Differential regulation of cadherin expression by osteotropic hormones and growth factors in vitro in human osteoprogenitor cells
Introduction
Cell adhesion molecules (CAM) are believed to play a key role in morphogenesis by modulating cellular proliferation and differentiation through cell-cell interaction and/or cell-matrix interactions[1]. In bones, the differentiation of human bone marrow stromal cells (hBMSC) into mature osteoblasts is essential for bone formation[2]. However, the mechanisms by which CAM modulate this process are still poorly understood. Cadherins, a family of transmembrane glycoproteins, are calcium-dependent homophilic cell-cell adhesion molecules, which have been shown to play an important role in the differentiation of a wide variety of cells and tissues[3].
Cadherins are divided into subclasses, all of which share a common basic structure. Four subclasses have been well characterized at the molecular level and they have about 50% amino acid sequence identity. These include E-cadherin, P-cadherin, and N-cadherin[3]. The extent of conservation varies depending on the region of the molecule with the cytoplasmic domain being the most conserved. A monoclonal antibody to this cytoplasmic domain designated pan-cadherin MoAb was produced, which can be used to recognize novel cadherins in a variety of cells and tissues and displays a broad interspecies cross-reactivity[4]. The evidence that cadherin-mediated interactions can actually modulate cell differentiation has been accumulated[5]. For example, N-cadherin has been demonstrated to be present in the limb mesenchyme in a development-specific manner in vivo and in vitro. In addition, functional N-cadherin is necessary for limb mesenchymal cells to undergo condensation and progress through chondrogenesis in vitro and in vivo[6].
Cadherin has recently been found in the cells of osteoblastic lineage[7]. E-cadherin is expressed by the UMR 106-H5 rat osteoblastic osteosarcoma cells as well as by the mouse calvarial MC3T3-E1 cells, and its expression is regulated by PTH in the UMR 106-H5 cells[8]. E-cadherin also plays a role in the generation of multinucleated osteoclasts by mediating fusion of mononuclear cells in murine marrow[9]. In addition, specific osteoblast-like cadherin (OB-cadherin) has been found to be expressed in osteoblasts[10]. However, it remains unclear whether these or other ubiquitous cell adhesion molecules are involved in the differentiation of human osteoblast precursors, which are derived mainly from hBMSC[2]. For better understanding of osteoblast lineage differentiation, this study was to examine the cadherins expression pattern and their regulation by osteotropic agents including systemic hormones and local growth factors in hBMSC.
Materials and methods
Antibodies and chemicals Mouse monoclonal antibody to pan-cadherins (MoAb C-1821) (mouse IgG1 isotype) was purchased from Sigma Chemical Co (St Louis, USA); Mouse monoclonal antibody to human E-cadherin (MoAb 004FD) (mouse IgG1 isotype) was purchased from R & D Systems Europe Ltd (Oxon, UK). Recombinant human (rh)-tumor necrosis facotr (TGF)-β was a gift from Genentech Inc (CA, USA). Recombinant human basic fibroblast growth factor (bFGF) was a gift from Schering Research Laboratories (Berlin, Germany). Stock concentration: 1 mg/L and it was diluted into 1 μg/L in PBS containing 0.1% BSA and aliquots of this was stored at -70 ºC. 1,25(OH)2D3 was a gift from Hoffmann-La Roche, Inc (Nutley, NJ, USA). Dexamethasone (Dex), parathyroid hormone (PTH) and estradiol (E2) were purchased from Sigma Chemical Co. Other chemicals were purchased from Sigma Chemical Co.
Cultures of human bone marrow stromal cells Primary cultures of hBMSC were described previously[11]. Briefly, when the cells became confluent (3-4 weeks after seeding) in the primary cultures, they were subcultured onto glass coverslips, Petri dishes, or into flasks as necessary. At confluence, first passage cells were treated with 1,25(OH)2D3 (50 nmol/L), PTH (100 nmol/L), E2 (10 nmol/L), Dex (10 nmol/L), prostaglandin E2 (PGE2 10 nmol/L), bFGF (1 and 10 μg/L), and TGF-β (1 and 10 μg/L) for 7 d. The doses for the tested agents were selected based on our previous works and others were used for examining osteogenic differentiation of hBMSC[2,7,12–18]. The cell lysates were extracted with RIPA buffer containing 1% NP-40, 1% Triton X-100, 2 mmol/L CaCl2, 0.5% NaCl, 0.1% SDS in PBS with fresh protease inhibitors, including phenyl methyl sulfonyl fluoride (PMSF), pepstatin, 1,10-phenantroline and E-64.
Cultures of conditionally immortalized hBMSC hBMSC were transfected with a viral vector coding a temperature-sensitive mutant of the SV40 large T antigen. The growth characteristic of this immortalized hBMSC is controlled by this temperature-sensitive large T antigen. The large antigen expression was activated at 33 ºC and this allowed the cells to proliferate, producing a large population of homogenous hBMSC. While, the large T antigen was disabled at 39 ºC allowing these immortalized hBMSC to undergo osteogenic differentiation. One of nine clones obtained, hOP-7, was well characterized for osteogenic differentiation . These cells were induced to differentiate into osteoblasts by Dex and 1,25(OH)2D3 at 39 ºC. Therefore, this cell line is thought to be a good model for the study of osteogenic differentiation[19].
hOP-7 cells were seeded onto LabTek chamber slides and maintained for 1–2 d at 33 ºC before being switched to 39 ºC to allow osteogenic differentiation. In some cases, cultures were plated out initially at a higher density to allow formation of nodules. These were also maintained at 33 ºC and thereafter switched to 39 ºC for a further 5 d.
