Inhibitory effect of triptolide on lymph node metastasis in patients with non-Hodgkin lymphoma by regulating SDF-1/CXCR4 axis in vitro1
Introduction
Triptolide is a diterpenoid triepoxide extracted from the traditional Chinese herb Tripterygium wilfordii Hook F (TWHF), and exhibits potent immunosuppressive and anti-inflammatory properties, which are effectively used in treating autoimmune diseases[1]. Recent data have shown that triptolide has a broad spectrum of activity against tumors. In vitro, triptolide inhibited the proliferation of a number of tumor cell lines including H23 (non-small cell lung cancer, NSCLC), HT1080 (fibrosarcoma) and COLO205 (colon carcinoma) at extremely low concentrations (2–10 ng/mL). Likewise, in vivo treatment of mice with triptolide inhibited the growth of xenografts formed by different tumor cell lines (B16 melanoma, MDA-435 breast cancer, TSU bladder cancer, and MGC80-3 gastric carcinoma). Importantly, it is found that the antitumor effect of triptolide was comparable or superior to that of conventional antitumor drugs, such as adriamycin, mitomycin, and cisplatin. Additionally, tumor cells that were resistant to Taxol, attributable to the overexpre-ssion of the multidrug resistant gene 1, were still sensitive to triptolide[2].
Chemokines are a group of small pro-inflammatory chemoattractant proteins that participate in homeostatic process, including lymphocyte homing to secondary lymphoid organs, eg, lymph nodes and Peyer’s patches under physiological conditions, as well as in pathologic conditions such as inflammation, tumor growth and metastasis[3,4]. Stromal cell-derived factor-1 (SDF-1), which binds only to CXC chemokine receptor 4 (CXCR4), belongs to the CXC chemo-kine subfamily. It is produced mostly by bone marrow-, lymph node-, muscle-, and lung-derived stromal cells, endothelial cells and fibroblasts. In humans there are two identified splice variant forms of SDF-1, SDF-1α, and SDF-1β, derived from a single gene, and the former is more abundant than the latter. This chemokine ligand/receptor axis plays an important biological role in homing hematopoietic stem cells into the marrow microenvironment[5]. Accumulating evidence suggested that functional CXCR4 was highly expressed on a wide variety of tumor cells including those in breast cancer, NSCLC, prostate cancer, glioma, glioblastoma, and so on. Moreover, it has been shown that these CXCR4 positive tumor cells can metastasize preferentially to the organs that secrete SDF-1 (eg, bone marrow, lymph node, lung or liver)[6–10]. Given the evidence that SDF-1/CXCR4 axis plays a key role in regulating the metastatic behavior of several tumors, modulation of this axis may be important for inhibiting metastasis of CXCR4 positive tumor cells.
Non-Hodgkin’s lymphoma (NHL) is a heterogeneous group of malignancy of B cells or T cells that usually originate in the lymph node, and are characterized by a lymph node metastatic pattern involving principally organs with large concentrations of lymphatic tissue such as lymph nodes, tonsils, spleen and bone marrow[11]. Regular radiotherapy and chemotherapy simply target at killing tumor cells, the remission rate is limited. If a new antitumor agent is designed to focus on mechanisms involved in tumor metastasis but not only to kill cells, a new field may be expanded in treatment of NHL. CXCR4 has been reported to be also expressed on the majority of NHL cell lines and primary cells including those in diffuse large B-cell lymphoma, peripheral blood T-cell lymphoma, lymphocytic lymphoma, follicular lymphoma and mantle cell lymphoma as well, and CXCR4 is required for invasion of lymphoma cells into tissues and therefore essential for lymphoma metastasis[12]. Because the SDF-1/CXCR4 axis is pivotal for modulating the trafficking of lymphoma cells, it can obviously become a new target for the antitumor drug in the treatment of NHL.
In the present study, we chose B-NHL cell line Raji cells to investigate the antiproliferative effects of triptolide. Furthermore, we chose stromal cells and lymphoma cells freshly isolated from the lymph nodes of patients with NHL as the target to study the effects of triptolide on the lymph node metastasis and to explore the mechanism by which triptolide regulates SDF-1/CXCR4 axis in NHL. We focused on the changes in the expression of SDF-1 and CXCR4, and observed the effects of triptolide modulating the migration of primary lymphoma cells in vitro.
