Glycine blunts transplantative liver ischemia-reperfusion injury by downregulating interleukin 1 receptor associated kinase-41
Introduction
Despite intense research on the development of novel therapeutic strategies, primary graft dysfunction caused by ischemia-reperfusion injury (I/RI) still represents a major problem in clinical liver transplantation[1–3]. The most common candidates for liver transplantation are patients with all kinds of end-stage liver diseases combined with high levels of endotoxin or lipopolysaccharides (LPS), an integral component of the cell wall of gram-negative bacilli. LPS, a potent stimulus for Kupffer cells (KC), is involved in priming and deterioration in I/RI[4–6]. Substantial evidence indicates that KC, the resident macrophages of the liver, play a central role in the pathogenesis of liver parenchyma cell damage during the reperfusion phase, because activated KC are capable of releasing numerous mediators for regulating hepatic microcirculation[7], and microcirculatory disturbance is a key factor in enhanced susceptibility of the donor liver to reperfusion[8].
As a consequence, blockade of LPS-induced KC activation with glycine, has been shown to reduce hepatic I/RI in animal models and humans[8–11]. Glycine is a nontoxic nonessential amino acid and inhibitory neurotransmitter in the central nervous system[12]. It prevents the increase of intracellular Ca2+ concentration by activating a glycine-gated chloride channel. Increases of intracellular Ca2+ concentration in KC are essential for the release of prostanoids and inflammatory cytokines in response to stimuli such as LPS[8,13]. Furthermore, Glycine can also inhibit the expression of LPS membrane-bound receptor CD14 on KC[14]. However, little has so far been documented about the relationship between glycine and the mediators of LPS signaling transduction. It’s well known that toll-like receptor (TLR) dependent pathways are critical for receiving LPS signals to initiate inflammation responses. Recently, a key breakthrough has been made in the area of TLR signaling with the discovery of interleukin 1 receptor associated kinase-4 (IRAK-4). In IRAK-4-deficient mice, the LPS signals mediated by TLR are seve-rely impaired, indicating that IRAK-4 plays an essential role in these signaling pathways[15]. The aim of this study was to determine whether brief intravenous infusion of glycine to donors would downregulate IRAK-4, interfering with LPS signal transduction and blunt I/RI after liver transplantation.
Materials and methods
Experimental animals and treatment Male Sprague-Dawley rats (250–270 g) were purchased from the Chongqing Experimental Animal Center of Chongqing University of Medical Sciences and randomly divided into the glycine group and control group. Donor animals of the glycine group (n=40) were given glycine by infusion (1.5 mL; 300 mmol/L) through the femoral vein 1 h before harvest, and the control group were treated with 1.5 ml physiological saline (n=40)[8]. Experimental procedures were approved by the Institutional Animal Care and Use Committee.
Transplantation procedure Liver transplantation was performed according to the Kamada technique[6]. Donor livers were harvested in a standardized fashion, including freeing the organ from ligaments and cannulation of the bile duct, retracting, and moving the liver lobes in situ continuously to minimize the harvest-dependent graft injury[2]. Cuffs were attached in the infrahepatic vena cava and portal vein of the donor liver, which were stored at 0 oC– 4 oC UW solution for 1 h. Orthotopic liver transplantation was then performed in hepatectomized recipient rats by connecting the suprahepatic vena cava with a running 7/0 Prolene suture, inserting cuffs into the corresponding vessels and anatomizing the bile duct over an intraluminal polyethylene splint, without reconstruction of the hepatic artery. During this time the recipient portal vein was clamped for 15–17 min.
Determination of survival rate and experimental design After the abdominal incision was closed the rats were provided with food and water. We followed up 10 animals of each group for 7 d after surgery to assess survival. The other animals of each group were divided into 3 subgroups (10 animals of every subgroup) at 1 h, 2 h and 6 h after portal vein reperfusion, blood from portal vein blood and liver tissues were sampled respectively.
LPS determination[16] The plasma of portal vein blood was obtained and subjected to a deproteination and neutralization process by acid base precipitation using perchloric acid and sodium hydroxide, and then, the LPS levels were assayed by the chromogenic limulus amebocyte lysate (LAL) test with a kinetic modification according to the test kit procedure.
Enzyme assays ALT and AST activity of serum was determined by standard enzymatic methods, while total bilirubin (TB) was detected by direct spectrophotometry at 454 nm.
