Electrophysiological actions of cyclosporin A and tacrolimus on rat hippocampal CA1 pyramidal neurons1
Introduction
Ca2+/calmodulin-dependent protein phosphatase 2B (calcineurin) is widely distributed within the brain with the highest levels in the hippocampus and striatum[1,2]. It has been found that calcineurin regulates ion channel activities, neurotransmitter release, synaptic plasticity, and gene transcription through dephosphorylation of a variety of target proteins[2,3]. Calcineurin-mediated dephosphorylation plays important roles not only in normal neuronal functions, but also in pathological processes in the brain[1,4-6]. For instance, both ischemic insults and kainate-induced seizures were demonstrated to cause Ca2+-dependent activation of calcine-urin, which resulted in surface translocation of the Kv2.1 channel in rat cortical neurons and marked enhancement of the delayed rectifier K+ current (IK)in cultured rat hippocampal neurons[5,6]. The changes were thought to be a novel compensatory mechanism, which suppresses neuronal hyperexcitability and excitotoxicity in the pathological conditions.
Immunosuppressant drugs cyclosporin A (CsA) and tacrolimus (FK506) are the specific inhibitors of calcineurin. The drugs were found to bind to immunophilins cyclophilin A and FKBP12, respectively, within neurons. Both the CsA/cyclophilin A and FK506/FKBP12 complexes specifically inhibit the phosphatase activity of calcineurin[7]. Nowadays, the drugs are routinely used as research tools to elucidate the functional roles of calcineurin in the brain. Thus far, however, interests have been focused on their actions on individual target proteins, such as ion channels, G protein-coupled receptors, and other proteins[2,3]. It is unclear whether and how the drugs affect the excitability of neurons in the brain. The aim of the present study was to investigate the actions of CsA and FK506 on the intrinsic membrane properties of CA1 pyramidal neurons in rat hippocampal slices. The ionic basis underlying the actions was further addressed in acutely dissociated hippocampal neurons.
Materials and methods
Materials CsA and other reagents were purchased from Sigma-Aldrich China. FK506 was kindly provided by the representative of Fujisawa Pharmaceutical in Shanghai. CsA and FK506 were dissolved in absolute ethyl alcohol to prepare stock solutions, with a concentration of 10 mmol/L, which were diluted to the desired concentrations before use. The concentration of ethanol in the final dilution was less than 0.1% and had no observed effect on the membrane properties and voltage-activated K+ currents of hippocampal CA1 pyramidal neurons.
Experiments on hippocampal slices Sprague-Dawley rats (5–9 d old) were obtained from the Shanghai Experimental Animal Center, Chinese Academy of Sciences (Shanghai, China). Transverse hippocampal slices (400 µm) were cut in ice-cold artificial cerebrospinal fluid (ACSF) using a M752 vibroslice (Campden Instruments, UK). The ACSF contained the following (in mmol/L): 125 NaCl, 1.25 KCl, 1.25 KH2PO4, 2 CaCl2, 1 MgCl2, 26 NaHCO3, and 10 glucose, bubbled with a gas mixture (95% O2 /5% CO2). The slices were incubated at 24–25 ℃ at least for 1 h, and then transferred to a submerged recording chamber (Medical System, USA) perfused with the ACSF at a rate of 2 mL/min at 30–32 ℃. Recording electrodes (a tip resistance of 3–5 MΩ) were pulled from borosilicate glass pipettes (Sutter Instruments, USA), and filled with a standard pipette solution containing the following (in mmol/L): 140 KCl, 2 MgCl2, 1 CaCl2, 10 HEPES, and 10 EGTA 10 (pH 7.3 with KOH). As previously described[8], the membrane potential of CA1 pyramidal neurons was monitored using whole-cell current clamp recoding with an Axoclamp 2B amplifier (Axon Instruments, USA). Input resistance was calculated as the slope of the current-voltage (I-V) curve between the current amplitudes of -50 and +50 pA[9]. Depolarizing current pulses with a 300 ms duration were injected to elicit a train of action potentials once every 30 s. The first action potential in each train was used to compare the action potential shape. Signals were filtered at 2 kHz and sampled at frequencies of 10–40 kHz using pClamp 9.0 software via a DigiData-1322A interface (Axon Instruments, USA). FK506- and CsA-containing ACSF was delivered through perfusion.
