Expression of nicotinic acetylcholine receptor subunit α9 in type II vestibular hair cells of rats1
Introduction
The peripheral vestibular system consists of 2 sack-like structures: the sacculus and utricle, and 3 tubular structures known as the semicircular canals. Vestibular hair cells (VHC) were characterized as type I or type II based on their characteristic innervation pattern[1]. Type I vestibular hair cells (VHC I), which are shaped like bottles or flasks with rounded bases and short necks, are present in reptiles, birds and mammals, but not in fish or amphibians. VHC I is innervated by an afferent nerve calyx engulfing the entire cell body. Type II hair cells (VHC II) have cylindrical shapes and are innervated by both afferent and efferent nerve branches, mostly synapsing with the basal part of the cell[2,3]. The vestibular endorgans detect both angular velocity and linear acceleration, including gravity; the information from this system is conveyed to the central nervous system via afferent nerve fibers. Meanwhile, the efferent terminals arising in the brainstem form direct synaptic contacts with VHC II and form postsynaptic connections with the afferent calyx surrounding the VHC I.
There is a general consensus that acetylcholine (ACh) is the major transmitter released at efferent synapses in the peripheral vestibular system[4–6]. By using histochemical techniques, acetylcholinesterase (AChE), the ACh inactivating enzyme, was localized to the vestibular efferent neurons and endings[7–10]. The choline acetyltransferase (ChAT) like immunoreactivity was also traced to the efferent endings in vestibular endorgans[11–13]. ChAT and AChE activity were found in efferent terminals contacting the basal area of VHC II and the afferent chalices surrounding VHC I. Cholinergic receptors belong to 2 different classes: muscarine cholinergic receptors and nicotinic cholinergic receptors (nAChR). By using various nucleic acid and ligand-binding methods, nAChR were also localized in vestibular endorgans[14–19].
A total of 17 nAChR subunits (α1–α10, β1–β4, γ, δ, and ε) have been identified in vertebrate species which can co-assemble to generate a wide variety of nAChR[20]. The α9 and α10 subunits are localized predominantly in neuroepithelial auditory and VHC[21–25], although their presence has also been found in other tissues[26]. Recent studies have suggested the localization of mRNA in VHC of mammals. Hiel et al localized α9 AChR mRNA in VHC I and VHC II of rats using in situ hybridization with 35S-labeled ribo-probes[16]. Using immunohistochemistry techniques, Luebke et al reported that in chinchillas, rats and guinea pigs, immunoreactivity to the α9 AChR was confined to calyces around VHC I and the synaptic pole of VHC II[19]. The single cell reverse transcription polymerase chain reaction (RT-PCR) technique has higher sensitivity than the in situ hybridization technique and immunohistochemistry techniques, particularly in studies aimed to localize the cell specific expression of receptors in an individual cell[27]. With the single cell RT-PCR technique, the cell specific expression of α9 AChR in auditory hair cells has been reported[27]. With the single cell RT-PCR technique, Lustig et al demonstrated the expression of α9 AChR mRNA in both the VHC I and VHC II of chicks[18]. However, expression of α9 AChR has not been detected in the VHC of mammals by using the single cell RT-PCR technique. It was reported that the innervation pattern of the ChAT-like immunostained efferents in humans is similar to that of other mammals, including rats[13]; there were many differences between the efferent vestibular system of birds and that of mammals[6]. Further investigations on the ACh-ergic innervation pattern of the peripheral vestibular system of rats will help us to understand the efferent innervation pattern of the human vestibule.
In the present study, in order to explore the cell specific expression of α9 AChR in the VHC II of rats, we used the RT-PCR technique with gene-specific primers to detect the expression of α9 AChR in the vestibular endorgans of rats, then used single cell RT-PCR with the same primers to detect the expression of α9 AChR in single rat VHC II.