Materials and methods
Drugs and reagents Triptolide (C20H24O6, molecular weight 360.4, Figure 1) was purchased from Sigma Chemical Company (St Louis, MO, USA) and was initially dissolved in dimethylsulfoxide (DMSO), and stored at -20 °C, and then thawed before use. 3-(4,5-Dimethyl-2-thiazolyl)-2,5-diphenyl-2H-tetrazolium (MTT) was purchased from Janssen Chimica Company (New Brunswick, NJ, USA). Dulbecco’s Modified Eagle’s Medium (DMEM, low-glucose), RPMI-1640 culture medium, new-born calf serum, fetal calf serum (FCS) and TRIzol reagent were purchased from Gibco, Invitrogen Corporation (Carlsbad, Califorlia, USA). Me2SO was purchased from Sigma. RevertAid first-strand cDNA synthesis kit was purchased from MBI Fermentas (Hanover, MD, USA). Trypsin was purchased from Amresco (Ohio, USA). Recombinant human SDF-1α (rhSDF-1α) was purchased from PeproTech (Rocky Hill, NJ). Phycoerythrin (PE)-conjugated anti-human CXCR4 (Cat 12-9999, clone: 12G5) and its isotype control PE-conjugated mouse IgG2a (Cat 12-4729) were purchased from eBioscience (CA, USA). Simultest IMK-lymphocyte mouse anti-human CD3-fluorescein isothiocyanate (FITC)/CD19-PE and its isotype control IgG1/IgG2a (Cat 340182) were purchased from Becton Dickinson Immunocyto-metry system (San Jose, CA, USA). Transwell (24-well cell clusters, 6.5 mm diameter, 5.0 µm pore size) was purchased from Corning Incorporated Costar (NY, USA). The Raji cell line was obtained from China Center for Typical Culture Collection (Wuhan, China), and was grown in RPMI-1640 culture medium containing 10% FCS and 2 mmol/L L-glutamine at 37 °C in a 5% CO2 incubator.
Patients After informed consent, cervical lymph nodes were obtained from newly diagnosed patients with NHL from the Department of Hematology at Union Hospital, Tongji Medical College, Huazhong University of Science and Technology. A total of 7 patients included 5 cases of diffuse large B-cell lymphoma (3 of stage IV, 2 of stage III) and 2 cases of peripheral T-cell lymphoma (1 of stage IV, 1 of stage II). The diagnosis and classification of patients were based on the Revised European American Lymphoma Classification[13]. In addition, reactive hyperplasia lymph nodes in 3 cases of non-malignant diseases were obtained as negative controls.
Lymph node stromal cells and lymphoma cells preparation Single cell suspensions of lymph node specimens obtained from patients with NHL were cultured in DMEM (low-glucose) supplemented with 10% heat-inactivated new-born calf serum in plastic flasks at a concentration of 1×106cells/mL at 37 °C in a humidified 5% CO2 atmosphere for 3 d. Any non-adherent cells were then removed and the adherent cells were cultured further until they reached confluency. Fresh medium was then supplied twice a week depending on their growth and the cells were passaged every 2–3 weeks. The adherent cells prepared in this way were hereafter referred to as lymph node stromal cells, and early passage cells were used in the subsequent studies. Cells were grouped as follows: the control group; and those treated with triptolide at concentrations of 12.5, 25, 50 nmol/L for 24 h, respectively. In addition, single cell suspensions freshly isolated from surgical lymph node specimens were washed and resuspended in D-Hank’s supplemented with 1% FCS. Trypan blue staining was used to assess the cell viability to be more than 98%. The cells prepared were used as lymphoma cells and grouped as above.
MTT assay The antiproliferative effects of triptolide against different cell groups were determined by the MTT dye uptake method. A total of 40 000 Raji cells per well were incubated in triplicates in a 96-well plate. Different concentrations of triptolide were added, and the final concentrations were 6.25, 12.5, 25, 50, and 100 nmol/L. The plates were in the presence or absence of the indicated test samples for 0, 24, 36, 48, 60, and 72 h. The largest Me2SO dissolved concentration group was used as the control group. MTT solution 20 µL [5 g/L in phosphate-buffered saline (PBS)] was then added to each well. After 4 h at 37 °C, the supernatant was removed and 150 µL DMSO was added. When the blue crystal was dissolved, the optical density (OD) was detected in the microplate reader at 490 nm wavelength by a 96-well multiscanner autoreader (Biotech Instruments, New York, USA). The following formula was used: cell proliferation inhibited (%)=[1-(OD of the experimental samples/OD of the control)]×100%.