Immunolocalization of cadherin expression Cells on coverslips or on chamber slides fixed with 4% paraformaldehyde were incubated with 5% goat serum for 30 min to block non-specific binding, followed by incubation with a murine monoclonal anti-pan-cadherin antibody (MoAb C-1821) (diluted 1:200 or 1:500) or a mouse monoclonal antibody to human E-cadherin (MoAb 004FD) (diluted 1:200) for 1 h at room temperature. The mouse cavarial cell line MC3T3-E1 was used as a positive control for pan-cadherin immuno-staining[8], and MDCK cells for E-cadherin[20]. Mouse ascites fluid and PBS were used to substitute primary antibodies as negative controls. Then, these cells were washed with high salt buffer for 10 min, and twice with TBS for 15 min each. Thereafter, these cells were incubated with biotinylated secondary antibody for 45 min at room temperature and then washed as before. Immunoreactivity was detected using the Vectastain ABC-AP kit. Incubation was for 45 min at room temperature, followed by staining for alkaline phosphatase in the presence of 1 mmol/L levamisole, to block endogenous alkaline phosphatase. The cells were washed again with TBS and the nuclei was stained with DAPI. After the coverslips and chamber slides were mounted with Kaiser’s glycerol jelly, immunostaining was visualized under fluorescence-microscopy using a filter appropriate for rhodamine and another for ultraviolet, and then photographed. Cadherin antigenicity appears as a red coloration, while the nuclei are blue.
Histological staining for alkaline phosphatase (ALP) and biochemical determination of its activity in cultured hBMSC
Histochemical staining of ALP At the end of the culture period, cells on chamber slides were washed twice with PBS and fixed with 4% paraformaldehyde at 4 ºC for 30 min, washed three times with distilled water and then air-dried for 10 min. The cells were then stained for ALP for 30 min with fresh naphthol AS-MX phosphatase solution (0.2 g/L) containing fast red violet B salt (0.42 g/L) in 100 mmol/L Tris-HCl (pH 0.9) at room temperature and then photographed. Under these conditions, ALP-positive cells were stained pink or red.
Biochemical determination of ALP activity At the end of the incubation period, the culture medium was removed from wells, and the cell layers were washed three times with ice cold PBS (pH 7.4). The cells were scraped off the plates into 300–500 µL ice-cold 0.1% Triton-X100 using a ‘rubber policeman’. The cell lysates were transferred into plastic tubes (LP4) on ice and subjected to mild sonication (3×5 s bursts, peak amplitude 10 µm at a frequency of 20 kHz) to completely solubilize these cells. The cell lysates were stored at -20 ºC until they were assayed. ALP activity was measured using 2 mmol/L para-nitrophenyl phosphate (p-NPP) in assay buffer (0.1 mol/L diethanolamine+1 mmol/L MgCl2+2 mmol p-NPP, pH 10.5) at 37 ºC for 10–60 min. Protein content was determined using the Bio-Rad protein assay kit using BSA as a standard according to the manufacturer’s manual. ALP activity was expressed as μmol⋅h-1⋅g-1 protein.
Western blot analysis At the end of incubation with the various hormones and/or growth factors, media were removed from Petri dishes and cells were washed with PBS at room temperature, after which the cells were scraped into RIPA buffer (PBS, 1% NP40, 0.5% sodium deoxycholate, 0.1% SDS, 2 mmol/L CaCl2) containing fresh protease inhibitors on ice. Cell lysates were then passed repeatedly through a 21-gauge needle, incubated for 30 min on ice, and then centrifuged for 20 min at 4 ºC. Thereafter, the supernatants were harvested and the protein content was determined using the Bio-Rad protein assay.
Protein 10 μg was loaded into each lane, separated on a 7.5% SDS polyacrylamide gel and transferred onto nitrocellulose membrane in transblotting buffer containing 20 mmol/L Tris, 150 mmol/L glycine, and 20% methanol (pH 8.0). The membranes were blocked with blocking buffer consisting of 1% non-fat dry milk, 1% BSA, 0.05% Tween-20 in TBS buffer for 30 min and immunoblotted with the MoAb C-1821 diluted 1:3000 in blocking solution (Blotto B) for 45 min at room temperature. Thereafter, the membranes were washed three times, incubated with biotin-conjugated rabbit anti-mouse Ig G for 45 min, followed by incubation with streptavidin-conjugated horseradish peroxidase (HRP) for 30 min.
At the end of this incubation, the membranes were washed again three times and incubated with Amersham enhanced chemiluminesence (ECL) reagents for exactly 1 min. Excess ECL reagents were removed and the membranes were sealed in plastic wrap and exposed to ECL hyperfilms in a darkroom for 30 s. Then, the films were developed as for autoradiograph. Finally, the intensity of the two major cadherin bands on the hyperfilms was analyzed by scanning laser densitometry (discovery pDi densitometer, Pharmacia, Uppsala, Sweden). The intensity of control bands is expressed as 100%, and the others are expressed as percentage of respective control bands.
Membranes were also stripped of bound antibodies and re-probed by submerging them in stripping buffer (100 mmol/L 2-mercaptoethanol, 2% SDS, 62.5 mmol/L Tris-HCl, pH 6.7) and incubating at 50 °C for 30 min with occasional agitation. Then, the membranes were washed twice with TBS-Tween-20 at room temperature for 10 min. Following this, the membranes were blocked in blocking solution for 1 h after which immunodetection of HECD was performed using MoAb 004FD as described previously.
Statistical analysis Results of representative experiments are presented except where otherwise indicated. Biochemical determinations of ALP were performed in triplicate or quadruplicate and specific enzyme activity were presented as mean±SEM.
Statistical significance of difference within experiments was assessed using one factor analysis of variance (one way ANOVA). Values of P refer to a comparison of measured activity with that of control.
