with different parenthood Blood pressure, HP, and BRS values in the F1 hybrids are shown in Figure 1. BP was slightly higher in H×S offspring rats (hybrids derived from male SHR and female SD rats) than in S×H offspring rats (hybrids derived from male SD and female SHR rats). This difference reached a significant level only for DBP in males and in the whole F1 hybrid group. Male H×S rats possess significantly higher DBP than female H×S rats, but this sex difference in DBP of S×H rats was not significant. A similar sex-difference tendency was found in SBP but never reached statistical significance. No obvious sex or strain differences in HP or BRS were found in F1 hybrids.
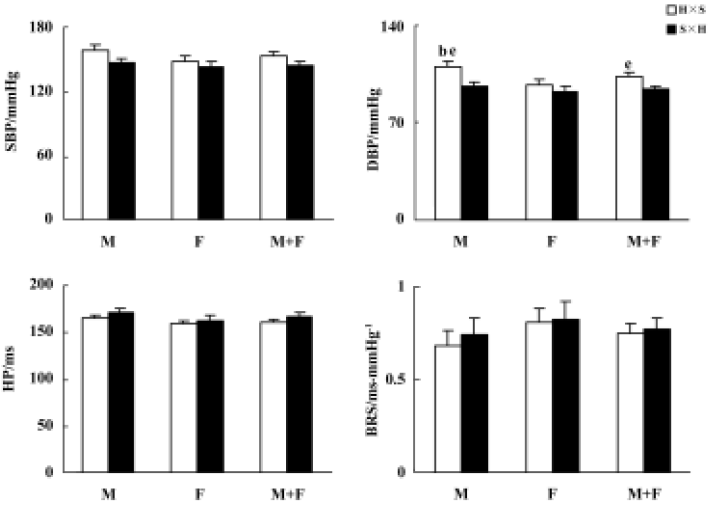
Blood pressure and baroreflex sensitivity in the F1 and F2 hybrids As shown in Figure 2, no obvious difference was found between F1 (including H×S and S×H offspring rats) and F2 hybrids with respect to BP, HP, and BRS. In F1 and F2 hybrids, male rats tended to have slightly higher BP than female rats. However, these sex differences in SBP and DBP did not reach statistical significance. The distribution of SBP and BRS in F1 and F2 hybrids is shown in Figure 3. No obvious difference between F1 and F2 was noted.
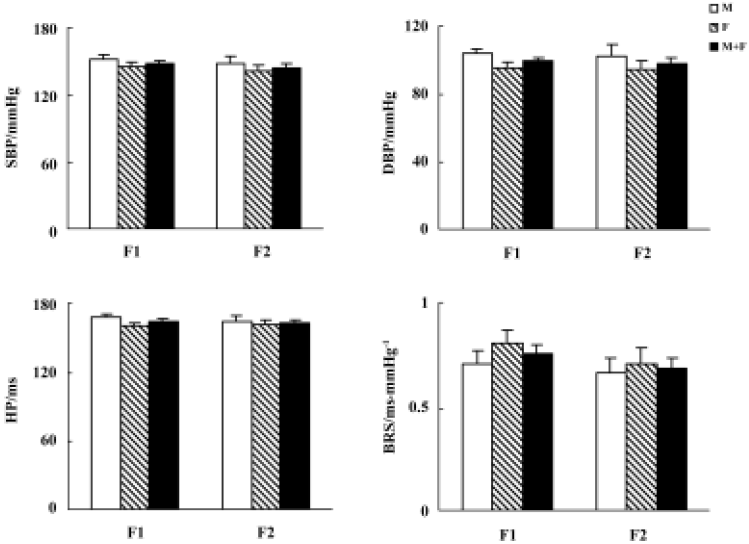