Experiments on dissociated hippocampal neurons Transverse hippocampal slices (500 µmol/L) were cut in oxygenated ice-cold dissociation solution containing the following (in mmol/L): 82 Na2SO4, 30 K2SO4, 5 MgCl2, 10 HEPES, and 10 glucose (pH 7.3). The slices were incubated in the solution containing protease XXIII (3 g/L) for 8 min at 32 ℃ and then placed in the solution containing trypsin inhibitor type II-S (1 g/L) and bovine serum albumin (1 g/L) under an oxygen atmosphere at 24–25 ℃. The slices remained viable at least for 5–6 h. When neurons were needed, the CA1 regions were dissected from 3–4 slices and triturated using a series of fire-polished Pasteur pipettes with decreasing tip diameters. Dissociated neurons were placed in a recording dish and superfused with a standard external solution containing the following (in mmol/L): 135 NaCl, 5 KCl, 1 CaCl2, 2 MgCl2, 10 HEPES, 10 glucose, and 0.001 tetrodotoxin (pH 7.3 with NaOH) at 24–25 ℃. Whole-cell voltage-clamp recording was made from large pyramidal-shaped neurons using an Axopatch 200A amplifier (Axon Instruments, USA). Voltage protocols were provided by pClamp 9.0 software. Series resistance was compensated by 75%–85%. Linear leak and residual capacitance currents were subtracted online using a P/4 protocol.
CsA-containing external solution was delivered to the neuron using RSC-100 rapid solution changer with a 9-tube head (BioLogic, France). For the intracellular dialysis, CsA in the pipette solution was diffused into the recorded neuron immediately after the patch membrane ruptured[10]. The low Ca2+ external solution was similar to the standard external solution, except that the concentration of CaCl2 was reduced to 0.25 mmol/L and nifedipine (5 µmol/L) was included. The composition of the 0 Ca2+ pipette solution was similar to that of the standard one, but CaCl2 was replaced with equimolar MgCl2.
Data analysis Data are presented as mean±SEM. The duration of the action potential was measured at half-maximal spike amplitude (half-height width). The amplitude of IK was measured at 300 ms latency. For analyzing the voltage-dependence of steady-state activation or inactivation of the IK, normalized conductance or current was plotted against the membrane potential and fitted to the Boltzmann equation: Y=1/(1+exp[{V-V1/2}/k]), where Y is the normalized conductance or current, V is the test potential, V1/2 is the voltage at half-maximal activation or inactivation, and k is the slope factor. The time course of the recovery of IK from inactivation was fitted with a mono-exponential function: I/Imax=A*(1-exp[-Δt/τ]), where Imax is the maximal current amplitude, I is the current after a recovery period of Δt, τ is the time constant, and A is the amplitude coefficient.
Statistical significance was assessed using a Student’s paired or unpaired t-test or ANOVA, as appropriate. P<0.05 was considered significant. All analyses were performed using the Prism 3.0 software.
Results
Effects of FK506 and CsA on intrinsic membrane pro-perties of hippocampal neurons Perfusion with CsA (100 µmol/L) or FK506 (50 µmol/L) for 10 min did not significantly alter the membrane potential and input resistance of the neurons tested (Figure 1). The pooled data from the groups of neurons are presented in Table 1. The drugs did not cause a significant change in the firing pattern of CA1 pyramidal neurons in response to depolarizing current pulses (n=7 for CsA and n=6 for FK506; Figure 2A, 2B).