Materials and methods
RT-PCR An adult Sprague-Dawley rat (200–250 g) was anesthetized and decapitated in 1 run of the experiment. The temporal bones were quickly removed and placed in ribonuclease (RNase)-free phosphate-buffered saline (PBS) at 4 °C and pH 7.4, mimicking extracellular conditions containing (in mmol/L) NaCl (129.4), KCl (5), MgSO4 (1.6), Na2HPO4 (0.3), CaCl2 (1.2), KH2PO4 (0.4) and D-Glucose (16.7). The osmolarity of the PBS was adjusted to 295 mOsm/L by NaCl. The experiments were repeated 5 times. A total of 5 rats were used in the experiments. The vestibular organs, including the sacculus, utricle and superior and horizontal ampulla, were dissected under Stereo Microscope (JSZ, Jiangnan Novel Optics Co Ltd, Nanjing, China) and immediately homogenized in 1 mL Trizol reagent (Life Technologies Inc., Gaithersburg, MD, USA). The total RNA of the vestibular endorgans was extracted from the tissue according to the manufacturer’s instruction. The total RNA was dissolved in 20 µL RNase-free water. The PBS was used as negative control at each run of the experiment.
Gene specific primers for α9 AChR were used as outlined by Glowatzki[28]. The predicted fragment length for α9 AChR was 576 bp. The primers were designed on different exons; the sense primer (nucleotide 664–689): 5'-CTCCTCATCCC-TTGCGTCCTCATAT-3' and the anti-sense primer (nucleotide 1239–1210): 5'-GAGGCACTTGGCAATGTATTCGATA-TTTT-3'. The gene specific primers for the β-actin protein based on the rat β-actin mRNA sequence (NM_031144) were used as internal control. The predicted fragment length for β-actin cDNA was 213 bp; the sense primer (nucleotide 303–322 bp): 5'-CATTGTAACCAACT-GGGACG-3' and the anti-sense primer (nucleotide 497–515 bp): 5'-GAGGCATACA-GGGACAACA-3'. The primers were designed with the assistance of Primer Premier 5.0 Software (Premier Biosoft International, Palo Alto, CA, USA) to amplify a fragment of the sequence, including an intron.
RT and PCR were performed in the same tube according to the manufacturer’s instructions (SuperScript™ III, One-Step RT-PCR System with Platinum® Taq DNA Polymerase, Invitrogene, Life Technologies, Gaithersburg, MD, USA) in a T-gradient thermal cycle (Biometra, Whatman Biometra, Goettingen, Germany). A 20 µL RT-PCR reaction system containing: 2×RT buffer (10 µL), 1 µL (10 pmol) gene specific primers, 1 µL (20 U) RNase Inhibitor (Promega, Madison, WI, USA), 6 µL RNase-free water, 1 µL (1 U) RT® Platinum Taq and a 1 µL total of RNA. The RT step was performed at 45 °C for 60 min. The PCR had 40 cycles; the amplification conditions for α9 AChR were as follows: 95 °C, 20 s; 58 °C, 30 s; 72 °C, 40 s. The amplification conditions for β-actin were as follows: 95 °C, 20 s; 54 °C, 30 s; 72 °C, 30 s. After cycling, the reaction was extended at 72 °C for 10 min. The products of the reaction were stored at 4 °C. Five microliters from the 20 µL products were run on a 1.5% agarose gel containing ethidium bromide. Then remnant PCR products were given to the sequencing company (BGI LifeTech Co Ltd, Beijing, China) for direct sequencing using an ABI377 sequencer (PE Applied Biosystems, Foster City, CA, USA).
Cell preparation One rat was used for 1 run of the experiment. A total of 5 runs of experiments were done as part of this study. The tissue of rat vestibular endorgans was dissected and immediately placed into calcium-free PBS supplemented with collagenase IA (0.25 mg/mL) (Sigma, St Louis, MO, USA). After the endorgans were incubated in the PBS solution for 5 min at 4 °C, PBS without collagenase I A was used in exchange to the solution 3 times. Subsequently, single hair cells were mechanically dissociated from the endorgans by cutting through the epithelium with a sharpened needle under Stereo Microscope (JSZ, Jiangnan Novel Optics Co, Ltd, Nanjing, China) at 4 °C. The single hair cells were prepared for single cell RT-PCR.