Reverse transcriptase-polymerase chain reaction (RT-PCR) for SDF-1α mRNA Oligonucleotide primers were designed by premier 5.0 from human cDNA sequences available in GeneBank and synthesized by Shanghai Sangon Biological Engineering & Technology Service (Shanghai, China) as follows: SDF-1α, the sense primer was 5'-GCCATG-AACGCCAAGGTCGTGGT-3', and the antisense was 5'-CCT-CGAGTGGGTCTAGCGGAAAG-3'; β-actin, the sense primer was 5'-TACATGGCTGGGGTGTTGAA-3' and the antisense was 5'-AAGAGAGGCATCCTCACCCT-3'. Amplified fragments were 317 bp for SDF-1α and 219 bp for β-actin. The lymph node stromal cells were digested by 0.25% trypsin. Total RNA was isolated from the cells by TRIzol reagent. The reverse transcription reaction was performed using the RevertAid first-strand cDNA synthesis kit and the synthesized cDNA was amplified by PCR. The 50 µL reaction mixture contained: 3 µL cDNA, 1 µL 10 mmol/L dNTPs, 3 µL 2.0 mmol/L MgCl2, 5 µL 10×PCR buffer, 3 µL of a pair of primer (10 pmol/µL), 1 µL Taq polymerase (1 U/µL), and 31 µL ddH2O. The PCR schedule consisted of a 5 min initial denaturation at 94 °C, followed by 30 cycles of denaturation for 1 min at 94 °C, annealing for 1 min at 60 °C, polymerization for 1 min at 72 °C, and finally extension for 10 min at 72 °C. Amplification of β-actin was also performed as a RT-PCR control. A total of 6 µL of the PCR product was separated using 2% agarose gel. DNA bands were visualized by UV light and documented by UVItec gel documentation and analysis system (Cambridge, UK). The ratio between the target gene and β-actin gene band densities was used for quantitative evaluation.
Flow cytometric analysis of CXCR4 expression A total of 1×106 single cell suspension prepared from lymph node specimens were stained with mouse anti-human CD3-FITC/CD19-PE firstly at 4 °C for 30 min in the dark and washed twice with PBS. Cells incubated with its isotype control IgG1/IgG2a were used as negative controls. The specimens contained more than 80% of CD19 or CD3 positive cells were determined as lymphoma cells of B-NHL or T-NHL and used to detect the CXCR4 expression in the subsequent study. Then another total of 1×106 single cell suspension were incubated with PE-conjugated anti-human CXCR4 monoanti-body for 30 min at 4 °C in the dark and washed twice with PBS. Cells incubated with PE-conjugated isotype mouse IgG2a were used as negative controls. Analysis was performed on a FACSort flow cytometry (Becton Dickinson, NJ, USA). A minimum of 10 000 cells were analyzed in all specimens. The percentage of positive cells was determined using the CellQuest software program (Becton Dickinson) and expressed as the results.
Chemotaxis assay Transwell was used to perform the chemotaxis assays. To examine the effect of triptolide on the migration of primary lymphoma cells towards rhSDF-1α in vitro, a total of 2×105 lymphoma cells freshly isolated were pretreated with triptolide. Final concentrations were 0, 12.5, 25 and 50 nmol/L, respectively, in 100 μL RPMI-1640 supplemented with 10% FCS (referred to hereafter as the migration medium) at 37 °C with 5% CO2 for 2 h. Cell suspension prepared for each group was loaded into the upper chamber of each transwell filter. Migration medium 500 µL containing 200 ng/mL rhSDF-1α was added to the lower chamber of each filter. The migration medium not containing rhSDF-1α in the lower chambers were used as blank controls. There-after, the wells were incubated at 37 °C for 4 h. The upper chambers were then removed carefully and the cells in the lower chambers were collected and counted using the trypan blue exclusion method. Cell number was counted in triplicates for each well and the following formula was used: cell migration rate(%)=(cell number of the lower chamber/cell number of the total)×100%. Furthermore, to examine the effects of triptolide on the migration of lymphoma cells towards cultured lymph node stromal cells in vitro, 5×104 stromal cells in 500 µL DMEM (low-glucose) culture medium were seeded into the lower chambers of each transwell filter at 37 °C, in a 5% CO2 incubator for 3–5 d. When the monolayers reached confluency, 100 µL migration medium contained 2×105 lymphoma cells pretreated with different concentrations of triptolide (0, 12.5, 25, 50 nmol/L) for 2 h were added to the upper chambers and the wells were then incubated at 37 °C for 4 h. The lower chambers without stromal cells cultured were used as blank controls. The cell migration rate was counted as above. Stromal cells used in this study were prepared from lymph node lesions obtained from a patient with diffuse large B-cell lymphoma.