Reverse transcription-polymerase chain reaction (RT-PCR) Total RNA was extracted from liver tissues using TRIzol reagent (Life Technologies, New York, USA.) according to the manufacturer’s instructions. Total RNA was quantified with the ratio of absorption values of RNA samples at 260 nm and 280 nm. Each total RNA sample was reverse-transcribed to complementary DNA (cDNA) using RT-PCR kit (Roche, USA) and stored at -70 oC until PCR. β-actin was used as an internal positive control. Specific primer sequences of β-actin, IRAK-4, TNF-α[17] were as follows and the sizes of production were 300, 597, and 694 bp respec-tively: IRAK-4: 5'-CGGCGACGACAGATACAATC-3' and 5'-TGC TGACACGTTGUATTAC-3'; β-actin: 5'-CATTGT-GATGGA CTCCGGAG-3' and 5'-ATAGTGATGACCTGG-CCGTC-3'; TNF-α: 5'-CCCAGACCCTCACACTCAGA-3' and 5'-GCCA CTACTTCAGCATCTCG-3'. All PCR products were electrophoresed on 2% agarose gels. The relative expression of mRNAs were assessed by taking the ratio of the intensity of the DNA bands of IRAK-4 or TNF-α to β-actin band using the Bio-Image analysis system (Bio-Rad Gel Doc 2000, Hercules, CA, USA) and expressed as arbitrary units.
Western blot Protein extracts of the liver tissues were obtained by homogenizing samples in a cell lysis buffer containing 20 mmol HEPES (pH 7.9), 25% glycerol, 0.42 mmol NaCl, 15 mmol MgCl2, 0.2 mmol EDTA, 0.5 mmol phenyl-methylsulfonyl fluoride (PMSF) and 0.5 mmol dithiothreitol (DTT), then by two cycles of centrifugation at 12 000×g for 15 min. Protein concentration was determined by Bradford assay kit (Bio-Rad, Hercules, CA, USA). Extracted protein was separated by 10% sodium dodecyl sulfate polyacrylamide gel electrophoresis, and transferred to polyvinylidene fluoride membranes (Dupont, Boston, MA, USA). Membrane was washed with 0.1% Tween20-PBS and incubated with 5% dry non-fat skimmed milk powder in 0.1% Tween20-PBS, pH 7.4 for 1 h, then with rabbit anti-mouse IRAK-4 polyclonal antibody (diluted 1:200, IMG-441, Imgenex, San Diego, CA, USA) and horseradish peroxidase-conjugated goat anti-rabbit IgG (Santa Cruz, CA, USA) for 1h. Finally, the membranes were developed with diaminobenzidine reagent. The relative amount of IRAK-4 protein was quantified from relative optical density of the band by Bio-Image analysis system (Gel Doc 2000, Bio-Rad, Hercules, CA, USA).
Analysis of nuclear factor-κB (NF-κB) transcriptional activity Electrophoretic mobility shift assay (EMSA) was performed for analysis of NF-κB transcriptional activity of liver as described in a previous study[15]. Nuclear extracts were prepared and protein concentrations were determined by assay kit (Bio-Rad, Hercules, CA, USA). The double-stranded consensus-binding sequences for the EMSA comprised the oligonucleotide 5'-AGTTGAGGGGACTTTCCC-AGG-3' for NF-κB and for the mutant NF-κB 10 µg of nuclear extract was incubated with an end-labeled, double-stranded, NF-κB oligonucleotide probe. The reaction was performed in a total of 20 µL binding buffer (5 mmol/L HEPES pH 7.8, 50 mmol/L KCl, 0.5 mmol/L dithiothreitol and 10% glycerol) for 20 min at room temperature. After incubation, samples were fractionated on a 5% polyacrylamide gel and complex formation was visualized by autoradiography film (Eastman Kodak, Belgium). At the same time, NF-κB DNA-binding activity in nuclear extracts was measured using the Trans-AM NF-κB p65 ELISA kit (Active Motif Europe, Rixensart, Belgium) according to manufacturer’s instructions[18]. Five µg nuclear extract were added to a 96-well plate to which oligonucleotide containing NF-κB consensus-binding site had been immobilized. The NF-κB complex bound to the oligonucleotide was detected by adding a specific mAb for p65 subunit. A secondary horseradish peroxydase-conjugated mAb was added and developed with tetramethylbenzidine substrate. After an optimal development time, the reaction was stopped using H2SO4 0.5 mol/L, and absorbance was measured at 450 nm.