Single cell harvest Single hair cells were moved to an inverted microscope (IX71, Olympus Corp, Tokyo, Japan). At 200× total magnification, an individual VHC II was identified according to criteria outlined by Ricci et al[29]. A glass pipette with a tip diameter of 50 µm was attached to the micromanipulator (Narashige, Tokyo, Japan). The pipette tip was advanced with the micromanipulator until contact with a VHC II, then gentle suction was used to aspirate the cell into the pipette. Subsequently, the pipette with the cell was immerged into a fresh PBS solution at room temperature. The pipette went down with the micromanipulator arm until its tip nearly made contact with the bottom of the glassy utensil and the VHC II were expelled by gentle positive pressure. Finally, another glass pipette with a tip diameter of 10–20 µm, filled with about 2 µL RNase-free water and 0.2 µL (2 U) RNase Inhibitor was used to aspirate the VHC II into the pipette again. The contents of the pipette were then immediately expelled into a 200 µL microfuge PCR tube containing 4 µL RNase-free water with 0.4 µL (4 U) RNase Inhibitor using positive pressure; the tip, which was about 2 mm long, was broken off. Samples of surrounding fluid and cell debris were also aspirated and used as negative controls. The PCR tube was used in the following reaction or stored at -75 °C.
Single cell RT-PCR RT and PCR were performed in the same tube according to the manufacturer’s instructions (SuperScript™ III One-Step RT-PCR System with Platinum® Taq DNA Polymerase, Life Technology, Gaithersburg, MD, USA) in a thermal cycler (Biometro, Germany). A 20 µL RT-PCR reaction system containing: 2×RT buffer (10 µL), 1 µL (10 pmol) gene specific primers, 1 µL (20 U) RNase Inhibitor, 6 µL RNase-free water, 1 µL (1 U) RT® Platinum Taq and PBS with single VHC II or PBS with cell debris or surrounding fluid (1 µL). These components were added and mixed by flicking the tube, followed by brief centrifugation. The RT-PCR reaction conditions were the same as earlier described. After the first round of RT-PCR, the second round of PCR was carried out. In the second round of PCR, 1 µL of the products yielded in the first round of PCR was used as template, using the same primers. A 50 µL PCR reaction system containing: 10×Mg2+-free PCR buffer (5 µL), 3 µL(25 mmol/L) MgCl2, 4 µL (2.5 mmol/L) dNTP, 1 µL (10 pmol) gene specific primers, 35 µL RNase-free water, 1 µL Taq enzyme (Fermentas International Inc, Vilnius, Lithuania) and 1 µL template. The second round PCR had 35 cycles and reaction conditions of amplification were the same as the the first round of PCR. Five microliters from the 50-µL products of the second round reaction were run on a 1.5% agarose gel containing ethidium bromide. The remnant PCR products were then given to the sequencing company (BGI Life Tech Co Ltd, Beijing, China) for directly sequencing.
Results
Expression of α9 AChR mRNA in the vestibular endorgans The PCR products of the RT-PCR reaction using the total RNA extracted from the vestibular endorgans as the template and the primers for α9 AChR and β-actin, respectively, are shown in their predicted sizes, approximately 576 bp for α9 AChR (Figure 1) and 213 bp for β-actin (Figure 2). The possible amplification of the nuclear DNA would have resulted in a larger PCR fragment. Negative controls using the PBS as a template for each run of the experiment were all negative. The messenger RNA encoding the α9 AChR and β-actin was detected from the vestibular endo-rgans. The PCR products were directly sequenced; sequence analysis of the band confirmed identity to rat β-actin and α9 AChR cDNA sequence in the predicted region.
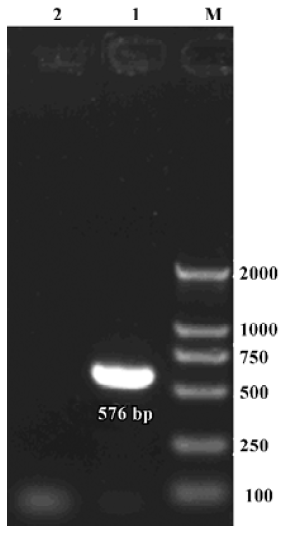
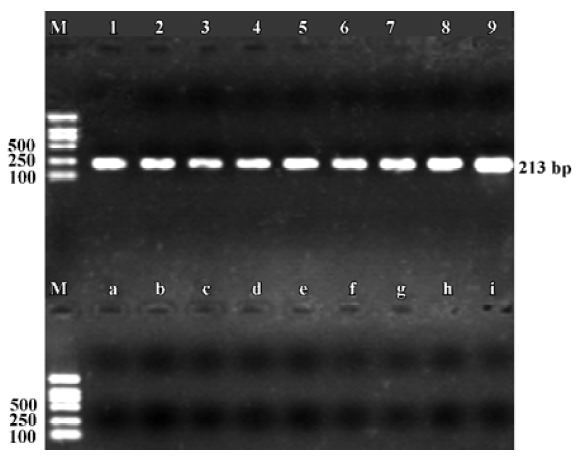
Expression of α9 AChR mRNA in single VHC II Messenger RNA encoding β-actin and α9 AChR was also detected in a few of the VHC II. Each lane demonstrated a single band at the predicted size, approximately 213 bp for β-actin (Figure 2) and 576 bp for α9 AChR (Figure 3). Seven single cells were examined for the expression of β-actin and all 7 cells were positive. Twenty VHC II were examined for the expression of α9 AChR and 4 VHC II were positive (Table 1). It is crucial to transfer the single VHC II from the solution which contains the dissociated individual cells to fresh PBS to avoid false positive results. In the negative control, the reaction that ran on the surrounding fluid bathing the single cell and cell debris failed to detect β-actin or α9 AChR mRNA. Seven and 18 negative controls for β-actin and α9 AChR respectively were done and all were negative. The PCR products have also been directly sequenced. Sequence analysis of the band confirmed identity to rat β-actin and α9 AChR cDNA sequence in the predicted region.