Results
Detection of cadherins expression in human bone marrow stromal cells Immunolocalization was performed to determine whether human bone-derived cells expressed cadherins using MoAb C-1821 in first passaged hBMSC and in hOP-7. Under fluorescence microscopy, there was no staining for cadherins in the negative controls (such as mouse ascites fluid and PBS), while MC3T3-E1 cells were found to be stained for cadherin expression as a positive control (Figure 1B). Interestingly, strong cadherin expression was observed in first passaged hBMSC (Figure 1D) and hOP-7 (Figure 1F).
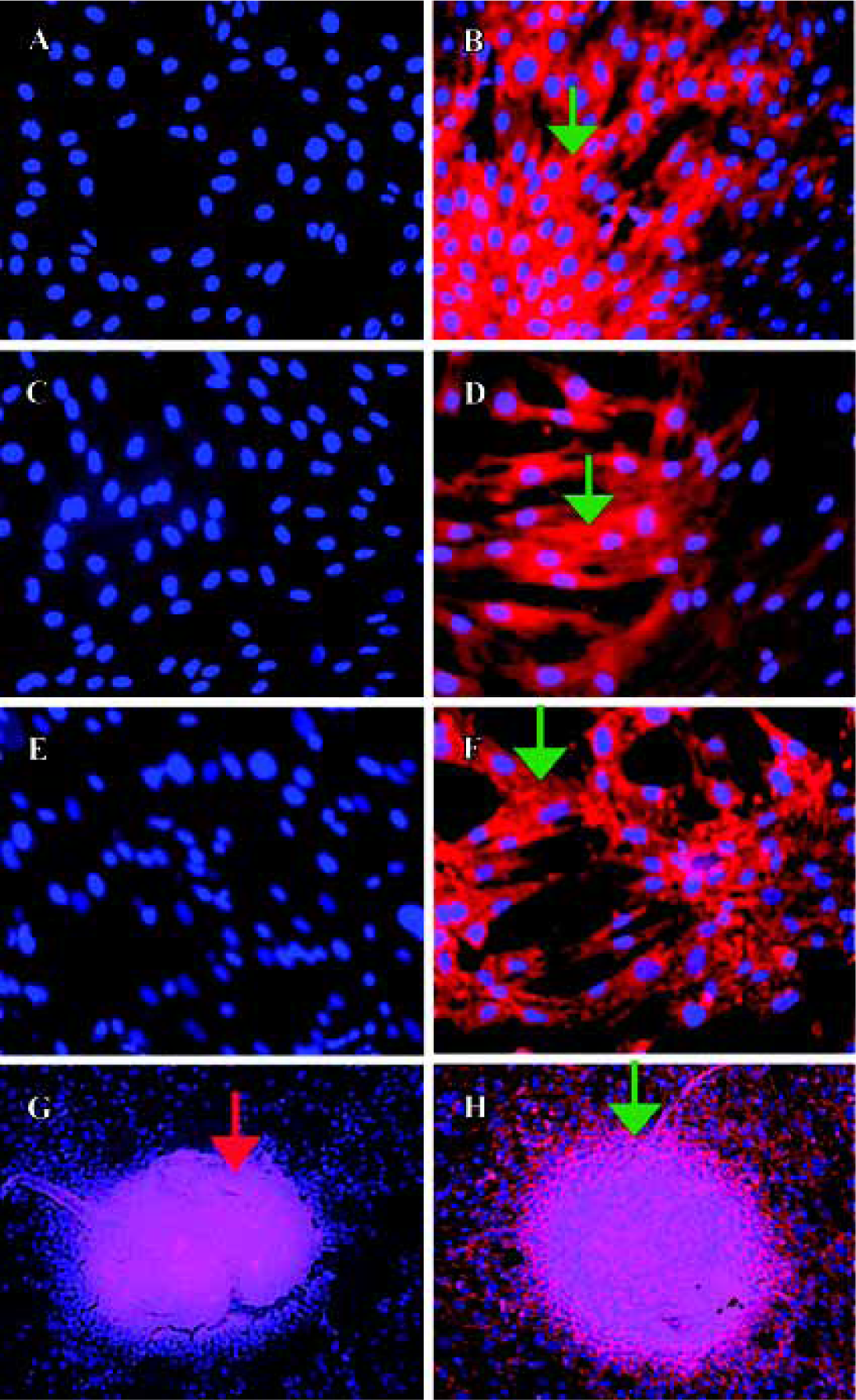
In the high-density cultures of hOP-7 cells, discrete nodules were formed and there were focal areas of intense immunostaining for cadherins in these nodules. However, staining at the periphery of the nodules was much weaker than at the center of the nodules. In addition, there was positive staining for ALP in the center of these nodules, while sparse ALP staining at the periphery of the nodules. This suggests an association between the expression of cadherins and ALP (Figure 1G and H), in which a close interaction of cells at the center of the nodules stimulates osteoblast differentiation. Human E-cadherin (HECD) expression was confirmed in the positive control cells, MDCK cells. However, HECD was not detectable by immunolocalization in hBMSC (Figure 2D). To clarify the expression of cadherins in these cells identified by immmunolocalization as described above, Western blotting was performed using MoAb C-1821 in untreated hBMSC extracts and this revealed the presence of two bands (139.7 kDa and 118.6 kDa), associated with several small bands (Figure 3B).
