Underlying mechanism of ASIC1a involved in acidosis-induced cytotoxicity in rat C6 glioma cells1
Introduction
Acid-sensing ionic channels (ASIC) are H+-gated cation channels, which belong to the degenerin/epithelial sodium channel superfamily. They are activated quickly as the extracellular pH falls. Until now, 6 ASIC subunits have been cloned: ASIC1a[1], ASIC1b[2], ASIC2a[3], ASIC2b[4], ASIC3[1,2,5], and ASIC4[6]. Functional ASIC are usually tetrameric assemblies of these ASIC subunits in homomeric or heteromeric conformation[7].
Different ASIC subunits have different kinetics and biological characteristics. Both the ASIC1a and ASIC2a subunits have been shown to be abundant in the brain, whereas ASIC2b is present in both brain and sensory neurons[8,9]. In the rat hippocampal neurons, our studies showed that these 3 subunits could form the function of ASIC [10]. ASIC4 was found to coexpress with other subunits in many areas of the brain. ASIC3 is expressed almost exclusively in the sensory neurons in rats[11]. Different from other subtypes, homomeric ASIC1a can flux Ca2+ besides Na+ permeability [12].
Until now, the functions of ASIC were still unclear, although they were related in acidosis-induced injury. In sensory neurons, ASIC were shown to play an important role in nociception during tissue acidosis, for instance, in muscle and cardiac ischemia[13] and in inflammation[14]. In peripheral sensory neurons, ASIC were implicated in mechanosensation[15] and the perception of pain during tissue acidosis, particularly in the ischemic myocardium where ASIC are likely transducer of anginal pain[16–19]. The presence of ASIC in the brain, which lacks nociceptors, suggests that these channels have functions beyond nociception. Now, it has shown that ASIC1a is involved in synaptic plasticity, learning/memory, and fear conditioning[20,21] and participates in neuronal damage associated with tissue acidosis[22].
ASIC have been found in malignant gliomas[23]. Recent studies have revealed that the surface expression of ASIC2 could inhibit the amiloride-sensitive current and migration of glioma cells[24]. In our former studies[25], we also found that extracellular acid induced cell death and apoptosis in rat C6 glioma cells via the ASIC1a mechanism, and reducing ASIC1a proteins by the stable expression of short hairpin RNA inhibited apoptosis and increased cell viability following acid exposure. These studies indicated that ASIC might have some important functions in the gliomas within acidosis. However, the underlying mechanism of ASIC1a involved in acidosis-induced cytotoxicity in rat C6 glioma cells is still unknown. Due to the function of intracellular calcium ([Ca2+]i) and the high Ca2+ permeability of ASIC1a, we aimed to explore the relationship between Ca2+ and ASIC1a during acidosis combined with the newly-developed RNA interference (RNAi) technique and the calcium imaging experiment in the present study.
Materials and methods
Materials Fluo-3 acetoxymethyl ester (Fluo-3-AM) was purchased from Molecular Probes (Eugene, OR, USA). Anti-ASIC1a was purchased from Cell Signaling Technology (Beverly, MA, USA). G418, penicillin, L-glutamine, nimodi-pine, MK801 (dizocilpine maleate), 6-cyano-7-nitroquino-xaline-2,3-dione (CNQX), and all other reagents were purchased from Sigma (St Louis, MO, USA) unless otherwise indicated.
Cell culture The rat glioma C6 cell line was derived from rat neurogliocytoma. The wild-type C6 cells were cultured in RPMI-1640 medium (Invitrogen, Carlsbad, CA, USA) supplemented with 10% fetal bovine serum (Invitrogen, USA), 100 units/mL penicillin, 100 µg/mL streptomycin, L-glutamine (0.03%, w/v), and sodium bicarbonate (2.2%, w/v). The ASIC1a-silenced cells and the negative control cells were cultured with G418 (400 mg/L). The cells were cultured at 37 °C and 5% CO2 in a humidified incubator (310/Thermo, Forma Scientific, Marietta, OH, USA).