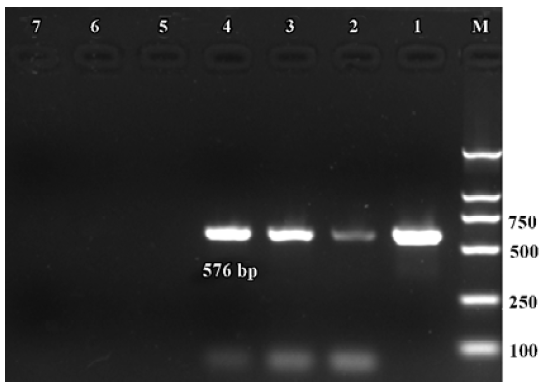
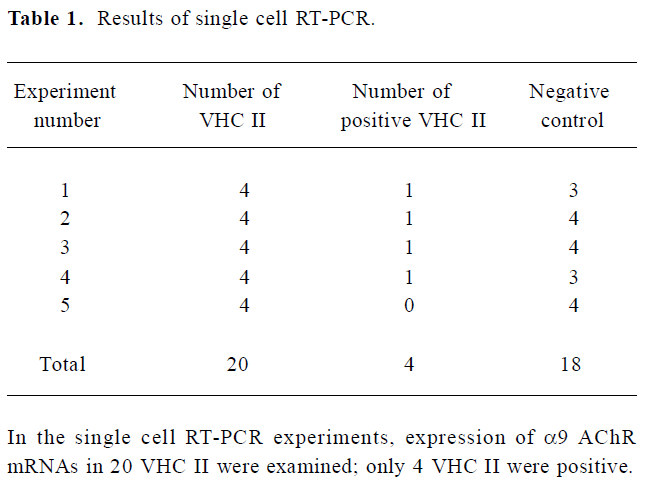
Full table
Discussion
ACh has long been considered the efferent transmitter of the peripheral vestibular system[5,30]. It was suggested that the nAChR subunit α9 is one of the important mediators of efferent cholinergic signaling in the peripheral vestibular system. α9 AChR was shown to be present in the vestibular sensory epithelium of rats in in situ hybridization and immunohistochemistry studies. Hiel et al localized α9 AChR mRNA in type I and type II VHC of rats using in situ hybridization with 35S-labeled riboprobes[16]. Luebke et al reported that in rats, immunoreactivity to the α9 AChR was confined to calyces around type I hair cells and the synaptic pole of type II hair cells[19]. Furthermore, Zuo et al demonstrated the expression of α9 AChR mRNA in VHC utilizing green fluorescent protein coexpressed with α9 AChR mRNA[31]. In this study, by using the RT-PCR technology, the expressions of α9 AChR mRNA was detected in the vestibular endorgans of rat. By using the single cell RT-PCR technique, it was shown that there was expression of α9 AChR mRNA in single VHC II of rats. It also indicates that VHC II of rats might contain α9 AChR. The present study confirmed the expression of α9 AChR in vestibular endorgans of rats and the cell specific expression of α9 AChR mRNA in individual VHC II of rats. Single cell RT-PCR is a highly sensitive method which can detect mRNA molecule coding for a specific protein in an individual cell. One of the drawbacks of this technique arises from high sensitivity: there is a high risk of false positive results due to contamination[27]. In this study, it is crucial to transfer the single VHC II from the solution which contains the dissociated individual cells to fresh PBS to avoid the false positive results. All negative controls being negative in this study indicate that this method could effectively avoid the possible contamination and successfully detect the cell specific expression of mRNA in single VHC II.