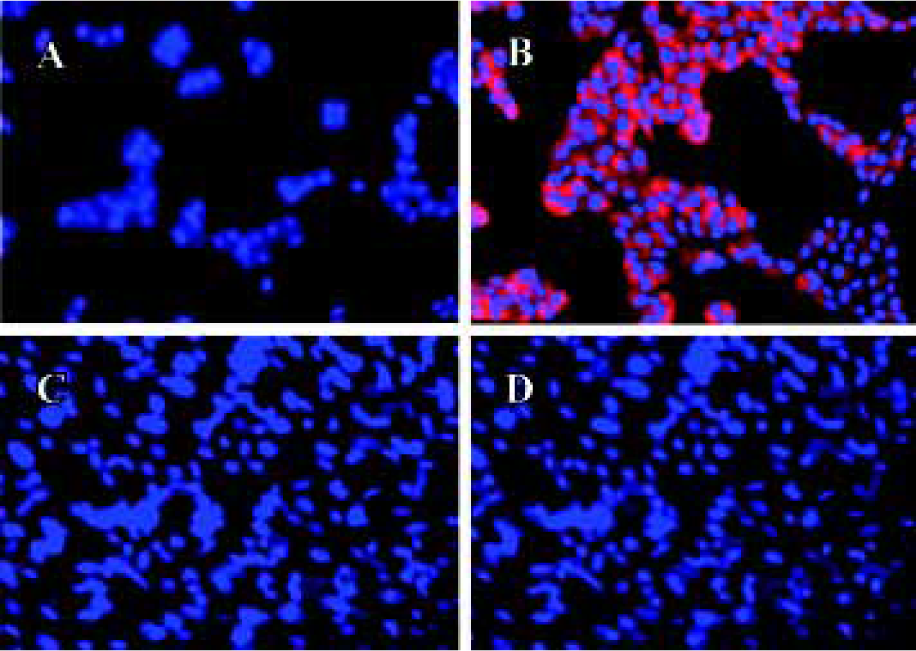
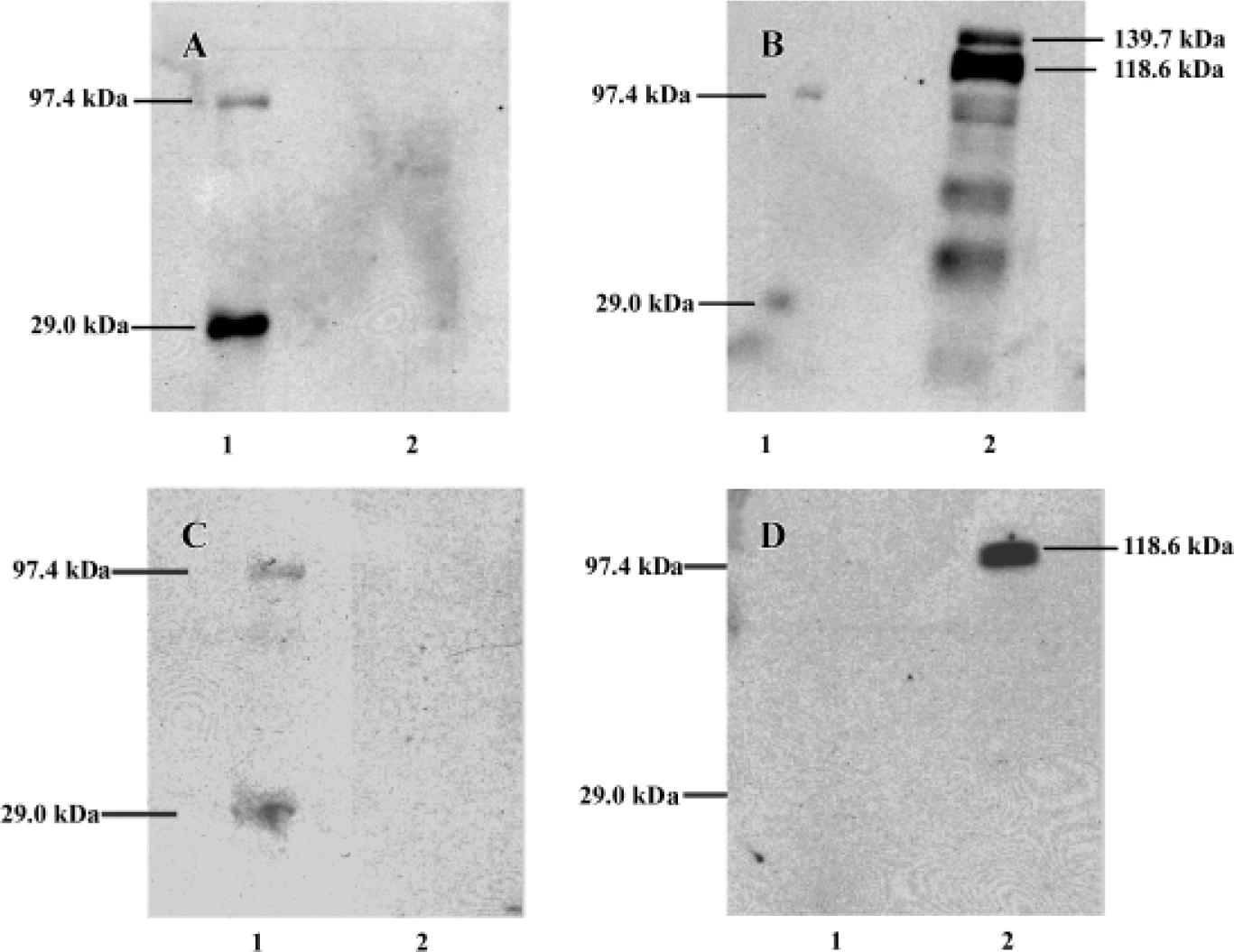
After being stripped, the membranes previously used for detection of cadherins were immunoblotted again with mouse anti-human E-cadherin (MoAb 004FD). Although human E-cadherin was not detectable in hBMSC by immunocytochemistry using MoAb 004FD, it was demonstrated by Western blot using the same antibody (MoAb 004FD) and there was only one band with a Mr of approximately 118.6 kDa, which was corresponding to the major band detected using anti-pan-cadherin antibody and was different from that of cadherins (Figure 3D). This indicates that MoAb 004FD specifically recognizes human E-cadherin.