Morphological study by light and electron microscopy Liver biopsies for light microscopy were cut into blocks of <1 mm diameter and immediately stored in formalin. Paraffin sections were stained with hematoxylin and eosin. The specimens for electron microscopy were immediately cut into 1 mm cubes and fixed in 2.5% glutaraldehyde in sodium carcodylate-hydrochloride buffer overnight at 4 oC for electron microscopy section. The sections were examined under a transmission electron microscope (Philips EM208, Eindhoven, Holland).
Data analysis Results were expressed as mean±SD. Statistical difference was calculated by analysis of Independent-Samples t-test using SPSS10.0 software. A P value <0.05 was considered significant.
Results
Survival A Kaplan–Meier model was constructed from the data to compare overall survival rates between the two groups. Seven-day survival rates were 80% (mean survival time, 6.3±0.49 d) in the glycine group, whereas the control group had a significantly lower survival rate of 50% (mean survival time, 5.1±0.7 d), (P<0.05, Figure 1). Therefore, our direct comparison indicates that glycine is effective in preserving long-term survival.
Ischemia-reperfusion induced a significant increase in portal vein blood LPS concentrations Results presented (Table 1) indicated that LPS concentrations in portal vein blood kept significantly increasing to the end of our observation period after reperfusion (6 h, P<0.01). However, the difference between the two groups at the same point was not significant (P>0.05).
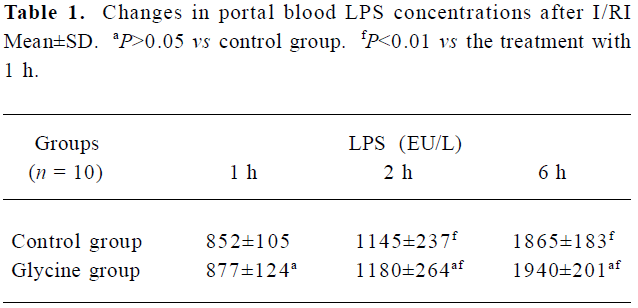
Full table
Glycine alleviates hepatic I/RI after liver transplantation Hepatocellular injury, reflected by serum AST, ALT and TB, was significantly reduced in the glycine group at 6 h after reperfusion (Table 2). Glycine pretreated livers had less severe signs of I/RI compared with the control group, as reflected by numbers of necrotic cells, infiltrating