Building the stable ASIC1a-silenced cell line To observe the role of [Ca2+]i in the acidosis and its relationship with ASIC1a, we built the ASIC1a-silenced C6 cell line. We designed and prepared the shRNA sequences directly against ASIC1a, and the control sequence was designed according to Dharmacon siDESIGN Center software (Dharmacon, Lafayette, CO, USA). The sequence for ASIC1a RNAi was: 5'-GAT CCC GCGTGAATTCTACGACAGATTCAAGAGA-TCTGTCGTAGAATTCACGCTTTTT-3' (forward) and 5'-AGCTAAAAAGCGTGAATTCTACGACAGATCTCTTGAATCT-GTCGTAGAATTCACGCGG-3' (reverse); the sequence for the control RNAi was: 5'-GATCCCTTCTCCGAACGTGTCA-CGTTTCAAGAGAACGTGACACGTTCGGAGAATTTTT-3' (forward) and 5'-AGCTAAAAATTCTCCGAACGTGTCA-CGTTCTCTTGAAACGTGACACGTTCGGAGAAGG-3' (reverse). The shRNA were synthesized in desalted and purified form by Dharmacon (USA). Then, we constructed the plasmid vectors with the pGCsi-U6-Neo-GFP (Green Fluorescent Protein) cloning vector. These C6 cells cotransfected pGCsi-U6-Neo-GFP with either an ASIC1a-specific RNAi or a control RNAi expression vector. Stable clones were selected with G418 and were screened for ASIC1a protein knockdown. Then we chose 1 clone from the ASIC1a-specific RNAi (C6-sh1a) and the control RNAi clones (C6-neg).
RT-PCR Total RNA was purified using RNeasy columns (Qiagen, Valencia, CA, USA). RT-PCR was performed in 50 µL reactions using 100 ng of RNA, 0.5 µmol/L of each primer, and an annealing temperature of 53 °C for 25 cycles. All other PCR conditions and reagents were supplied and recommended by the manufacturer’s protocol for the Titan one-step system (Roche Applied Science, Indianapolis, IN, USA). The primer sequences for ASIC1a were: 5'-CGGATCCATGG-AATTGAAGACCGAGGA-3' (forward) and 5'-CGATATCTG-CAGGTAAAGTCCTCAAACG-3' (reverse); for the GAPDH, the primers were: 5'-GTC AAC GGA TTT GGT CGT ATT G-3' (forward) and 5'-AGT GAT GGCATG GAC TGT GGT-3' (reverse).
Western blot analysis The Western blot analyses of ASIC1a were performed using specific antibodies (ADI, San Antonio, TX, USA)[1]. A cell lysate containing 30 µg protein was fractionated by SDS-PAGE, and then the proteins were transferred to a nitrocellulose membrane. The membrane was first rinsed with TBST [20 mmol/L Tris-HCl (pH 7.4), 0.15 mol/L NaCl, and 0.05% Tween 20] and then blocked with 5% (w/v) skim milk in TBST for 1 h at room temperature. The blocked membrane was subsequently probed for 1 h at room temperature with 1:200–1:1000 dilutions of first antibodies in blocking buffer. After the membrane had been washed 3 times with TBST, it was incubated for 1 h at room temperature either with horseradish peroxidase-conjugated antibodies against rabbit immunoglobulin G. After the membrane had been washed with TBST, bands of protein on the membrane were visualized using the Enhanced chemiluminescence detection system (PerkinElmer Life Sciences, Boston, MA, USA).
Measurement of [Ca2+]i calcium The cells were plated into a polylysine-coated coverslip 1 d before each experiment. After washing 3 times with D-Hanks’ balanced salt buffer, the cells were incubated with 4 µmol/L Fluo-3-AM (Molecular Probes, USA) for 30 min at 37 °C. The cells were then washed twice with fresh D-Hanks’ buffer and immediately used for the experiments. The fluorescence of intracellular Fluo-3 was quantitated by confocal laser scanning fluorescence microscopy (Leica TCS4D, Leica Lasertechnik, Heidelberg, Germany) using excitation and emission wavelengths of 488 and 525, respectively. Gray scale images with 0–255 steps were collected at different time points before and up to 10 min and archived as Tiff image files for later analysis. In order to eliminate the effect of the glutamate receptors and voltage-gated Ca2+ channels, MK801 (10 µmol/L), CNQX (20 µmol/L), and nimodipine (5 µmol/L) were also added in the extracellular solutions. Each time, 7–9 cells were measured, and the mean value of the fluorescence of each cell was calculated. After recording the basic fluorescence intensity for approximately 150 s, the acidic solution (hydrochloric acid), 20 µL of which had been adjusted and could make the pH value of the extracellular solution (pH 7.4, 1.5 mL) decrease to pH 6.0, was directly added with a pipettor far from these recording cells. The recording continued while adding acidic solution and lasted for approximately 600 s. The intensity of the fluorescence in individual cells was measured using Leica quantitation software (Germany).