Regulation of cadherins expression by osteotropic hormones and local growth factors hBMSC were treated with different agents for 7 d and the cell lysates were examined by Western blot. Using the ECL system, two major bands of cadherins with Mr 139.7 kDa and 118.6 kDa were examined using MoAb C-1821. These two bands were diminished in the 50 nmol/L 1,25(OH)2D3-treated cellular extracts, in comparison to the control (Figure 4A). Similarly, in Dex-treated-cellular extracts, the two major bands were also abolished (Figure 4B). This suggests that 1,25(OH)2D3 and Dex inhibit cadherin expression. With regards to 10 nmol/L PGE2, band a (Mr about 139.7 kDa) was inhibited, but band b (Mr about 118.6 kDa) was increased nearly 1.5-fold. The expression of cadherins was also found an increase by PTH, E2, bFGF, and TGF-β. All these agents increased band a more than band b in comparison to respective controls. PTH increased band a by more than 5-fold relative to the control and increased band b by only 1-fold. TGF-β enhanced band a by up to 3-fold and slightly stimulated band b. bFGF increased band a by up to 2-fold and also slightly enhanced band b (Figures 4C and D).
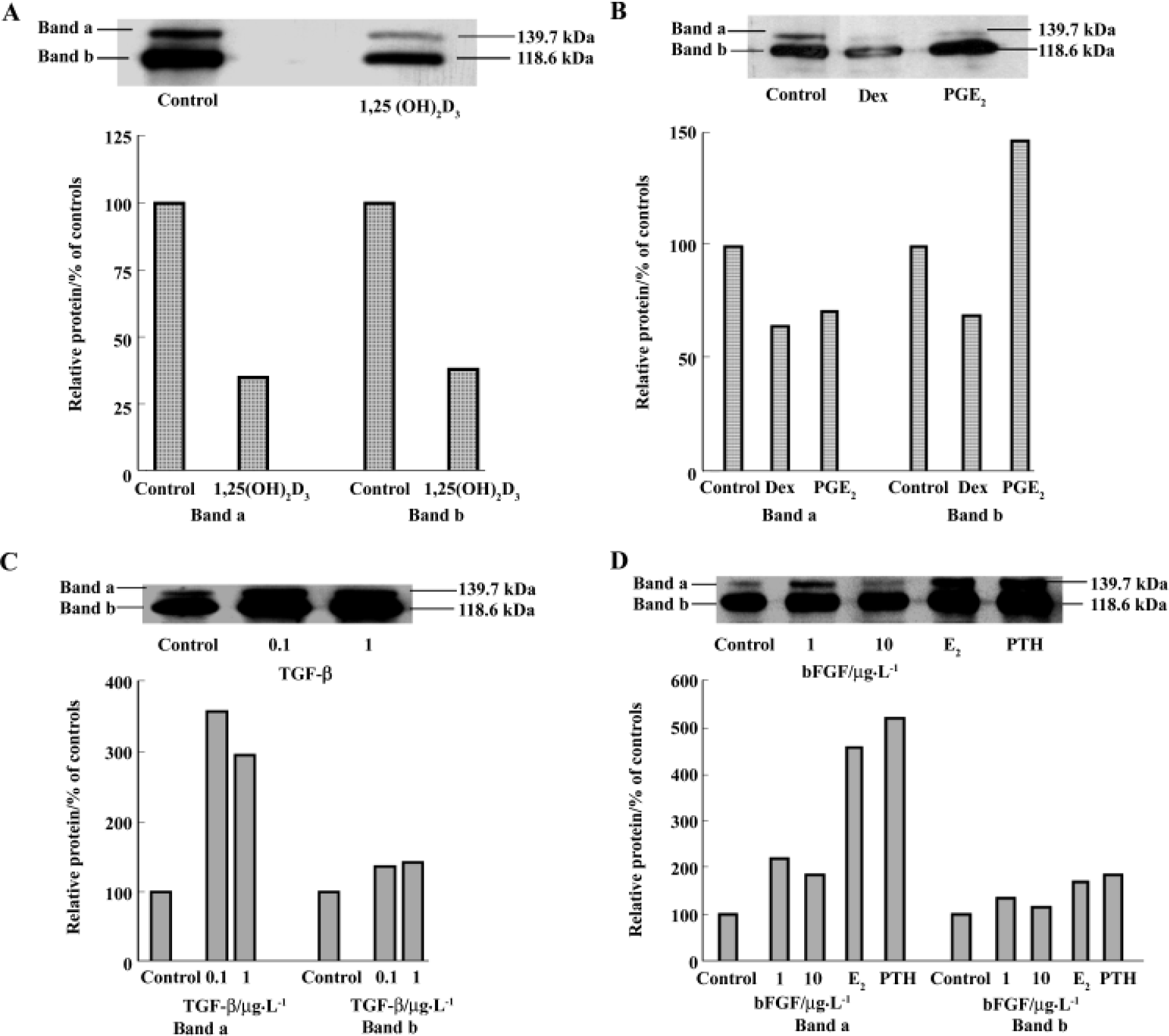
Effect of pan-cadherin MoAb on ALP activity To investigate a possible role of cadherins in the osteogenic differentiation of hBMSC, cadherins were blocked with MoAb C-1821 and ALP activity was examined. In these experiments, treatment of hBMSC with MoAb C-1821 for 48 h increased the level of ALP activity to about 125.9% of control (P<0.05 vs control), and it also enhanced the effect of 1,25(OH)2D3 on ALP activity (P<0.05 in experiment 1 and P<0.01 in experiment 2, compared to 1,25(OH)2D3-treated cells) (Figure 5).