leukocytes and vacuoles (Figure 2). Furthermore, the hepatic ultrastructural features were significantly different between the two groups. The hepatic sinusoid was well maintained in the glycine group compared to the obvious disruption of sinusoidal lining cells in the control group, in which degeneration of cytoplasm and tremendous mitochondria swelling were also found (Figure 3).
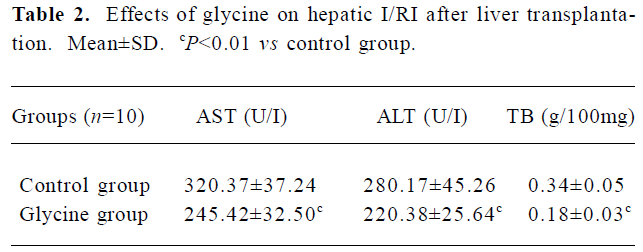
Full table
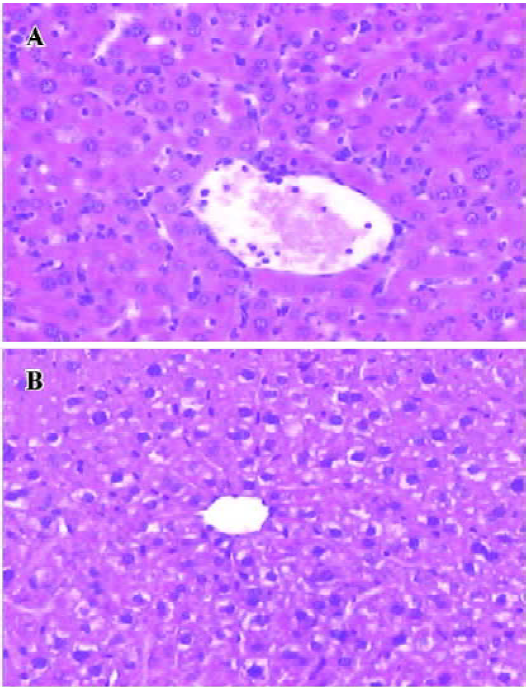
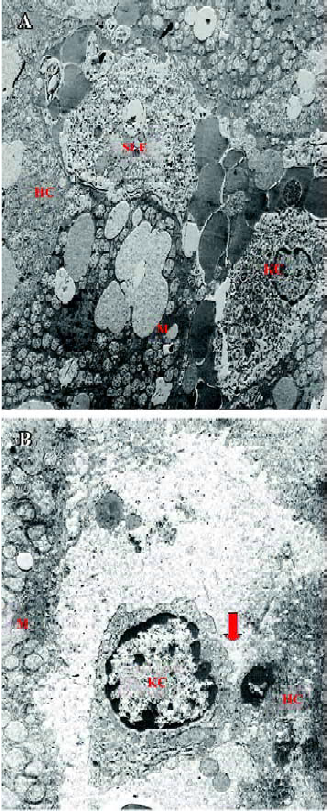
Glycine downregulates IRAK-4 after reperfusion To examine the interference effects of glycine on LPS signal transduction during reperfusion phase, IRAK-4 gene expression changes on reperfusion livers were examined with RT-PCR and Western-blot (Figure 4 and 5). After reperfusion, the expression of IRAK-4 were dramatic increased in control group and reached a peak value 2 h after reperfusion, strongly indicating that LPS induced dramatic inflammation after reperfusion, however, Glycine significantly diminished the expression of IRAK-4, supporting the hypothesis that downregulation LPS signal transduction mediator’s expression is a mechanism of glycine-mediated protection.
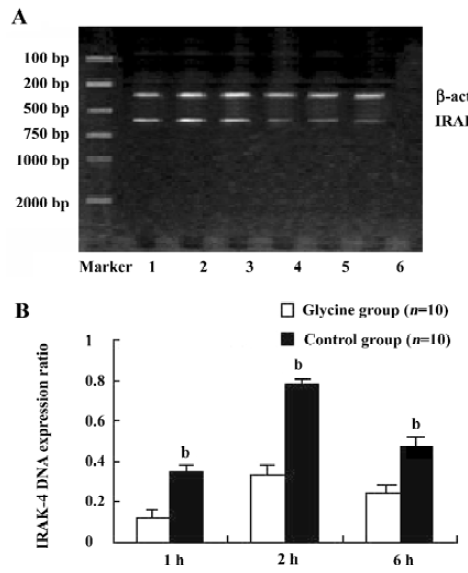
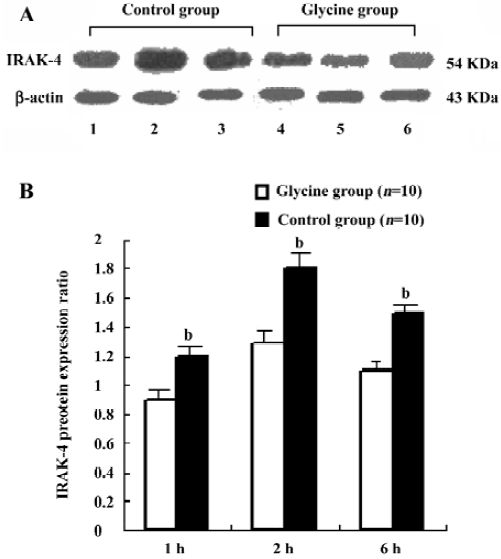
Glycine depresses NF-κB activation To further examine the interference effect of glycine on LPS signal transduction, NF-κB activation on reperfusion livers were first examined by ELISA (Figure 6A). We observed that the glycine group expressed low NF-κB p65 DNA-binding activity, and the control group induced a rapid and clear increase in NF-κB p65 activation, which was optimal after 2 h of reperfusion. This observation was confirmed and extended in EMSA experiments performed on nuclear extracts (Figure 6B). We observed a low level of NF-κB activation in the glycine group and a dramatic enhancement after 2 h of reperfusion in the control group.