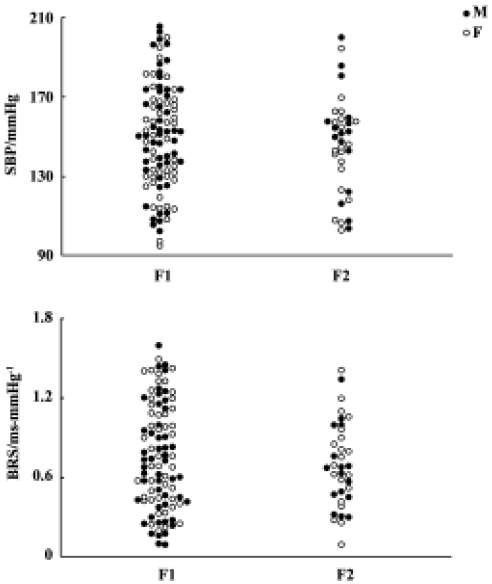
Relationship between systolic blood pressure and baroreflex sensitivity in the F1 and F2 hybrids It was found that BRS was significantly and inversely related to SBP in both F1 (r=-0.599, n=96, P<0.01) and F2 offspring (r=-0.510, n=36, P<0.01) (Figure 4A,4B). When the whole population (F1+ F2) were analyzed, the correlation coefficient (r) was -0.571 (n=132, P<0.01) (Figure 4C) and the determinant coefficient (r2) was 0.326. This value means that the BRS value is 33% determined by SBP level. In accordance with this result, it was found that lower BRS was not always linked to higher BP in the hybrid offspring rats. In the hybrids studied (Figure 4), we found some normotensive animals with a lower BRS (SBP<140 mmHg, BRS<0.4 ms/mmHg; n=5) and hypertensive animals with a higher BRS (SBP>150 mmHg, BRS>0.7 ms/mmHg; n=22). Two examples for BRS obtained from the hybrids are shown in Figure 5A, a normotensive animal with a lower BRS; and Figure 5B, a hypertensive animal with a higher BRS.
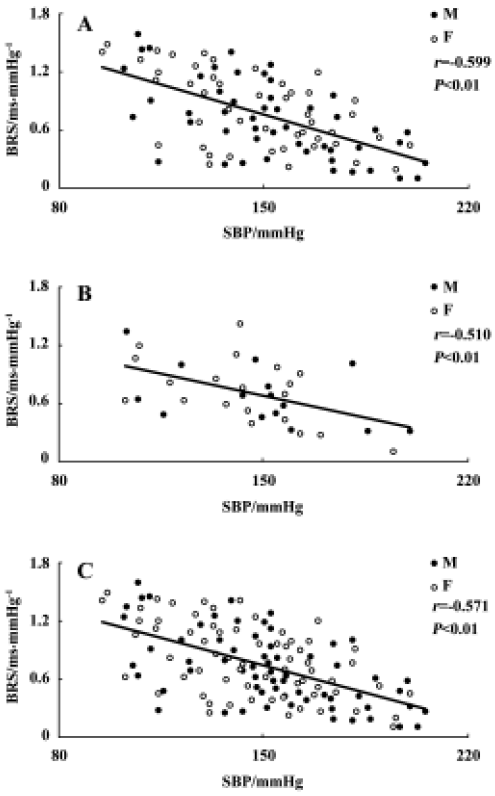
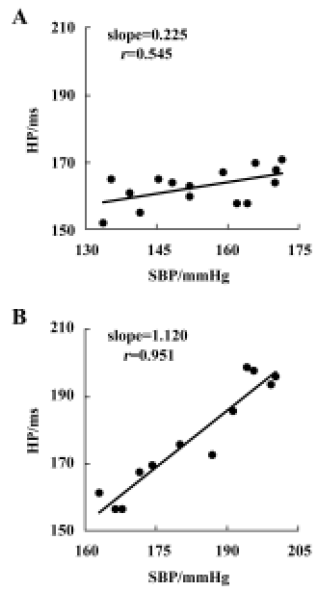
Organ damage in the F1 and F2 hybrids The average values of LVW/BW, AW/length, and GSS are presented in Figure 6. No obvious difference between F1 and F2 hybrids was found.