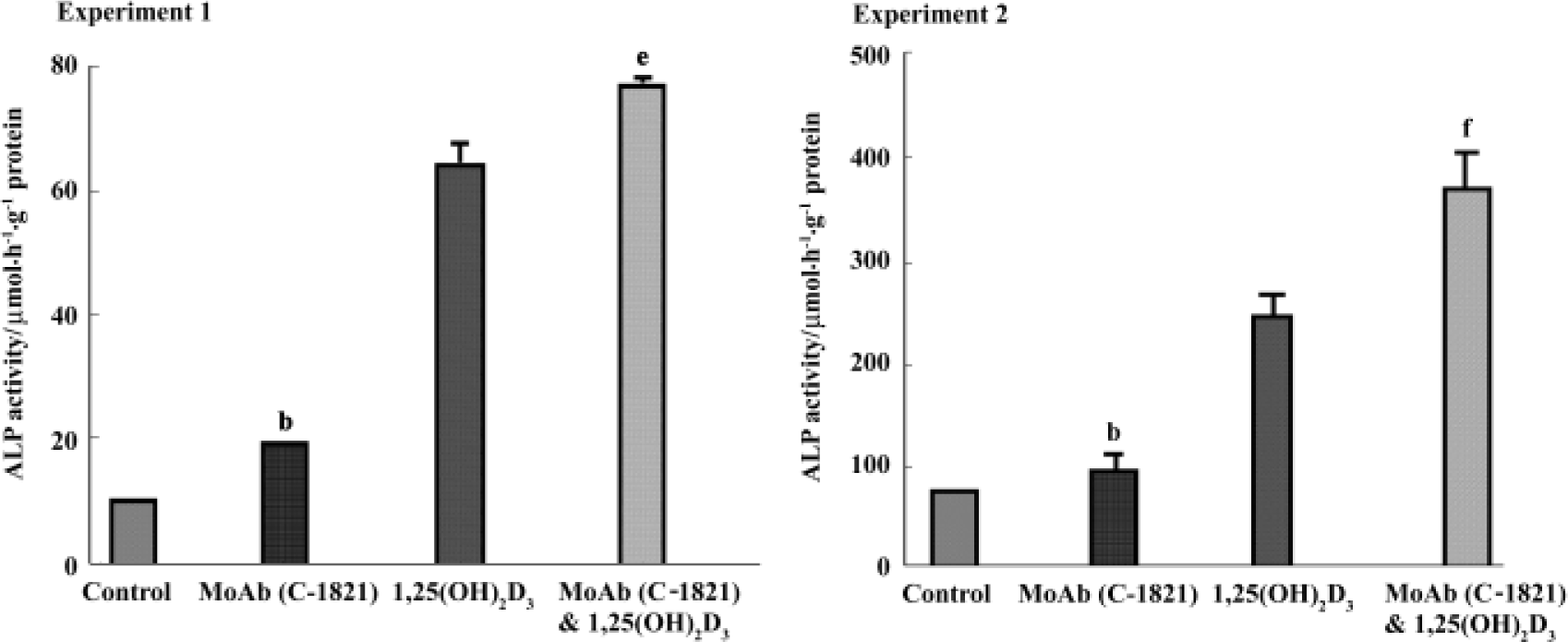
Discussion
There is a repertoire of cadherins expressed in osteoblasts. There are several subclasses of cadherins previously reported in cells of the osteoblast lineage, including E-cadherin[8], cadherin-4, cadherin-8, cadherin-11, protocadherin-43[14], and specific osteoblast-cadherin (OB-cadherins)[10]. In the present study, we demonstrated that cadherins were expressed in human osteoprogenitors. Cadherin expression was found to be associated with that of ALP in higher density cultures of hOP-7 cells. Furthermore, their expressions are regulated by osteotropic agents. These suggest that cadherins are involved in the process of osteogenic differentiation and some of the effects of these agents on bone are mediated, in part, via regulation of cadherins and possibly other cell adhesion molecules.
In the immunoblotting experiments, there were two major bands identified in hBMSC with molecular weights approximately 139.7 kDa and 118.6 kDa, along with several small bands using the anti-pan cadherin MoAb C-1821 (Figure 1), and this observation was consistent with a previous report using the same MoAb[4]. There was only one band with a Mr of 118.6 kDa using anti-human E-cadherin MoAb 004FD, which was in close agreement with human E-cadherin size (120 kDa)[21]. In this case, one of two major bands demonstrated by MoAb C-1821 may be human E-cadherin, another may be other cadherins, for example, osteoblast-like cadherin or N-cadherin, which was demonstrated to be specific for osteoblasts and its expression in the osteoblastic cells was up-regulated during differentiation, indicating an important role in osteogenic differentiation[10]. The results presented here are supported by a previous report in osteoblastic UMR106-H5 rat osteosarcoma cells[8]. It is necessary to determine whether all the polypeptides identified by the anti-pan cadherin MoAb C-1821 represent cadherin products encoded by distinct genes. Although roles of N-cadherin and osteoblast-like cadherins remains unknown, our data and others strongly suggest they are important regulators of osteoblast differentiation and osteogenesis and play a role in normal and pathological bone formation[8,10,13–18,22].