VHC II are directly innervated by efferent nerve terminals and ACh is the major efferent transmitter in the peripheral vestibular system of rats[4]. In addition, α9 AChR have been localized to VHC II by using immunohistochemistry techniques[19] and the in situ hybridization technique[16]. Furthermore, ACh can induce modifications of VHC II membrane potential[4]. In this experiment, we further demonstrated the localization of α9 AChR mRNA in VHC II. We indicate that VHC II may possess α9 AChR. The results indicate that VHC II may be directly modulated by ACh-ergic efferent terminals and α9 AChR may be one of the important mediators of efferent cholinergic signaling in the peripheral vestibular system of rats.
The α9 AChR is specifically expressed in hair cells of the inner ear and is believed to be involved in synaptic transmission between efferent nerves and hair cells[31]. It was also reported that α10 AChR was a determinant of nAChR function in mammalian vestibular and cochlear mechanosensory hair cells, and efferent modulation of hair cell function occurs, at least in part, through heteromeric nAChR assembled from both α9 and α10 subunits[22,23]. Coinjection of Xenopus oocytes with α9 and α10 cRNA results in the appearance of ACh-gated channels possessing properties that are distinct from α9-injected oocytes. In particular, α9 and α10-injected oocytes show ~100-fold larger currents, have a unique current-voltage relationship, exhibit more rapid and complete desensitization kinetics, and show a biphasic response to changes in extracellular Ca2+ ions[22]. The single cell RT-PCR technique can be used to detect the coexpression of α9 and α10 subunits in a VHC because it can detect a set of mRNA sequences in an individual cell[27].
In summary, the results of this experiment provide the direct evidence to confirm that VHC II of rats might express mRNA of α9 AChR. It indicates that VHC II may be directly modulated by ACh-ergic efferent terminals. However, the nature of the expression of α9 AChR in rat VHC is still unclear. Further morphological and electrophysiological studies of VHC are needed to address this issue.
References
- Wersall J. Studies on the structure and innervation of the sensory epithelium of the cristae ampulares in the guinea pig; a light and electron microscopic investigation. Acta Otolaryngol Suppl 1956;126:1-85.
- Wersall J, Bagger-Sjoback D. Morphology of the vestibular sense organ. In: Kornhuber HH, editor. Handbook of Sensory Physio-logy. Berlin: Springer-Verlag; 1974. p 123–70.
- Lysakowski A. Synaptic organization of the crista ampullaris in vertebrates. Ann N Y Acad Sci 1996;781:164-82.
- Guth PS, Norris CH. The hair cell acetylcholine receptors: a synthesis. Hear Res 1996;98:1-8.
- Guth PS, Perin P, Norris CH, Valli P. The vestibular hair cells: post-transductional signal processing. Prog Neurobiol 1998;54:193-247.
- Lysakowski A, Goldberg JM. Morphophysiology of the vestibular periphery. In: Highstein SM, Fay RR, Popper AN, editors. The Vestibular System. New York: Springer; 2004. p 57–152.
- Hilding D, Wersall J. Cholinesterase and its relation to the nerve endings in the inner ear. Acta Otolaryngol 1962;55:205-17.
- Nomura Y, Gacek RR, Balogh K. Efferent innervation of vestibular labyrinth: Histochemical demonstration of Acetylcholinesterase activity in the guinea pig inner ear. Arch Otolaryngol 1965;81:335-9.
- Iurato S, Luciano L, Pannese E, Reale E. Histochemical localization of acetylcholinesterase (AChE) activity in the inner ear. Acta Otolaryngol 1971;279 Suppl:1-50.
- Cohen GM. Acetylcholinesterase activity in the embryonic chick’s inner ear. Hear Res 1987;28:57-63.
- Gonzalez A, Meredith GE, Roberts BL. Choline acetyltransferase immunoreactive neurons innervating labyrinthine and lateral line sense organs in amphibians. J Comp Neurol 1993;332:258-68.
- Kong WJ, Egg G, Hussl B, Spoendlin H, Schrott-Fischer A. Localization of chat-like immunoreactivity in the vestibular endorgans of the rat. Hear Res 1994;75:191-200.