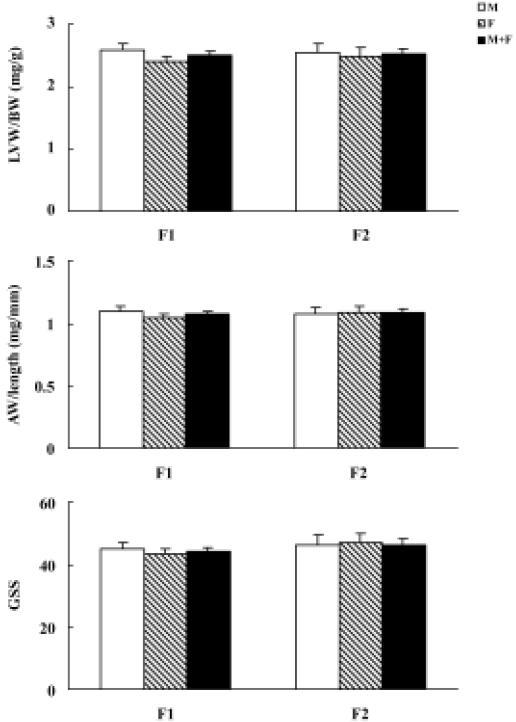
Relationships between blood pressure, baroreflex sensitivity, and organ damage in hybrids The relationships between BP, HP, BRS, and organ damage in the hybrids were shown in Table 1. It was found that LVW/BW, an index for left ventricular hypertrophy, AW/length, an index for aortic hypertrophy, and GSS, an index for renal damage, were all related positively to BP, and related negatively to BRS. There was no significant relationship between HP and organ damage parameters (Table 1). Some examples for important correlations were shown in Figure 7.
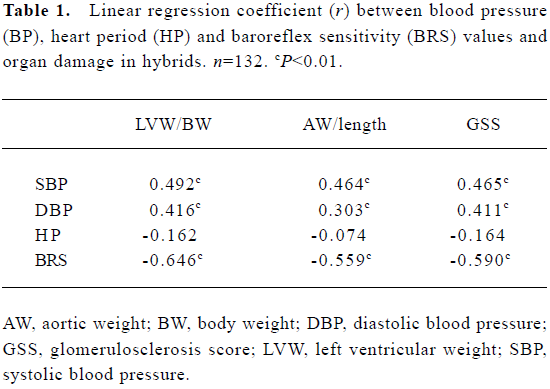
Full table
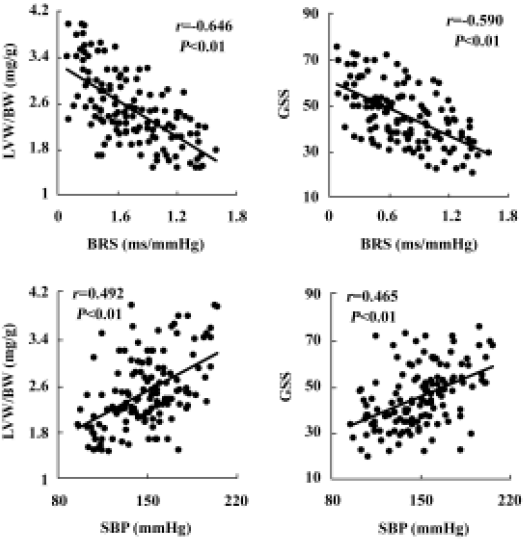
The relative dependencies of organ damage on hemodynamic parameters were assessed by stepwise multiple-regression analysis. LVW/BW, AW/length, and glomerulosclerosis score were all most significantly associated with lower BRS (β=-0.542, P<0.01; β=-0.436, P<0.01; and β=-0.482, P<0.01, respectively) and higher SBP (β=0.183, P<0.01; β=0.216, P<0.01; and β=0.191, P<0.01, respectively). After comparing the standardized partial regressive coefficients, it was found that the contribution of BRS to left ventricular, aortic hypertrophy, and glomerulosclerosis was greater than that of SBP.