Statistical analysis Data were described using mean± SEM. Mean values were compared using either Student’s t-test or ANOVA. Two-way ANOVA was used to separate the effects of 2 variables and determine their interaction. P<0.05 was considered statistically significant.
Results
Confirming the ASIC1a-silenced C6 cell line In the rat C6 glioma cells, the mRNA expression of ASIC1a, ASIC1b, ASIC2a, and ASIC2b was identified with the RT-PCR experiment and we did not find the expression of ASIC3 and ASIC4[25]. To observe the role of [Ca2+]i in the acidosis and its relationship with ASIC1a, we used the ASIC1a-silenced C6 cell line. The method to build the stable cell line was described in Materials and methods.
The result of the RT-PCR (Figure 1A) and Western blotting (Figure 1B) showed that a dramatic decrease in ASIC1a mRNA level and protein expression occurred in the ASIC1a-silenced cells (C6-sh1a) compared with the wild-type C6 cells (C6-neo) and the control RNAi cells (C6-neg). It was confirmed that the ASIC1a-knockdown C6 glioma cell line was built.
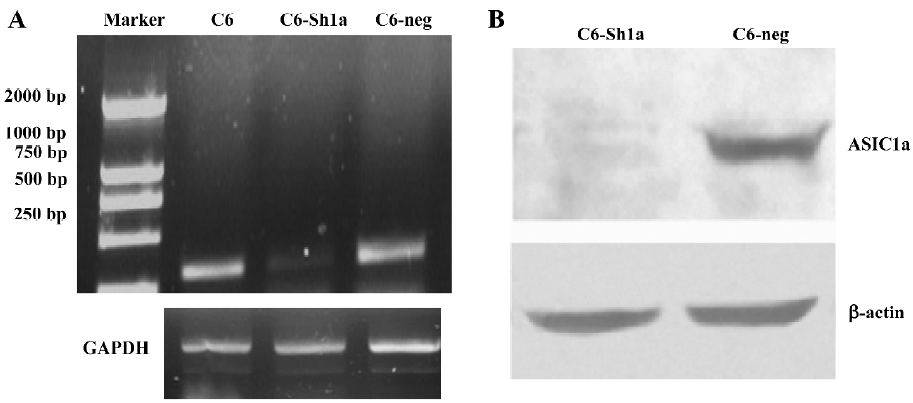
Application of acid increased the [Ca2+]i transitorily in the wild-type C6 cells, but not in the ASIC1a-silenced cells It is well-known that [Ca2+]i plays a critical role in the regulation of cell death via either the apoptotic or necrotic pathways[26–30]. ASIC1 has been proposed to play a role in the pathogenesis of diseases associated with extracellular acidosis, including seizures and cerebral ischemia[20–22]. Here, we tested the changes of [Ca2+]i among the 3 types of cells applied with extracellular acid. From the confocal micrographs (Figure 2A) and the representative traces (Figure 2B), the application of extracellular acid (pH 6.0) significantly increased the [Ca2+]i in the wild-type C6 cell line (Figure 2A,2B), while the changes of [Ca2+]i in the C6-sh1a cells were much lower than those in 2 other types of cell lines (Figure 2C, 2D). After the acid application, [Ca2+]i recovered near the basal value. Thus, the results showed that ASIC1a mediated the increase of [Ca2+]i induced by extracellular acid, which also indicates that cytotoxicity induced by acidosis might be related with [Ca2+]i .