- Kong WJ, Hussl B, Thumfart WF, Schrott-Fischer A. Ultrastructural localization of ChAT-like immunoreactivity in the human vestibular periphery. Hear Res 1998;119:96-103.
- Ishiyama A, Lopez I, Wackym PA. Distribution of efferent cholinergic terminals and alpha-bungarotoxin binding to putative nicotinic acetylcholine receptors in the human vestibular end-organs. Laryngoscope 1995;105:1167-72.
- Wackym PA, Popper P, Lopez I, Ishiyama A, Micevych PE. Expression of alpha 4 and beta 2 nicotinic acetylcholine receptor subunit mRNA and localization of alpha-bungarotoxin binding proteins in the rat vestibular periphery. Cell Biol Int 1995;19:291-300.
- Hiel H, Elgoyhen AB, Drescher DG, Morley BJ. Expression of nicotinic acetylcholine receptor mRNA in the adult rat peripheral vestibular system. Brain Res 1996;738:347-52.
- Luo L, Bennett T, Jung HH, Ryan AF. Developmental expression of alpha 9 acetylcholine receptor mRNA in the rat cochlea and vestibular inner ear. J Comp Neurol 1998;393:320-31.
- Lustig LR, Hiel H, Fuchs PA. Vestibular hair cells of the chick express the nicotinic acetylcholine receptor subunit alpha 9. J Vestib Res 1999;9:359-67.
- Luebke AE, Maroni PD, Guth SM, Lysakowski A. Alpha-9 nicotinic acetylcholine receptor immunoreactivity in the rodent vestibular labyrinth. J Comp Neurol 2005;492:323-33.
- Millar NS. Assembly and subunit diversity of nicotinic acetylcholine receptors. Biochem Soc Trans 2003;31:869-74.
- Elgoyhen AB, Johnson DS, Boulter J, Vetter DE, Heinemann S. Alpha 9: an acetylcholine receptor with novel pharmacological properties expressed in rat cochlear hair cells. Cell 1994;79:705-15.
- Elgoyhen AB, Vetter DE, Katz E, Rothlin CV, Heinemann SF, Boulter J. alpha10: a determinant of nicotinic cholinergic receptor function in mammalian vestibular and cochlear mechano-sensory hair cells. Proc Natl Acad Sci USA 2001;98:3501-6.
- Morley BJ, Simmons DD. Developmental mRNA expression of the alpha10 nicotinic acetylcholine receptor subunit in the rat cochlea. Brain Res Dev Brain Res 2002;139:87-96.
- Simmons DD, Morley BJ. Differential expression of the alpha 9 nicotinic acetylcholine receptor subunit in neonatal and adult cochlear hair cells. Brain Res Mol Brain Res 1998;56:287-92.
- Drescher DG, Ramakrishnan NA, Drescher MJ, Chun W, Wang X, Myers SF, et al. Cloning and characterization of alpha9 subunits of the nicotinic acetylcholine receptor expressed by saccular hair cells of the rainbow trout (Oncorhynchus mykiss). Neuroscience 2004;127:737-52.
- Sgard F, Charpantier E, Bertrand S, Walker N, Caput D, Graham D, et al. A novel human nicotinic receptor subunit, alpha10, that confers functionality to the alpha9-subunit. Mol Pharmacol 2002;61:150-9.
- Glowatzki E, Wild K, Brandle U, Fakler G, Fakler B, Zenner HP, et al. Cell-specific expression of the alpha 9 n-ACh receptor subunit in auditory hair cells revealed by single-cell RT-PCR. Proc Biol Sci 1995;262:141-7.
- Glowatzki E. Analysis of gene expression in the organ of Corti revealed by single-cell RT-PCR. Audiol Neurootol 1997;2:71-8.
- Ricci AJ, Rennie KJ, Cochran SL, Kevetter GA, Correia MJ. Vestibular type I and type II hair cells. 1: Morphometric identification in the pigeon and gerbil. J Vestib Res 1997;7:393-406.
- Highstein SM. The central nervous system efferent control of the organs of balance and equilibrium. Neurosci Res 1991;12:13-30.
- Zuo J, Treadaway J, Buckner TW, Fritzsch B. Visualization of alpha9 acetylcholine receptor expression in hair cells of transgenic mice containing a modified bacterial artificial chromosome. Proc Natl Acad Sci USA 1999;96:14100-5.