On the basis of detecting cadherin expression in hBMSC, we investigated the regulation of cadherin expression by osteotropic hormones and local growth factors. All of these factors are thought to play important roles in bone remodeling[23]. Up until now, little was known of the role of cadherins in bone remodeling, particularly in osteogenic differentiation and whether osteotropic hormones and local growth factors regulate the expression of cadherin. With treatments of hBMSC with various hormones and growth factors for 7 d, it was observed that both 1,25(OH)2D3 and dexamethasone inhibited the expression of cadherins. In contrast, PTH, PGE2, estradiol, bFGF, and TGF-β increased the expression. However, these agents are found to differentially modulate these two bands. In these experiments, band a was enhanced more by certain agents than band b, and PGE2 inhibited band a, but increased band b. These results suggest that there may be more than one subclass of cadherins expressed in hBMSC and osteotropic hormones and growth factors may differentially regulate these individual cadherins. Certain factors, such as TGF-β, PTH, and E2, had more specific effects on Band a, and these two bands may represent different cadherin expressions. PTH was shown to increase the expression of E-cadherin in mouse MC3T3-E1 osteoblastic cells[8] and increase mRNA level of the neural-cadherin-like adhesion molecule (N-CAD) in vitro and in vivo[15]. Dexamethasone was shown to decrease N-CAD and to completely inhibit cadherin mRNA in rats[15]. In addition, dexamethasone was also found to increase steady state mRNA for cadherin-4, but decrease mRNA expression for N-cadherin[14]. However, steady-state mRNA expression for protocadherin and cadherin-11 was not affected by dexamethasone[14]. Estradiol was shown to cause a rapid and significant increase in the ovarian E- and N-cadherin mRNA levels in mice in vivo[24]. It was reported that TGF-β up-regulated N-cadherin expression at mRNA level in mesenchymal cells obtained from early chick limb bud, which can differentiate into chondrocytes when plated at a high density[25].
It was known that osteogenic differentiation of hBMSC was stimulated by 1,25(OH)2D3[12] and dexamethasone[11], and the proliferation of these cells is inhibited by the two agents[11,12]. PTH was shown to be mitogenic for human osteoblast-like cells[26]. Estradiol stimulates the proliferation of osteoblast-like cells derived from explants of rat trabecular bone and the immortalized rat calvarial RCT-3 cell line[27], mouse MC3T3-E1 osteoblastic cells[28] and human osteoblastic osteosarcoma SAOS-2 cell line[29]. The local factor PGE2 was demonstrated to stimulate the proliferation of bone cells[30]. In addition, TGF-β and bFGF inhibit osteogenic differentiation of hBMSC and stimulate cell proliferation[12,31]. Taken together, it seems likely that the agents that promote osteogenic differentiation and inhibit cell proliferation may decrease certain cadherin expression, while other agents that inhibit osteogenic differentiation and stimulate cell proliferation may increase certain cadherin expressions, indicating that an increase in cadherin expression is associated with cell proliferation and a decrease in cadherin expression with osteogenic differentiation at a ‘specific stage’ of differentiation.
Because the C-terminal regions of cadherins are essential domains for function, and the anti-pan cadherin MoAb C-1821 recognizes this site, this MoAb was used as a function-perturbing antibody for examining a possible role for cadherins in osteogenic differentiation of hBMSC. A perturbing function of the cadherins with the MoAb caused the fibroblast-like hBMSC to become more or less ploygonal observed under microscope (data not shown here). It was also observed that this block with the MoAb significantly increased alkaline phosphatase activity, and enhanced 1,25(OH)2D3-induced increase in ALP activity in which first passaged hBMSC were induced for osteogenic differentiation. This indicates that cadherins may play a role in differentiation of hBMSC. Based on 1:500 dilution used in immuno-localization described previously, a 1:200 dilution was used in these experiments. It remains possible that ALP activity may be increased more if higher concentrations of the MoAb are used. However, the MoAb C-1821 is expensive, so reasonable dilution 1:200 was applied to the experiments. As mentioned above, 1,25(OH)2D3 inhibited the cadherin expression in hBMSC and 1,25(OH)2D3 promoted osteogenic differentiation[12], including enhancement of ALP activity and osteocalcin expression. These suggest that the role of 1,25(OH)2D3 on osteoblast differentiation may be partially through mediating the cadherin expression. There may be other cell adhesion molecules involved in the mediation of 1,25(OH)2D3-induced effect on ALP activity. A role for E-cadherin in osteogenic differentiation had also been indicated in the fetal rat calvarial (FRC) osteoblast system. In these FRC cultures, treatment of FRC osteoblasts with polyclonal antibodies to murine E-cadherin significantly increased mineralized bone nodule formation[32]. Taken together, these data indicate that some specific cadherins may negatively regulate osteogenic differentiation at a ‘specific stage’ during osteogenic differentiation.
In conclusion, the effects of osteotropic hormones and local growth factors on osteogenic differentiation of hBMSC may be, at least, partially via regulation of cell adhesion molecules, such as cadherins. Cadherins may function as mediators of transition between proliferation and differentiation and then negatively regulate osteogenic differen-tiation.
References
- Edelman GM, Crossin KL. Cell adhesion molecules: implications for a molecular histology. Annu Rev Biochem 1991;60:155-90.
- Aubin JE. Advances in the osteoblast lineage. Biochem Cell Biol 1998;76:899-910.
- Takeichi M. Cadherin cell adhesion receptors as a morphogenetic regulator. Science 1991;251:1451-5.
- Geiger B, Volberg T, Ginsberg D, Bitzur S, Sabanay I, Hynes RO. Broad spectrum pan-cadherin antibodies, reactive with the C-terminal 24 amino acid residues of N-cadherin. J Cell Sci 1990;97:607-14.
- Geiger B, Ayalon O. Cadherins. Annu Rev Cell Biol 1992;8:307-32.
- Oberlender SA, Tuan RS. Spatiotemporal profile of N-cadherin expression in the developing limb mesenchyme. Cell Adhes Commun 1994;2:521-37.
- Ahdjoudj S, Lasmoles F, Holy X, Zerath E, Marie PJ. Transforming growth factor beta2 inhibits adipocyte differentiation induced by skeletal unloading in rat bone marrow stroma. J Bone Miner Res 2002;17:668-77.
- Babich M, Foti LR. E-cadherins identified in osteoblastic cells: effects of parathyroid hormone and extracellular calcium on localization. Life Sci 1994;54:PL201-8.