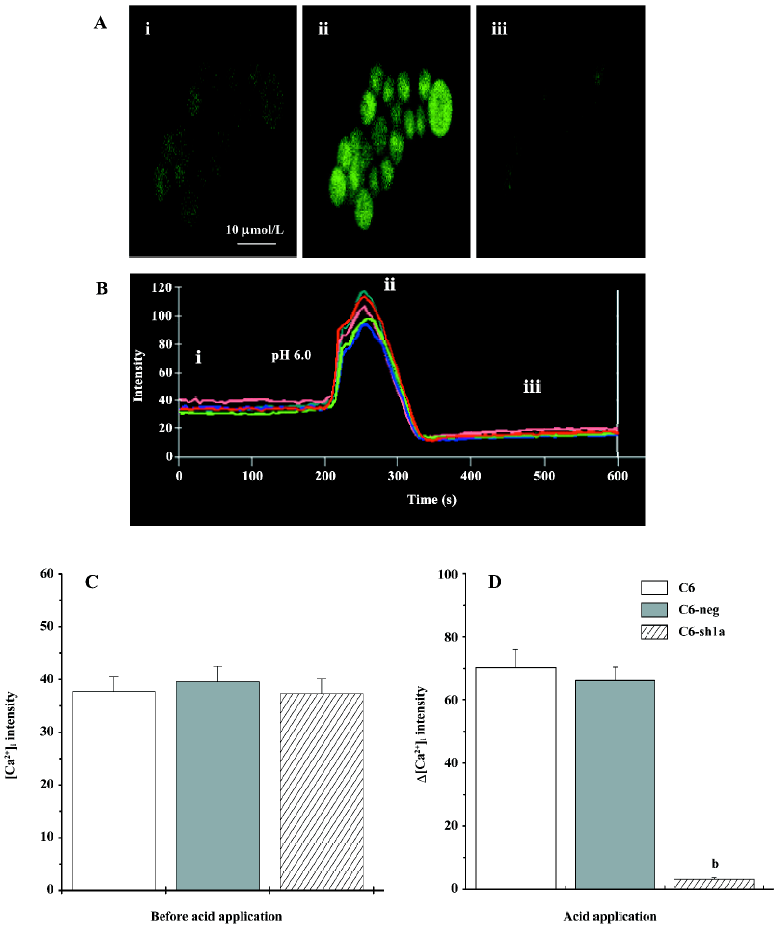
Knockdown of ASIC1a decreased [Ca2+]i during pronged acid exposure Although the results suggest that ASIC1a mediated the increase of [Ca2+]i induced by extracellular acid, it might be only part of the reason for cytotoxicity. After the acid application, the detectable increase in [Ca2+]i occurred and lasted for only several minutes (Figure 2B). During prolonged acid application, ASIC1a currents desensitized, and [Ca2+]i returned to basal values (Figure 2A, 2B). If the acid stimulus (pH 6.0) to cells was limited in a few minutes, the cytotoxicity among the 3 types of cells would not be severe and distinct (data not shown). So we measured the [Ca2+]i before and after the 3 h acid stimulus when apoptosis among the 3 types of cell lines was distinct. Using the Fluo-3 fluorescent Ca2+-imaging technique, we found that [Ca2+]i in the wild-type C6 cells and C6-neg cells had increased compared to the C6-sh1a cells (Figure 3). This result was consistent with acid-induced cytotoxicity, although the detailed mechanism is still unclear. The result suggests that prolonged acid exposure induces the changes of [Ca2+]i via ASIC1a mechanisms.
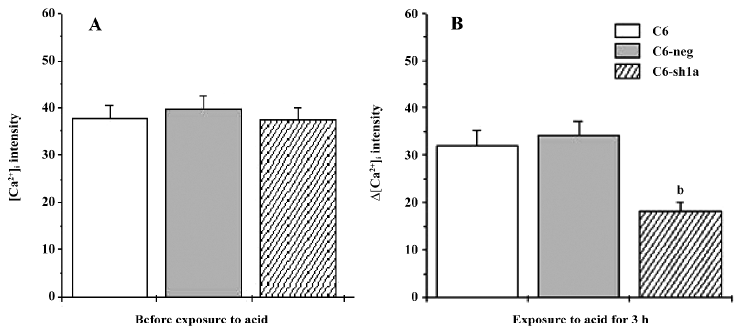
Discussion
The ASIC were activated by H+ and inactivated rapidly after activation. This transient current carried Na+ displays a Na+/K+ selectivity ratio of 10. At the same time, ASIC showed some permeability to Ca2+. The relative Ca2+ permeability was highest in ASIC1a, for which PNa/PCa permeability ratios of 2.5–17 have been determined[1]. The PNa/PCa ratio for ASIC3 and ASIC1b was ~100. Thus, we presumed that the Ca2+ permeability of ASIC1a might be important for its cellular function. From our studies, the [Ca2+]i in the cells expressing ASIC1a was higher than that in the ASIC1a-silenced cells, which indicated that ASIC1a activity enhanced acidosis-induced injury, not only because the higher [Ca2+]i contrast to the ASIC1a-silenced cells could evoke the mechanism of apoptosis and then lead to cell death of some cells, but also because the overloading of [Ca2+]i in some cells activated a cascade of cytotoxic events and directly induced cell death[26–29].