Discussion
The main contributions of the present work may be summarized as follows:
Many abnormalities or changes have been reported to relate to high BP level in hypertension. Among these abnormalities or changes, some are intrinsically related to hypertension and some are only accompanying phenomena in hypertension. Hybrid offspring of hypertensive and normotensive rats are often used to distinguish these 2 sorts of changes found in hypertension. Using these hybrid offspring, it was found that the genes responsible for the hypertensive trait and those responsible for the hyperactivity trait are not tightly linked[22,23]. The relationships between BP and other parameters, such as salt appetite[24], temperature[25], and sensitivity to stress[26], have also been studied in the hybrids rats derived from hypertensive and normotensive rats. Recently, hybrid rats derived from a hypertensive and normotensive strain were produced to study genetic markers for blood pressure using statistical techniques for quantitative trait loci analysis[27]. It has been noted that BRS is lower in hypertensive patients and hypertensive animals. However, no study has been carried out using hybrid rats to analyze the relationship between BP and BRS. This may be due to the large quantity of work that it entails; the measurement of BRS in conscious and unrestrained rats requires a computerized BP monitoring system and more than 100 rats are needed for such a study. Investigating the relationship between BP and BRS using hybrid offspring rats was the first aim of the present work.
To analyze the links between BP and other parameters previous studies have used hybrid rats derived from a cross between SHR and Wistar-Kyoto (WKY) rats[22–26], stroke-prone SHR (SHRSP) and WKY rats[28], Dahl/Iwai salt-sensitive rats and WKY rats[29], New Zealand genetically hypertensive rats and Wistar rats[23], and SHR and Donryu rats[30]. In the present study, SHR were crossbred with SD rats, but not with WKY rats. This is based on the following considerations: (i) WKY rats possess a relatively higher BP level than SD rats; and (ii) compared with WKY rats, the genetic background of SD rats is more different from that of SHR. Therefore, using SD rats may help to produce hybrid rats exhibiting wider range of BP levels or BRS values, which would be expected for the correlation analysis.
It was found in previous studies that the BP of hybrid offspring derived from SHR and WKY rats depended on the strain of the male progenitor of the cross; male offspring with an SHR male progenitor had significantly higher BP (SBP, +20 mmHg) than male offspring with a WKY male progenitor[31]. The same results were also found in hybrids of SHRSP and WKY rats (SBP/DBP, +20/15 mmHg)[28]. These observations showed strong evidence for a locus or loci on the SHR or SHRSP Y chromosome that contributed to hypertension in these models. Some subsequent studies confirmed the Y-chromosome effect on BP[27,32]. In accordance with previous reports, the current work showed that the male F1 hybrids with hypertensive fathers had higher BP levels than those with normotensive fathers (SBP/DBP, +12/14 mmHg). The difference reached a significant level only for DBP. These differences found in the present work were slightly lower than those in previous studies. This might be due to the difference in BP measurement: we measured BP continuously for 24 h while others measured BP either once by tail sphygmomanometry[31] or by using average values sampled every 5 min for 10 s over a 4 d period using the radiotelemetry system[28]. However, in our study, the F1 hybrids with different parenthood had similar HP and BRS values.
In previous studies concerning hybrid offspring rats, F1, F2 or the whole population of F1 and F2 were used[22–26,30]. The use of F2 hybrids is based on the expectation of obtaining a wider distribution range in the parameters studied. In the present work, no obvious differences in the average values of SBP and BRS were found between F1 and F2 hybrids. In addition, the distribution of SBP and BRS values was similar in F1 and F2 hybrids. It is known that BP and BRS phenotypes are not controlled by only one gene, but rather by many genes[27]. This may explain the wide distribution range of SBP and BRS values. Accordingly, we propose that F1 hybrids may be suitable for these kinds of studies.
Abnormalities in baroreflex have been demonstrated in numerous studies in both experimental and human hypertension[15,33], and the impairment in baroreflex is thought to be the result of elevated BP or reduced arterial distensibility[6,7]. In agreement with previous studies, we found that BRS was significantly and inversely related to SBP in the hybrid offspring rats. However, in the hybrids studied, we also found some normotensive animals with a lower BRS and hypertensive animals with a higher BRS. These results indicate that lower BRS is not always linked to higher blood pressure in the hybrid offspring rats. Previous studies have shown that impairment of BRS may be present early in the course of, or may precede the development of, hypertension. BRS has been shown to be diminished in the early phase of hypertension in SHR as well as in borderline essential hypertension in humans; impairment of BRS is thought to be genetically determined in part[10,34]. In addition, it was found that other factors, such as alterations in endothelial prostaglandins and opioid peptides, might also contribute to the impairment of BRS[35,36]. Therefore, BP is not the only determinant of BRS. However, it is not clear how important BP level is in influencing BRS. Our study, for the first time, demonstrated quantitatively that BRS was one-third determined by SBP level (the determinant coefficient was 0.326).