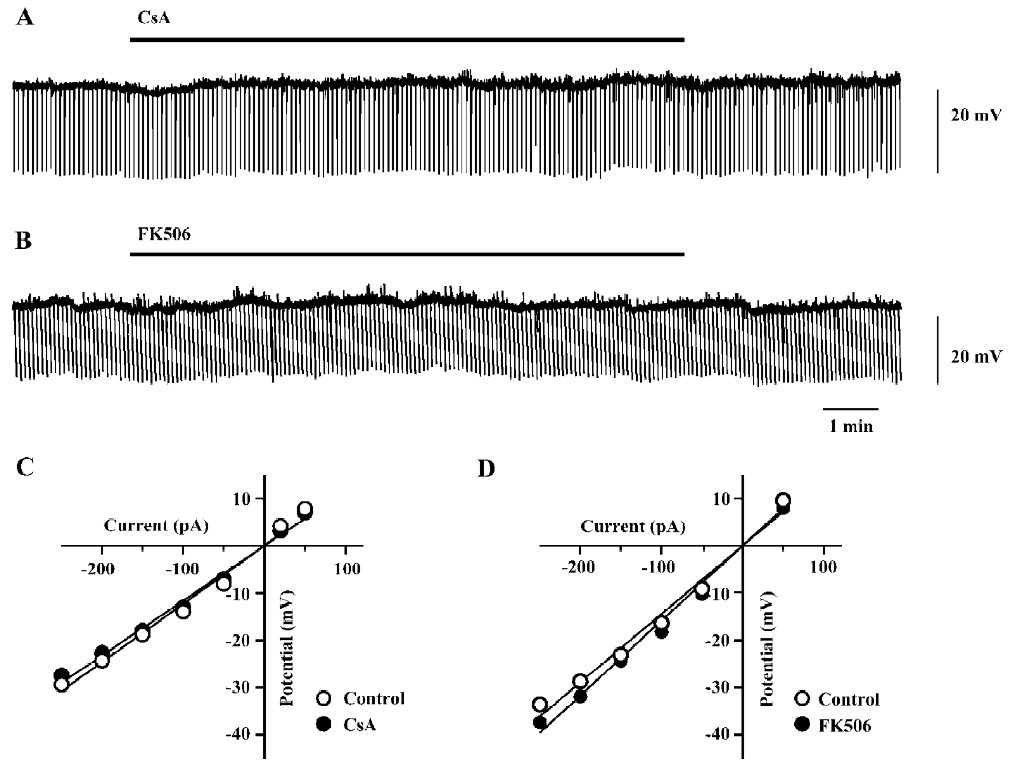
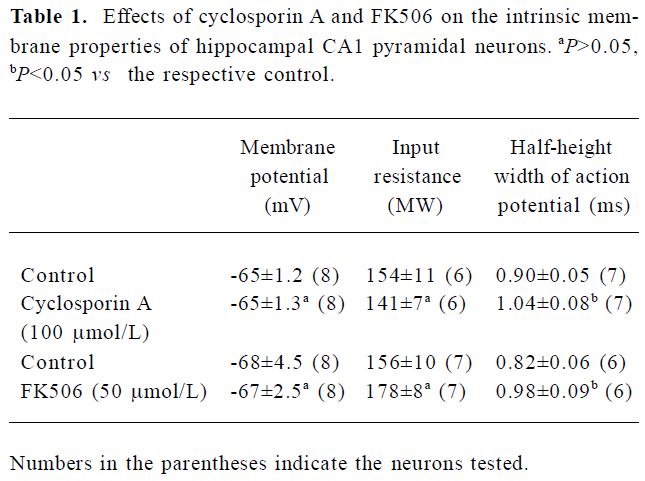
Full table
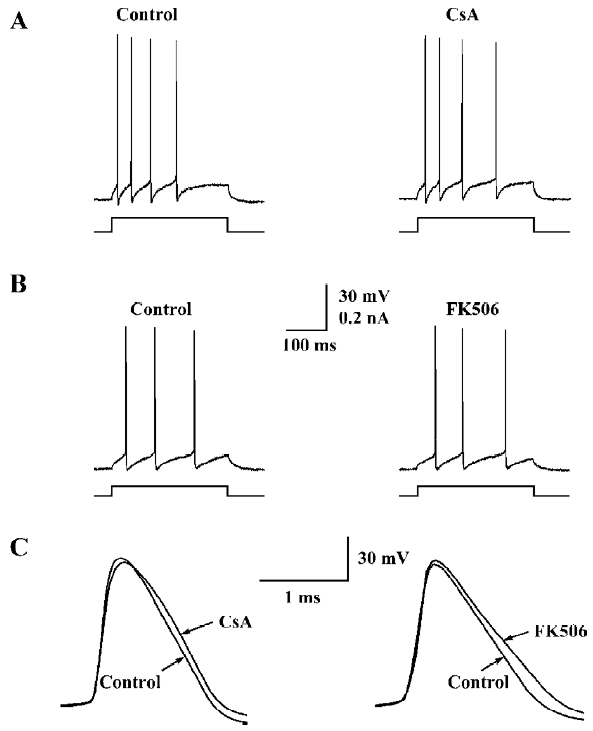
However, a closer examination revealed that both the drugs caused moderate but consistent broadening of the ac-tion potential without affecting its upstroke and amplitude (Figure 2C). As shown in Table 1, the half-height width of the action potential was increased by 18%±5% (n=7, P<0.05) during perfusion with CsA (100 µmol/L), and by 19%±3% (n=6, P<0.05) during perfusion with FK506 (50 µmol/L).
CsA selectively inhibits the IK in hippocampal neurons FK506-induced spike broadening might be ascribed to its inhibition on the IK[11]. However, the mechanism underlying CsA-induced spike broadening remains unclear. Thus, the effects of CsA on voltage-activated K+ currents were investigated in dissociated hippocampal neurons. As shown in Figure 3A, the external application of CsA (100 µmol/L) inhibited the IK, but did not affect the fast transient K+ current (IA). The inhibition of IK by CsA developed rapidly and reached a level of nearly 80% of the maximum inhibition within 20 s (Figure 3B). Moreover, the current only partially recovered upon washing out for 2 min. Comparing the I-V relationship of IK in the control and in the presence of CsA reveals that the drug does not change the threshold for the activation of the K+ current, but markedly reduces its amplitude over the entire activation range (Figure 3C). In the presence of CsA (100 µmol/L), the relative amplitudes of the IK (I/I0) elicited with depolarizing steps to 0, +20, +40, and +60 mV were 70.9%±6.1%, 76.4%±4.3%, 76.7%±2.8%, and 73.1%±3.3%, respectively (n=5, P>0.05, ANOVA), suggesting that the inhibition was voltage-independent. The threshold concentration of CsA was 10 µmol/L (Figure 3D). At 30 and 100 µmol/L, the drug inhibited the K+ current by 25.5%±1.9% and 34.6%±4.8% (n=5 for each), respectively. At concentrations above 100 µmol/L, CsA was not completely dissolved.