- Mbalaviele G, Chen H, Boyce BF, Mundy GR, Yoneda T. The role of cadherin in the generation of multinucleated osteoclasts from mononuclear precursors in murine marrow. J Clin Invest 1995;95:2757-65.
- Okazaki M, Takeshita S, Kawai S, Kikuno R, Tsujimura A, Kudo A, et al. Molecular cloning and characterization of OB-cadherin, a new member of cadherin family expressed in osteoblasts. J Biol Chem 1995;269:12092-8.
- Cheng SL, Yang JW, Rifas L, Zhang SF, Avioli LV. Differentiation of human bone marrow osteogenic stromal cells in vitro: induction of the osteoblast phenotype by dexamethasone. Endocrinology 1994;134:277-6.
- Liu P, Oyajobi BO, Russell RG, Scutt A. Regulation of osteogenic differentiation of human bone marrow stromal cells: interaction between transforming growth factor-beta and 1,25(OH)(2) vitamin D(3) in vitro. Calcif Tissue Int 1999;65:173-80.
- Castro CH, Shin CS, Stains JP, Cheng SL, Sheikh S, Mbalaviele G, et al. Targeted expression of a dominant-negative N-cadherin in vivo delays peak bone mass and increases adipogenesis. J Cell Sci 2004;117:2853-4.
- Cheng SL, Lecanda F, Davidson MK, Warlow PM, Zhang SF, Zhang L, et al. Human osteoblasts express a repertoire of cadherins, which are critical for BMP-2-induced osteogenic differentiation. J Bone Miner Res 1998;13:633-4.
- Ferrari SL, Traianedes K, Thorne M, Lafage-Proust MH, Genever P, Cecchini MG, et al. A role for N-cadherin in the development of the differentiated osteoblastic phenotype. J Bone Miner Res 2000;15:198-208.
- Kawaguchi J, Azuma Y, Hoshi K, Kii I, Takeshita S, Ohta T, et al. Targeted disruption of cadherin-11 leads to a reduction in bone density in calvaria and long bone metaphyses. J Bone Miner Res 2001;16:1265-71.
- Marie PJ, Debiais F, Hay E. Regulation of human cranial osteoblast phenotype by FGF-2, FGFR-2, and BMP-2 signaling. Histol Histopathol 2002;17:877-85.
- Marie PJ. Role of N-cadherin in bone formation. J Cell Physiol 2002;190:297-305.
- Houghton A, Oyajobi BO, Foster GA, Russell RG, Stringer BM. Immortalization of human marrow stromal cells by retroviral transduction with a temperature sensitive oncogene: identification of bipotential precursor cells capable of directed differentiation to either an osteoblast or adipocyte phenotype. Bone 1998;22:7-16.
- Vandenbossche GM, De Bruyne GK, Bruyneel EA, Clemminck G, Vleminckx K, et al. Micro-encapsulation of MDCK-ras-e cells prevents loss of E-cadherin invasion-suppressor function in vivo. Int J Cancer 1994;57:73-80.
- Wheelock MJ, Knudsen KA. Cadherins and associated proteins. In Vivo 1991;5:505-13.
- Nagahata M, Tsuchiya T, Ishiguro T, Matsuda N, Nakatsuchi Y, Teramoto A, et al. A novel function of N-cadherin and connexin 43: marked enhancement of alkaline phosphatase activity in rat calvarial osteoblast exposed to sulfated hyaluronan. Biochem Biophys Res Commun 2004;315:603-11.
- Canalis E. Insulin like growth factors and the local regulation of bone formation. Bone 1993;14:273-6.
- MacCalman CD, Farookhi R, Blaschuk OW. Estradiol regulates N-cadherin mRNA levels in the mouse ovary. Dev Genet 1995;16:20-4.
- Tsonis PA, Del Rio-Tsonis K, Millan JL, Wheelock MJ. Expression of N-cadherin and alkaline phosphatase in chick limb bud mesenchymal cells: regulation by 1,25-dihydroxyvitamin D3 or TGF-beta 1. Exp Cell Res 1994;213:433-7.
- MacDonald BR, Gallagher JA, Russell RG. Parathyroid hormone stimulates the proliferation of cells derived from human bone. Endocrinology 1986;118:2445-9.
- Ernst M, Heath JK, Rodan GA. Estradiol effects on proliferation, messenger ribonucleic acid for collagen and insulin-like growth factor-I, and parathyroid hormone-stimulated adenylate cyclase activity in osteoblastic cells from calvariae and long bones. Endocrinology 1989;125:825-33.
- Masuyama A, Ouchi Y, Sato F, Hosoi T, Nakamura T, Orimo H. Characteristics of steroid hormone receptors in cultured MC3T3-E1 osteoblastic cells and effect of steroid hormones on cell proliferation. Calcif Tissue Int 1992;51:376-81.
- Slootweg MC, Ederveen AG, Schot LP, Schoonen WG, Kloosterboer HJ. Oestrogen and progestogen synergistically stimulate human and rat osteoblast proliferation. J Endocrinol 1992;133:R5-8.
- Somjen D, Waisman A, Weisman J, Kaye AM. Nonhypercalcemic analogs of vitamin D stimulate creatine kinase B activity in osteoblast-like ROS 17/2.8 cells and up-regulate their responsiveness to estrogens. Steroids 1998;63:340-3.
- Pri-Chen S, Pitaru S, Lokiec F, Savion N. Basic fibroblast growth factor enhances the growth and expression of the osteogenic phenotype of dexamethasone-treated human bone marrow-derived bone-like cells in culture. Bone 1998;23:111-7.
- Kato Y, Boskey A, Spevak L, Dallas M, Hori M, Bonewald LF. Establishment of an osteoid preosteocyte-like cell MLO-A5 that spontaneously mineralizes in culture. J Bone Miner Res 2001;16:1622-33.