It is well known that high BP level induces organ damage, while decreasing BP level can prevent EOD. Our present study showed that both SBP and DBP were significantly and positively related to LVW/BW, AW/length, and GSS. These results indicate that elevated BP has a major effect on EOD in hybrid offspring rats. However, BP level is not the only determinant for EOD. Our previous study proposed that BRS was one of the independent variables related to EOD score [12]. In the present work, BRS was significantly and negatively related to LVW/BW, AW/length, and GSS. Multiple-regression analysis showed that the contribution of BRS to left ventricular, aortic hypertrophy, and glomerulosclerosis was greater than that of SBP. The present study is the first to show that BRS makes a greater contribution to hypertensive organ damage than does BP level.
In conclusion, the present work with hybrid rats demonstrated quantitatively that BRS value was one-third determined by SBP level. Both BP level and BRS value contributed to the hypertensive organ damage. However, the contribution of BRS to the hypertensive organ damage was greater than that of BP level in these rats.
References
- Parati G, Saul JP, Castigliooni P. Assessing arterial baroreflex control of heart rate: new perspectives. J Hypertens 2004;22:1259-63.
- Mancia G, Grassi G, Ferrari AU. Reflex control of the circulation in experimental and human hypertension. In: Zanchetti A, Mancia G, editors. Handbook of hypertension. Amsterdam: Elsevier Science BV; 1997; p568–601.
- Bristow JD, Honour AJ, Pickering GW, Sleight P, Smyth HS. Diminished baroreflex sensitivity in high blood pressure. Circulation 1969;39:48-54.
- Su DF, Cerutti C, Barres C, Vincent M, Sassard J. Blood pressure and baroreflex sensitivity in conscious hypertensive rats of Lyon strain. Am J Physiol 1986;251:H1111-7.
- Jones JV, Floras JS. Baroreflex sensitivity changes during the development of Goldblatt two-kidney one-clip hypertension in rats. Clin Sci 1980;59:347-52.
- Moyes MR, Carbral AM, Marcal D, Vasquez EC. Sigmoidal curve-fitting of baroreceptor sensitivity in renovascular 2K1C hypertensive rats. Braz J Med Biol Res 1994;27:1419-24.
- Jones JV, Floras JS. Baroreflex sensitivity changes during the reversal of Goldblatt two-kidney one-clip hypertension in rats. Clin Exp Hypertens A 1982;4:903-14.
- Lu ZA, Xie HH, Xu LP, Yin AF, Miao CY, Su DF. Restoration of arterial baroreflex function contributes to organ protection in spontaneously hypertensive rats treated with long-term hydrochlorothiazide mixture. Clin Exp Pharmacol Physiol 2003;30:49-54.
- Du WM, Miao CY, Liu JG, Shen FM, Yang XQ, Su DF. Effects of long-term treatment with ketanserin on blood pressure variability and end-organ damage in spontaneously hypertensive rats. J Cardiovasc Pharmacol 2003;41:233-9.
- Parmer RJ, Cervenka JH, Stone RA. Baroreflex sensitivity and heredity in essential hypertension. Circulation 1992;85:497-503.
- Yamada Y, Miyajima E, Tochikubo O, Matsukawa T, Shionoiri H, Ishii M, et al. Impaired baroreflex changes in muscle sympathetic nerve activity in adolescents who have a family history of essential hypertension. J Hypertens Suppl 1988;6:S525-8.
- Shan ZZ, Dai SM, Su DF. Relationship between baroreceptor reflex function and end-organ damage in spontaneously hypertensive rats. Am J Physiol 1999;277:H1200-6.
- Miao CY, Su DF. The importance of blood pressure variability in rat aortic and left ventricular hypertrophy by sinoaortic denervation. J Hypertens 2002;20:1865-72.
- Su DF, Xu LP, Miao CY, Xie HH, Shen FM, Jiang YY. Two useful methods for evaluating antihypertensive drugs in conscious freely moving rats. Acta Pharmacol Sin 2004;25:148-51.