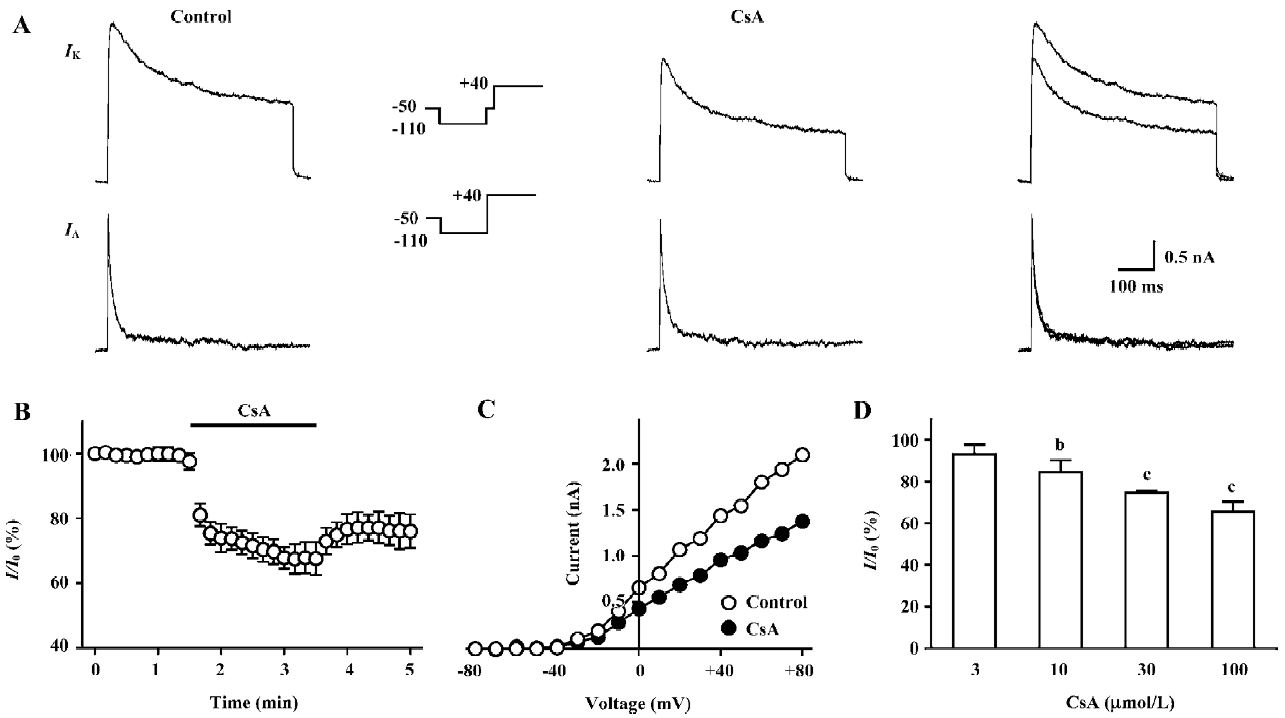
Effects of CsA on the kinetic properties of the IK in hippocampal neurons The external application of CsA did not significantly alter the voltage-dependence both of the steady-state activation and inactivation of the IK (Figure 4A, 4B). In the presence of CsA (100 µmol/L), the value of V1/2 of activation was changed from -0.4±4.3 mV to -2.5±2.9 mV (n=6, P>0.05), whereas the value of slope factor k of activation was almost identical (from 16.5±1.2 to 16.0±0.7, n=6, P>0.05). The value of V1/2 of inactivation was changed from -87.4±2.4 mV to -92.9±1.2 mV (n=5, P>0.05), whereas the value of slope factor k of inactivation changed from -11.6±0.6 to -12.7±2.0 (n=5, P>0.05). The external application of CsA (100 µmol/L) also did not alter the time course of recovery of the IK from inactivation (Figure 4C). In the control and in the presence of CsA, the time constant of recovery was 301.9±8.9 ms and 314.9±16.4 ms, respectively (n=5, P>0.05).