Statistical analysis All data are expressed as mean±SD, and analyzed using SPSS 11.5 for windows XP. Paired-samples t-test and independent-samples t-test was used for statistical analysis. P values of less than 0.05 or 0.01 were considered to be statistically significant.
Results
Effects of triptolide on the proliferation of Raji cells by MTT Raji cells treated with different concentrations of triptolide for 0, 24, 36, 48, 60, and 72 h resulted in the inhibition of cell proliferation, and the effects were dose- and time-dependent. The OD value of triptolide-treated groups decreased significantly compared with the untreated group. The results show great differences between the untreated group and the triptolide-treated groups (Figure 2). The IC50 value of 24 h is 43.06 nmol/L, and the IC50 value of 36 h is 25.08 nmol/L.
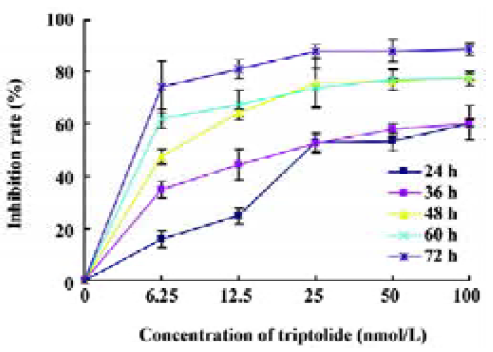
Effects of triptolide on the SDF-1α mRNA expression in lymph node stromal cells Extensive SDF-1α mRNA expression was detected in lymph node stromal cells from all 7 patients with different types of NHL, whereas it was only expressed weakly in the reactive hyperplasia lymph node stromal cells, the mRNA expression ratio for SDF-1α/β-actin was 1.22±0.30 and 0.25±0.15, respectively (P<0.01) (Figure 3). Lymph node stromal cells from patients with NHL were incubated with triptolide for 24 h at different concentrations. The densities of DNA bands weakened accordingly as the treatment concentration increased. The mRNA expression ratio for SDF-1α/β-actin were 1.15±0.33, 0.88±0.35, and 0.74±0.32 for stromal cells treated by 12.5, 25, 50 nmol/L triptolide, respectively. Compared with the untreated control cells, triptolide (12.5 nmol/L) reduced the expression of SDF-1α slightly, but the difference was not statistically significant (P>0.05), whereas triptolide (25, 50 nmol/L) sharply inhibited the SDF-1α expression and the inhibition was dose-related (P<0.01) (Figure 4).

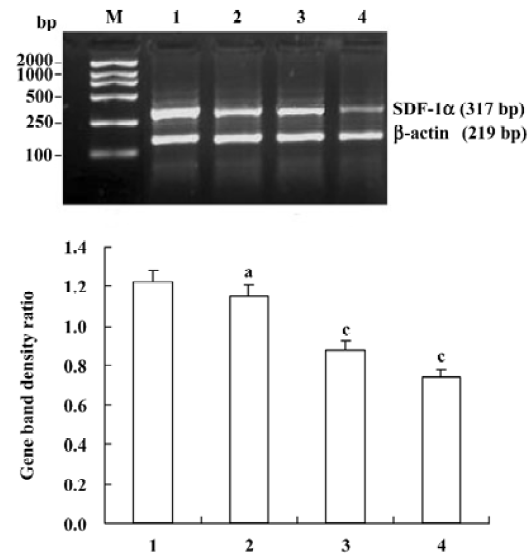
Effects of triptolide on the CXCR4 expression of lymphoma cells Flow cytometry was performed to determine the expression of CXCR4 on the surface of lymphoma cells freshly isolated from lymph node lesions of patients with NHL. The percentage of the lymphoma cell contained in the samples analyzed was more than 80% in all cases. In untreated cells, the average expression rate of CXCR4 was 17.37%±7.21%. After treatment with 12.5, 25, 50 nmol/L triptolide for 24 h, the CXCR4 expression rate of lymphoma cells were 11.13%±5.66%, 8.30%±4.61%, and 3.31%±2.35%, respectively, and the expression decreased in a dose-dependent manner in comparison with the control. These data suggest that triptolide could inhibit the expression of CXCR4 receptor on lymphoma cells (Figure 5).