- Smyth HS, Sleight P, Pickering GW. Reflex regulation of arterial pressure during sleep in man: a quantitative method of assessing baroreflex sensitivity. Circ Res 1969;24:109-21.
- Su DF, Chen L, Kong XB, Cheng Y. Determination of arterial baroreflex-blood pressure control in conscious rats. Acta Pharmacol Sin 2002;23:103-9.
- Su DF, Cerutti C, Barres C, Julien C, Vincent M, Paultre C, et al. Arterial baroreflex control of heart period is not related to blood pressure variability in conscious hypertensive and normotensive rats. Clin Exp Pharmacol Physiol 1992;19:767-75.
- Hayakawa H, Raij L. The link among nitric oxide synthase activity, endothelial function, and aortic and ventricular hypertrophy in hypertension. Hypertension 1997;29:235-41.
- Miao CY, Xie HH, Wang JJ, Su DF. Candesartan inhibits sinoaortic denervation-induced cardiovascular hypertrophy in rats. Acta Pharmacol Sin 2002;23:713-20.
- Miao CY, Tao X, Gong K, Zhang SH, Chu ZX, Su DF. Arterial remodeling in chronic sinoaortic-denervated rats. J Cardiovasc Pharmacol 2001;37:6-15.
- Kimula K, Tojo A, Matsuoka H, Sugimoto T. Renal arteriolar diameters in spontaneously hypertensive rats: Vascular cast study. Hypertension 1991;18:101-10.
- Hendley ED, Atwater DG, Myers MM, Whitehorn D. Dissociation of genetic hyperactivity and hypertension in SHR. Hypertension 1983;5:211-7.
- Wickens JR, Macfarlane J, Booker C, McNaughton N. Dissociation of hypertension and fixed interval responding in two separate strains of genetically hypertensive rat. Behav Brain Res 2004;152:393-401.
- Yongue BG, Myers MM. Further evidence for genetic independence of blood pressure and salt appetite in spontaneously hypertensive and Wistar-Kyoto rats. Clin Exp Hypertens A 1989;11:25-33.
- Myers MM. Blood pressure development in F2 rats derived from SHR and WKY progenitors. Clin Exp Hypertens A 1991;13:573-86.
- Bachmanov AA. The characteristics of the F1 hybrids of spontaneously hypertensive and normotensive rats. Fiziol Zh SSSR Im I M Sechenova 1989;75:1714-7.
- Rapp JP. Genetic analysis of inherited hypertension in the rat. Physiol Rev 2000;80:135-72.
- Davidson AO, Schork N, Jaques BC, Kelman AW, Sutcliffe RG, Reid JL, et al. Blood pressure in genetically hypertensive rats. Influence of the Y chromosome. Hypertension 1995;26:452-9.
- Takami S, Higaki J, Miki T, Katsuya T, Nakata Y, Rakugi H, et al. Analysis and comparison of new candidate loci for hypertension between genetic hypertensive rat strains. Hypertens Res 1996;19:51-6.
- Harrap SB, Jones EF. Cardiovascular hypertrophy does not predispose to genetic hypertension. Clin Exp Hypertens 1997;19:531-41.
- Ely DL, Turner ME. Hypertension in the spontaneously hypertensive rat is linked to the Y chromosome. Hypertension 1990;16:277-81.
- Ely DL, Daneshvar H, Turner ME, Johnson ML, Salisbury RL. The hypertensive Y chromosome elevates blood pressure in F11 normotensive rats. Hypertension 1993;21:1071-5.
- Parmer RJ, Cervenka JH, Stone RA, O’Connor DT. Autonomic function in hypertension: Are there racial difference? Circulation 1990;81:1305-11.
- Eckberg DL. Carotid baroreflex function in young men with borderline blood pressure elevation. Circulation 1979;59:632-6.
- Xie P, Chapleau MW, McDowell TS, Hajduczok G, Abboud FM. Mechanism of decreased baroreceptor activity in chronic hypertensive rabbits. J Clin Invest 1990;86:625-30.
- Weinstock M, Korner PI, Head GA, Dorward PK. Differentiation of cardiac baroreflex properties by cuff and drug methods in two rabbit strains. Am J Physiol 1988;255:R654-64.