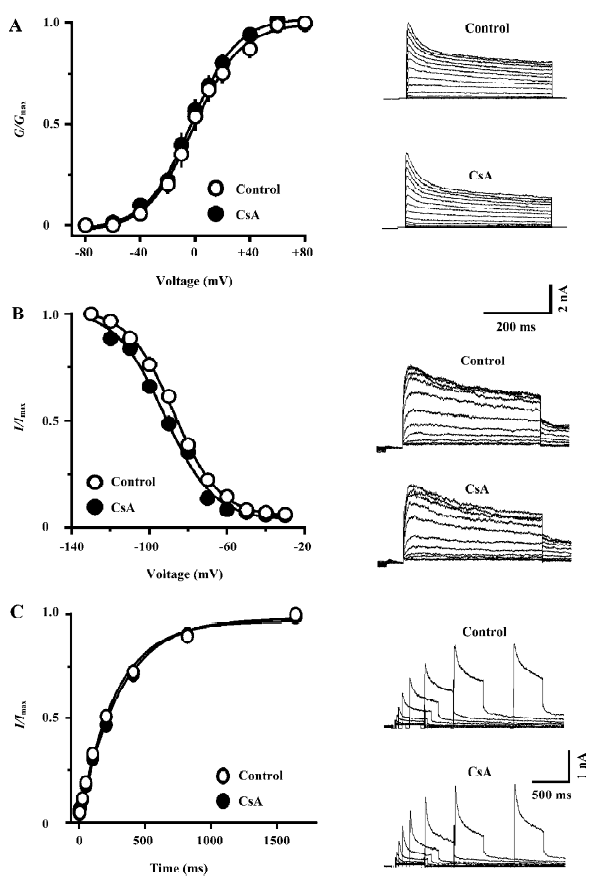
Lack of effect of intracellular dialysis of CsA on the IK in hippocampal neurons CsA is membrane permeable[3]. If externally-applied CsA had inhibited the IK through the inhibition of calcineurin within neurons, the intracellular dialysis of CsA would have caused similar inhibition. The concentration of CsA for the intracellular dialysis was 100 µmol/L, which inhibited the K+ current by approximately 35%, when applied externally (Figure 3D). After the patch membrane was ruptured, the relative amplitudes of the IK (I/I0) in the neurons dialyzed with CsA were nearly identical to the respective control values throughout the recording period of 10 min (Figure 5A). Instead, the intracellular dialysis of a blocker of the IK, tetraethylammonium (TEA; 5 mmol/L) caused progressive inhibition on the K+ current (Figure 5B). At 10 min after the patch membrane was ruptured, the value of I/I0 in the neurons dialyzed with TEA (49.5%±9.7%, n=5) was significantly different from that in the control group (88.6%±2.3%, n=6, P<0.05). The result suggests that the inhibition of the IK by CsA should not be caused by the inhibition of calcineurin.
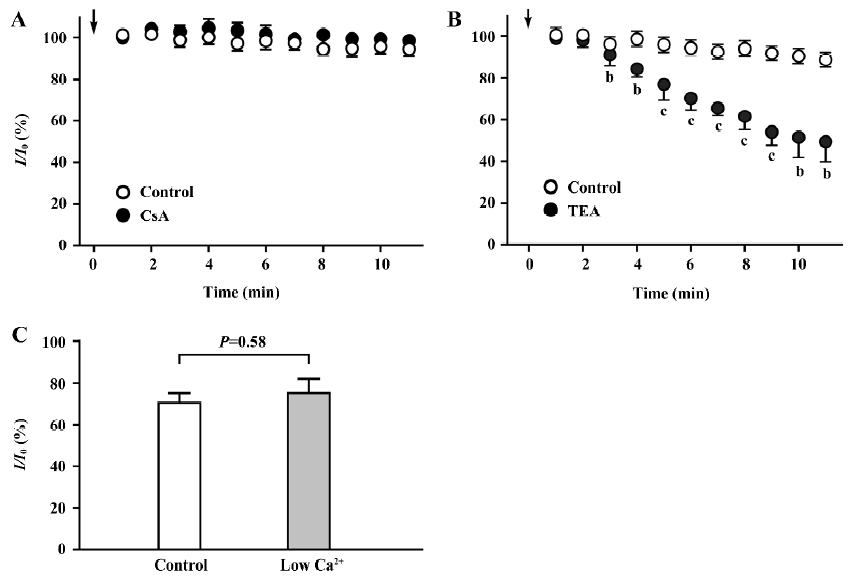
Inhibition of the IK by CsA in low Ca2+ conditions To further rule out the involvement of calcineurin in the inhibition of the IK by CsA, we examined whether the inhibition could occur under low Ca2+ conditions that block the basal activity of calcineurin. The conditions were achieved with a 0 Ca2+ pipette solution containing EGTA (10 mmol/L) and a low Ca2+ external solution that contained Ca2+ (0.25 mmol/L) and nifedipine (5 µmol/L) to minimize the influx of Ca2+ [17]. We found that the effect of CsA (100 µmol/L) on the IK examined under low Ca2+ conditions was almost identical to that examined under the control conditions (Figure 5C). The value of I/I0 under low Ca2+ conditions was 75.2%±6.9% (n=5), whereas that under control conditions was 70.3%±4.9% (n=6, P=0.58 vs low Ca2+ conditions).