In our previous study, we observed that acidosis-induced cytotoxicity could be obviously inhibited by targeting the ASIC1a mRNA with short hairpin RNA[25]. In the present study, we further studied the underlying mechanism involved in this process. It was found that the activation of ASIC1a could induce glutamate-independent and VGCC (voltage-gated calcium channels)-independent Ca2+ entry in the wild-type C6 cells, but not in the stably ASIC1a-silenced C6 cell line. Ca2+ performed a very important role in cytotoxicity. Evidence has revealed that cytotoxicity could be directly induced by the increase of [Ca2+]i[24–28]. When Ca2+ has a 20% increase in the total calcium content, cell death can take place[28]. According to the cytotoxicity of [Ca2+]i, it indicated that the increase of [Ca2+]i was most likely to be respon-sible for acidosis-induced cytotoxicity. Thus, these results provided evidence that the change of [Ca2+]i during extracellular acidosis might be responsible for the cytotoxicity via the ASIC1a mechanism. The results indicated that [Ca2+]i was one of the mechanism for cytotoxicity via the ASIC1a pathway.
ASIC1a is a quick transient channel that can only induce short depolarization or calcium entry in the cell. Even if the acidic stimulus lasts, ASIC1a becomes inactive. The reason why [Ca2+]i was lower in the ASIC1a silenced cells than in the longer exposure to acid remains unclear, and further research was still needed to explain the mechanism in detail.
We utilized the highly specific technology of RNAi to directly demonstrate that extracellular acid induces cytotoxicity by activating ASIC1a with the resultant toxicity of [Ca2+]i . Using ASIC1a-deficient mice is limited and difficult, thus, the use of ASIC1a shRNA will greatly facilitate the precise identification of the ASIC1a gene function and its mechanism. RNAi mediated by shRNA is a powerful technology that allows the silencing of genes with great specificity and potency. Through our studies, we are sure that RNAi will be more widely used in future pharmacological research.
Footnote
Project supported by a grant from the National Natural Science Foundation of China (N
References
- Waldmann R, Champigny G, Bassilana F, Heurteaux C, Lazdunski M. A proton-gated cation channel involved in acid-sensing. Nature 1997;386:173-7.
- Waldmann R, Bassilana F, de Weille J, Champigny G, Heurteaux C, Lazdunski M. Molecular cloning of a non-inactivating proton-gated Na+ channel specific for sensory neurons. J Biol Chem 1997;272:20975-8.
- Waldmann R, Lazdunski M. H. +-gated cation channels: neuronal acid sensors in the NaC/DEG family of ion channels. Curr Opin Neurobiol 1998;8:18-24.
- Lingueglia E, de Weille JR, Bassilana F, Heurteaux C, Sakai H, Waldmann R, . A modulatory subunit of acid sensing ion channels in brain and dorsal root ganglion cells. J Biol Chem 1997; 272: 29 778–83.
- Babinski K, Le KT, Seguela P. Molecular cloning and regional distribution of a human proton receptor subunit with biphasic functional properties. J Neurochem 1999;72:51-7.
- Akopian AN, Chen CC, Ding Y, Cesare P, Wood JN. A new member of the acid-sensing ion channel family. Neuroreport 2000;11:2217-22.
- Waldmann R, Champigny G, Lingueglia E, De Weille JR, Heurteaux C, Lazdunski M. H. +-gated cation channels. Ann N Y Acad Sci 1999;868:67-76.
- Price MP, Snyder PM, Welsh MJ. Cloning and expression of a novel human brain Na+ channel. J Biol Chem 1996;271:7879-82.
- Bassilana F, Champigny G, Waldmann R, de Weille JR, Heurteaux C, Lazdunski M. The acid-sensitive ionic channel subunit ASIC and the mammalian degenerin MDEG form a heteromultimeric H-gated Na channel with novel properties. J Biol Chem 1997; 272: 28 819–22.