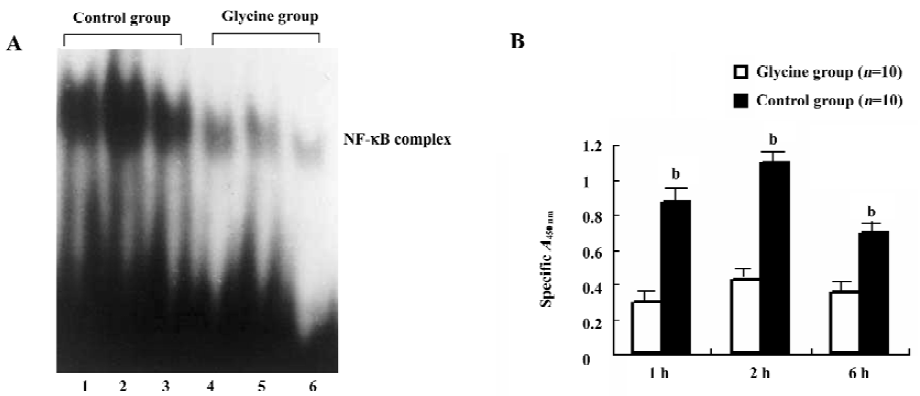
Glycine inhibits TNF-α mRNA expression As NF- κB family members are known to control the expression of the TNF-α gene, TNF-α mRNA levels on reperfusion livers were also quantified using RT-PCR (Figure 7). Reperfusion markedly raised TNF-α mRNA levels, which reached a peak value 1 h after reperfusion. However, glycine significantly suppressed the elevation of TNF-α mRNA throughout the experimental period when compared with the control group (P < 0.05).
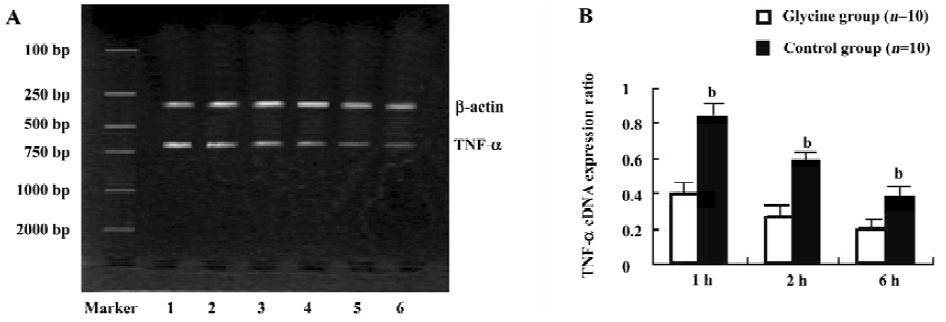
Discussion
The present study showed that a marked increase of LPS concentrations in portal vein persisted to the end of our study. Indeed, it is a ubiquitous phenomenon in liver transplantation for recipients of end-stage liver disease[5]. Furthe-rmore, prospective organ donors may be exposed to various types of stress, such as trauma, hemorrhage or transient intestinal ischemia. As a result, translocation of gut-derived bacteria and LPS into body fluids is also frequent[19]. Substantial evidence indicates that recipients are susceptible to LPS with an increase in mortality in rat liver transplantation, and the dominating nosogenesis of LPS is to active KC.
LPS acts as a potent stimulus for KC mainly through TLR signaling pathways, which results in the activation of transcription factors. LPS stimulation triggers TLR-4 aggregation that facilitates recruitment of myeloid differentiation factor 88 (MyD88) to LPS-receptor complexes via interactions of the C-terminal TIR domains within these receptors and MyD88. MyD88 also interacts with IRAK-4 and IRAK-1 through its death domain, via the region of MyD88 situated between its C-terminal TIR and N-terminal death domains. Interaction of IRAK-4 with IRAK-1 induces IRAK-1 phosphorylation and activation, which also has been reported to facilitate recruitment of IRAK-1 to MyD88. Once hyperphosphorylated, IRAK-1 releases from the receptor complex and interacts with the TNF associated factor 6 complex (TRAF6) to trigger downstream signaling cascades, leading to activation of NF-κB and TNF-α expression. In contrast, in IRAK-4-deficient mice, the LPS signals mediated by TLR are severely impaired. Thus, IRAK-4, bridging the gap between the stimulated receptor complex and IRAK-1 activation, is considered to be a key mediator in the LPS signaling pathway [20–23].