Discussion
A large body of evidence has shown that CsA and FK506 exert modulatory actions on ion channels, G protein-coupled receptors, and other target proteins in the brain through the inhibition of calcineurin[2,3]. The present study demonstrates for the first time that CsA (up to 100 µmol/L) and FK506 (up to 50 µmol/L) did not significantly alter the passive electrical properties of native cortical neurons, but slowed down repolarization of the action potential. The results differ from that obtained in cultured cortical neurons, where the perfusion of CsA (20 µmol/L) caused sustained depolarizing responses with an increasing rate of spontaneous firing[14]. The discrepancy is most likely due to the different preparations used. In the present study, we further demonstrate that CsA selectively inhibits the IK in rat hippocampal neurons. Taken together with a similar result with FK506[11], it is conceivable that the spike broadening caused by the immunosuppressant drugs is due to the inhibition of the IK.
An interesting finding in this study is that in addition to acting through the inhibition of calcineurin[5,6], CsA could exert a direct inhibition on the IK in rat hippocampal neurons. Increasing evidence shows that FK506 could modulate the activities of K+ channels without the involvement of calcineurin. For instance, FK506 was found to directly prolong the mean open time of the Ca2+-activated K+ channel in cultured rat hippocampal neurons[15]. FK506 was also found to prolong the duration of the action potential of rat ventricular myocytes, which resulted from the direct inhibition on the transient outward and the delayed rectifier K+ currents[16,17]. In 2 recent studies, FK506 was demonstrated to directly inhibit the IK in rat hippocampal CA1 pyramidal neurons[11] and in the Kv1.3 channel expressed in CHO cells[18]. Thus far, however, it is unclear whether CsA may directly affect the K+ channels. In this study, we demonstrate that the inhibition of the IK by CsA occurred without the involvement of calcineurin. The intracellular dialysis of CsA was ineffective (Figure 5A), suggesting that the inhibition of the IK by CsA was not caused by the formed CsA/cyclophilin A complex, or the subsequent inhibition of calcineurin[7], but by the drug molecule itself. The inhibition persisted under the low Ca2+ conditions (Figure 5C), which led to a low nanomolar level of intracellular-free Ca2+ [12,18] and blocked the basal activity of calcineurin[17].
It should be noted that CsA is much less potent than FK506 for causing direct inhibition on the IK. In contrast to FK506, which caused marked hyperpolarizing shifts of steady-state activation and inactivation curves of the IK[11], CsA at the highest concentration tested (100 µmol/L) did not significantly alter the kinetic parameters of the IK (Figure 4), suggesting that the mechanism of the inhibition of the IK by CsA was different from that by FK506. The hyperpolarizing shift of the steady-state inactivation curve by FK506 has been proposed as the mechanism underlying its inhibition on the Kv1.3 channel expressed in CHO cells[18], and is probably responsible for the slowly developed inhibition on the IK in hippocampal neurons, which accounts for approximately 60% of the maximum inhibition[11]. In contrast, the inhibition of the IK by CsA had rapid onset, and immediately reached a level of nearly 80% of the maximum inhibition (Figure 3B), suggesting that CsA mainly acts as a blocker at the out mouth of the delayed rectifier K+ channel.