- Weng XC, Zheng JQ, Gai XD, Li J, Xiao WB. Two types of acid sensing ion channels expressed in rat hippocampal neurons. Neurosci Res 2004;50:493-9.
- Olson TH, Riedl MS, Vulchanova L, Ortiz-Gonzalez XR, Elde R. An acid sensing ion channel (ASIC) localizes to small primary afferent neurons in rats. Neuroreport 1998;9:1109-13.
- Yermolaieva O, Leonard AS, Schnizler MK, Abboud FM, Welsh MJ. Extracellular acidosis increases neuronal cell calcium by activating acid-sensing ion channel 1a. Proc Natl Acad Sci USA 2004;101:6752-7.
- Reeh PW, Steen KH. Tissue acidosis in nociception and pain. Prog Brain Res 1996;113:143-51.
- Price MP, Lewin GR, McIlwrath SL, Cheng C, Xie J, Heppenstall PA, et al. The mammalian sodium channel BNC1 is required for normal touch sensation. Nature 2000;407:1007-11.
- Bevan S, Yeats J. Protons activate a cation conductance in a sub-population of rat dorsal root ganglion neurons. J Physiol 1991;433:145-61.
- Ugawa S, Ueda T, Ishida Y, Nishigaki M, Shibata Y, Shimada S. Amiloride-blockable acid-sensing ion channels are leading acid sensors expressed in human nociceptors. J Clin Invest 2002;110:1185-90.
- Sluka KA, Price MP, Breese NM, Stucky CL, Wemmie JA, Welsh MJ. Chronic hyperalgesia induced by repeated acid injections in muscle is abolished by the loss of ASIC3 but not ASIC1. Pain 2003;106:229-39.
- Chen CC, Zimmer A, Sun WH, Hall J, Brownstein MJ, Zimmer A. A role for ASIC3 in the modulation of high-intensity pain stimuli. Proc Natl Acad Sci USA 2002;99:8992-7.
- Benson CJ, Eckert SP, McCleskey EW. A possible mediator of myocardial ischemic sensation. Circ Res 1999;84:921-8.
- Wemmie JA, Askwith CC, Lamani E, Cassell MD, Freeman JHJ, Welsh MJ. Acid-sensing ion channel 1 is localized in brain regions with high synaptic density and contributes to fear conditioning. J Neurosci 2003;23:5496-502.
- Wemmie JA, Chen J, Askwith CC, Hruska-Hageman AM, Price MP, Nolan BC. The acid-activated ion channel ASIC contributes to synaptic plasticity, leaning, and memory. Neuron 2002;34:463-77.
- Xiong ZG, Zhu XM, Chu XP, Minami M, Hey J, Wei WL, et al. Neuroprotection in ischemia: blocking calcium-permeable acid-sensing ion channels. Cell 2004;118:687-98.
- Berdiev BK, Xia J, McLean LA, Markert JM, Gillespie GY, Mapstone TB, . Acid-sensing ion channels in malignant gliomas. J Biol Chem 2003; 278: 15 023–34.
- Vila-Carriles WH, Kovacs GG, Jovov B, Zhou ZH, Pahwa AK, Colby G, . Surface expression of ASIC2 inhibits the amiloride-sensitive current and migration of glioma cells. J Biol Chem 2006; 281: 19 220–32.
- Weng XC, Zheng JQ, Jin QE, Ma XY. Involvement of ASIC1a in apoptosis and cell death induced by extracellular acidosis. Acta Pharmacol Sin 2006;1 Suppl 1:186.
- Choi DWW. Glutamate neurotoxicity in cortical cell culture is calcium dependent. Neurosci Lett 1985;58:293-7.
- Mattson MPP, Chan SL. Calcium orchestrates apoptosis. Nat Cell Biol 2003;5:1041-3.
- Leist M, Nicotera P. Calcium and neuronal death. Rev Physiol Biochem Pharmacol 1998;132:79-125.
- Sattler R, Sattler M, Tymianski Y. Molecular mechanisms of calcium-dependent excitotoxicity. J Mol Med 2000;78:3-13.
- Kristián T, Siesjö BK. Calcium in ischemic cell death. Stroke 1998;29:705-18.