A number of experimental studies demonstrated that glycine has beneficial effects against LPS inducing hepatic injury. In the presence of glycine, a glycine-gated chloride channel is activated causing an influx of chloride, leading to hyperpolarization of cell membrane, which makes calcium channels on the plasma membrane more difficult to open and inhibits the influx of calcium. Moreover, the influx of chloride could also inactivate the IP3-gated calcium channels and block the release of calcium from intracellular stores. We reported here that pretreatment of glycine led to downregulation of IRAK-4 expression in reperfusion graft. It is well known that calcium acts as an intracellular second messenger, phosphorylation of MAP kinases and translocation of NF-kB to the nucleus were preceded by an increase in intracellular Ca2+ levels after LPS stimulation[24]. As both NF-κB and MAP kinases are important mediators of intracellular LPS signal transduction mediator and inflammatory cytokine gene expression, from this fact we could deduce that inactivation of MAP kinases and NF-κB also impaired the synthesis of IRAK-4 during hepatic I/RI. As a result, the downstream signaling cascades were also blunted, resulting in inactivation of NF-κB and depression TNF-α expression. TNF-α is a potent inflammatory cytokine mainly produced by the macrophage lineage. This cytokine exerts pleiotropic activities including the induction of adhesion molecules, complement activation, acute phase response, priming of the oxidative burst in granulocytes and promotes apoptotic or necrotic cell death in various cell types. There is increasing evidence indicating that TNF-α plays a key role in the initiation or progression of hepatic IR/I. Inhibition of TNF-α synthesis or activity attenuates this injury[25,26]. Being consistent with those reports, our current study also demonstrated that hepatic I/RI can be markedly reduced by glycine pretreatment with the inhibition of TNF-α synthesis, reflected by both plasma levels of AST, ALT, TB and liver histology.
In summary, we clearly demonstrated that glycine had a potent effect against LPS induced liver impairment caused by interference with the LPS signal transduction pathway through inhibition of IRAK-4 expression, prevention of NF-κB activation, and reduction of TNF-α during reperfusion phase. Given the impact of glycine on multiple factors critical to the LPS related I/RI[27], it could be a novel compound for the development of an effective treatment for hepatic I/RI during liver transplantation.
References
- Kupiec-Weglinski JW, Busuttil RW. Ischemia and Reperfusion Injury in Liver Transplantation. Transplant Proc 2005;37:1653-6.
- Goss JA, Seu P, Gao FQ, Wyllie S. Ischemia-reperfusion of rat liver modulates hepcidin in vivo expression. Liver Transpl 2005;11:800-6.
- Anderson CD, Pierce J, Nicoud I, Belous A, Knox CD, Chari RS. Modulation of mitochondrial calcium management attenuates hepatic warm ischemia-reperfusion injury. Liver Transpl 2005;11:663-8.
- Kojima Y, Suzuki S, Tsuchiya Y, Konno H, Baba S, Nakamura S. Regulation of pro-inflammatory and anti-inflammatory cytokine responses by Kupffer cells in endotoxin-enhanced reperfusion injury after total hepatic ischemia. Transpl Int 2003;16:231-40.
- Frankenberg MV, Weimann J, Fritz S, Fiedler J, Mehrabi A, Buchler MW, et al. Gadolinium chloride-induced improvement of postischemic hepatic perfusion after warm ischemia is associated with reduced hepatic endothelin secretion. Transpl Int 2005;18:429-36.
- von Frankenberg M, Golling M, Mehrabi A, Nentwich H, Klar E, Kraus TW. Donor pretreatment with gadolinium chloride improves early graft function and survival after porcine liver transplantation. Transpl Int 2003;16:806-13.
- Rentsch M, Beham A, Sirek S, Iesalnieks I, Geissler EK, Anthuber M, et al. Glycine but not gadolinium chloride or methyl palmitate reduces postischemic white blood cell accumulation and early graft nonfunction after liver transplantation in the rat. Transplant Proc 2002;34:2389-90.
- Schemmer P, Bradford BU, Rose ML, Bunzendahl H, Raleigh JA, Lemasters JJ, et al. Intravenous glycine improves survival in rat liver transplantation. Am J Physiol 1999;276:924-32.
- Rentsch M, Puellmann K, Sirek S, Iesalnieks I, Kienle K, Mueller T. Benefit of Kupffer cell modulation with glycine versus Kupffer cell depletion after liver transplantation in the rat: effects on postischemic reperfusion injury, apoptotic cell death graft regeneration and survival. Transpl Int 2005;18:1079-89.
- Silva MA, Richards DA, Bramhall SR, Adams DH, Mirza DF, Murphy N. A study of the metabolites of ischemia-reperfusion injury and selected amino acids in the liver using microdialysis during transplantation. Transplantation 2005;79:828-35.