In conclusion, the present study demonstrates that CsA and FK506 do not significantly alter the passive electrical properties of rat hippocampal pyramidal neurons, but slow down the repolarizing phase of the action potential. Taken together with our previous finding with FK506, it is conceivable that the spike broadening caused by the immunosuppressant drugs is due to the direct inhibition on the IK.
References
- Price NE, Mumby MC. Brain protein serine/threonine phos-phatases. Curr Opin Neurobiol 1999;9:336-42.
- Yakel JL. Calcineurin regulation of synaptic function: from ion channels to transmitter release and gene transcription. Trends Pharmacol Sci 1997;18:124-34.
- Herzig S, Neumann J. Effects of serine/threonine protein phosphatases on ion channels in excitable membranes. Physiol Rev 2000;80:173-210.
- Mansuy IM, Shenolikar S. Protein serine/threonine phosphatases in neuronal plasticity and disorders of leaning and memory. Trends Neurosci 2006;29:679-86.
- Misonou H, Mohapatra DP, Menegola M, Trimmer JS. Calcium- and metabolic state-dependent modulation of the voltage-dependent Kv2.1 channel regulates neuronal excitability in response to ischemia. J Neurosci 2005; 25: 11 184–93.
- Misonou H, Mohapatra DP, Park EW, Leung V, Zhen D, Misouno K, et al. Regulation of ion channel localization and phosphorylation by neuronal activity. Nature Neurosci 2004;7:711-8.
- Snyder SH, Lai MM, Burnett PE. Immunophilins in the nervous system. Neuron 1998;21:283-94.
- Gao ZB, Chen XQ, Hu GY. Inhibition of excitatory synaptic transmission by trans-resveratrol in rat hippocampus. Brain Res 2006;1111:41-7.
- Magee JC. Dendritic hyperpolarization-activated currents modify the integrative properties of hippocampal CA1 pyramidal neurons. J Neurosci 1998;18:7613-24.
- Hu GY, Biro Z, Hill RH, Grillner S. Intracellular QX-314 causes depression of membrane potential oscillations in lamprey spinal neurons during fictive locomotion. J Neurophysiol 2002;87:2676-83.
- Yu Y, Chen XQ, Cui YY, Hu GY. Calcineurin-independent inhibition of the delayed rectifier K+ current by the immunosuppressant FK506 in rat hippocampal neurons. Brain Res 2007;1148:62-8.
- Numann RE, Wadman WJ, Wong RKS. Outward currents of single hippocampal cells obtained from the adult guinea-pig. J Physiol 1987;393:331-53.
- Klee R, Ficker E, Heinemann U. Comparison of voltage-dependent potassium currents in rat pyramidal neurons acutely isolated from hippocampal regions CA1 and CA3. J Neurophysiol 1995;74:1982-95.
- Victor RG, Thomas GD, Marban E, O’Rourke B. Presynaptic modulation of cortical synaptic activity by calcineurin. Proc Natl Acad Sci USA 1995;92:6269-73.
- Terashima A, Nakai M, Hashimoto T, Kawamata T, Taniguchi T, Yasuda M, et al. Single-channel activity of the Ca2+ -dependent K+ channel is modulated by FK506 and rapamycin. Brain Res 1998;786:255-8.
- duBell WH, Wright PA, Lederer WJ, Rogers TB. Effects of the immunosuppressant FK506 on excitation-contraction coupling and outward K+ currents in rat ventricular myocytes. J Physiol 1997;501:509-16.
- duBell WH, Gaa ST, Lederer WJ, Rogers TB. Independent inhibition of calcineurin and K currents by the immunosuppressant FK506 in rat ventricle. Am J Physiol 1998; 275 (Heart Circ Physiol 44): H2041–52.
- Ahn HS, Kim SE, Choi BH, Choi JS, Kim MJ, Rhie DJ, et al. Calcineurin-independent inhibition of Kv1.3 by FK-506 (Tacrolimus): a novel pharmacological property. Am J Physiol Cell Physiol 2007;292:C1714-22.