- Schemmer P, Golling M, Kraus T, Mayatepek E, Herfarth C, Klar E. Glycine Reduces Reperfusion Injury in Human Liver Transplantation: Our First Patients. Transplant Proc 2001;33:3750-2.
- Zhang SJ, Shi JH, Tang Z, Wu Y, Chen S. Protective effects of glycine pretreatment on brain-death donor liver. Hepatobiliary Pancreat Dis Int 2005;4:37-40.
- Bruck R, Wardi J, Aeed H, Avni Y, Shirin H, Avinoach I, et al. Glycine modulates cytokine secretion, inhibits hepatic damage and improves survival in a model of endotoxemia in mice. Liver Int 2003;23:276-82.
- Peng Y, Gong JP, Liu CA, Li SW, Gan L, Li SB. The effect of glycine on CD14 and NF-kappa B in Kupffer cells from rat liver grafts after ischemia-reperfusion injury. Chin J Hepatology 2005;13:179-82. Chinese..
- Lye E, Mirtsos C, Suzuki N, Suzuki S, Yeh WC. The role of interleukin 1 receptor-associated kinase-4 (IRAK-4) kinase activity in IRAK-4-mediated signaling. J Biol Chem 2004;279:40653-8.
- Sun XQ, Fu XB, Zhang R, Lu Y, Deng Q, Jiang XG, et al. Relationship between plasma D (-)-lactate and intestinal damage after severe injuries in rats. World J Gastroenterol 2001;7:555-8.
- Wu R, Zhou M, Wang P. Adrenomedullin and adrenomedullin binding protein-1 downregulate TNF-α in macrophage cell line and rat Kupffer cells. Regul Pept 2003;112:19-26.
- Vosters O, Beuneu C, Nagy N, Movahedi B, Aksoy E, Salmon I, et al. CD40 expression on human pancreatic duct cells: role in nuclear factor-kappa B activation and production of pro-inflammatory cytokines. Diabetologia 2004;47:660-8.
- Vajdova K, Smrekova R, Kukan M, Jakubovsky J, van Rooijen N, Horecky J, et al. Endotoxin-induced aggravation of preservation reperfusion injury of rat liver and its modulation. J Hepatol 2000;32:112-20.
- Anssens S, Beyaert R. Functional Diversity and Regulation of Different Interleukin-1 Receptor-Associated Kinase (IRAK) Family Members. Mol Cell 2003;11:293-302.
- Suzuki N, Suzuki S, Eriksson U, Hara H, Mirtosis C, Chen NJ, et al. IL-1R-associated kinase 4 is required for lipopolysaccharide-induced activation of APC. J Immunol 2003;171:6065-71.
- Suzuki N, Suzuki S, Duncan GS, Millar DG, Wada T, Mirtsos C, et al. Severe impairment of interleukin-1 and Toll-like receptor signalling in mice lacking IRAK-4. Nature 2002;416:750-6.
- Hatao F, Muroi M, Hiki N, Ogawa T, Mimura Y, Kaminishi M, et al. Prolonged Toll-like receptor stimulation leads to down-regulation of IRAK-4 protein. J Leukoc Biol 2004;76:904-8.
- Martin L, Pingle SC, Hallam DM, Rybak LP, Ramkumar V. Activation of the adenosine A3 receptor in RAW 264.7 cells inhibits lipopolysaccharide-stimulated tumor necrosis factor-alpha release by reducing calcium-dependent activation of nuclear factor-kappaB and extracellular signal-regulated kinase 1/2. J Pharmacol Exp Ther 2006;316:71-8.
- Fernandez ED, Flohe S, Siemers F, Nau M, Ackermann M, Ruwe M, et al. Endotoxin tolerance protects against local hepatic ischemia/reperfusion injury in the rat. J Endotoxin Res 2000;6:321-8.
- Ishizaki-Koizumi S, Sonaka I, Takei Y, Ikejima K, Sato N. The glycine analogue, aminomethanesulfonic acid, inhibits LPS-induced production of TNF-alpha in isolated rat Kupffer cells and exerts hepatoprotective effects in mice. Biochem Biophys Res Commun 2004;322:514-9.
- Schemmer P, Mehrabi A, Kraus T, Sauer P, Gutt C, Uhl W, et al. New aspects on reperfusion injury to liver-impact of organ harvest. Nephrol Dial Transplant 2004;19 suppl:26-35.