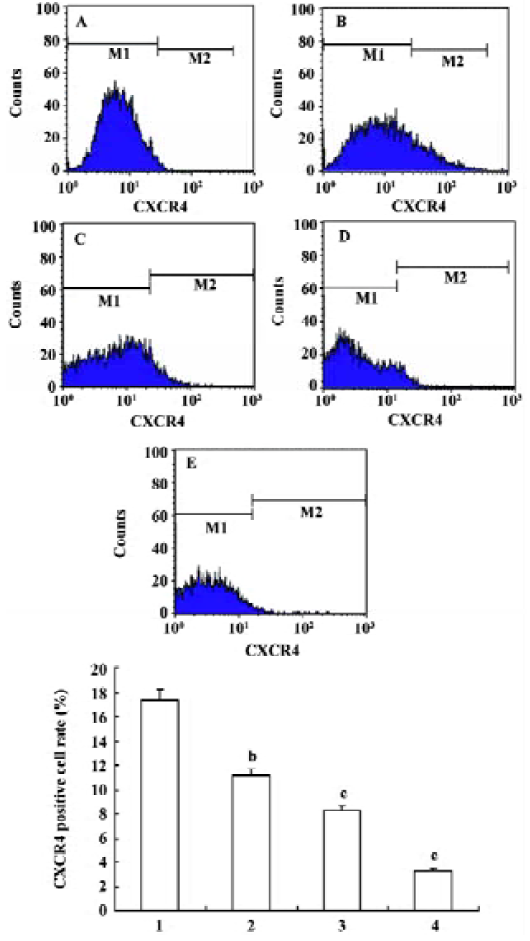
Effects of triptolide on the migration of lymphoma cells in vitro Chemotaxis assays showed that lymphoma cells isolated from the lymph node of patients with NHL in the upper chambers could not only migrate to the lower chambers contained rhSDF-1α, but also effectively migrate to the lower chambers seeded cultured lymph node stromal cells. After pretreatment with 0, 12.5, 25, 50 nmol/L triptolide for 2 h, when lower chambers contained rhSDF-1α, the cell migration rates were 75.4%±15.8%, 59.4%±17.3%, 30.3%±13.8%, and 21.4%±9.9%, respectively; whereas when lower chambers seeded the cultured stromal cells derived from the patient with diffuse large B-cell lymphoma, the cell migration rates were 61.1%±15.5%, 53.4%±17.3%, 20.8%±7.4%, and 15.4%±6.3%, respectively. Our results reveal that compared with the control groups, lymphoma cell migration towards either rhSDF-1α or the cultured lymph node stromal cells was markedly inhibited by the addition of triptolide in vitro, and the inhibitions were dose-dependent (Figure 6).
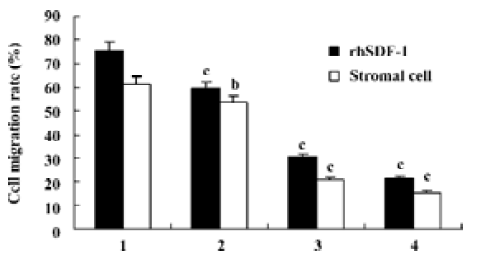
Discussion
The interest in exploiting traditional medicines for prevention or treatment of tumors is increasing. The Chinese herb TWHF has been used in traditional Chinese medicine for more than two thousand years. However, its potential value was recognized by Western medicine only after investigators observed the effectiveness of TWHF in the treatment of leprosy and rheumatoid arthritis. Triptolide is purified from TWHF, and has been identified as the major component of TWHF responsible for immunosuppressive and anti-inflammatory effects. It has been confirmed that triptolide is effective for the treatment of a variety of autoimmune diseases and for the prevention of allograft rejection and graft-versus-host disease, and it also possesses a male anti-fertility effect[1]. Currently, multiple studies in vitro and in vivo have shown that triptolide was cytotoxic to various types of tumor cells and significant anti-proliferative and apoptotic effects were observed in tumor cells treated by triptolide[14–17]. In the study of Yinjun et al[14], triptolide inhibited the proliferation of multiple myeloma cell lines (RPMI-8226 and U266) in a dose-dependent manner (10–80 ng/mL), and induced the apoptosis in MM cells through activation of the cystein protease caspase 8, 9 and 3, and subsequent cleavage of the DNA repair enzyme poly (ADP-ribose) polymerase. Moreover, triptolide downregulated nuclear factor (NF)-kappaB activity in MM cell lines, and also induced chemosensitivity to doxorubicin and suppressed cell proliferation of fresh MM cells. Lou et al[15] demonstrated that triptolide inhibited chronic myelogenous leukemia cell line K562 cell proliferation and induced apoptosis in a dose- and time-dependent manner. The growth-inhibitory IC50 value for triptolide treatment was 40 ng/mL. Obviously, triptolide-induced apoptosis of K562 cells was associated with a decline in the expression of bcr-abl, which was the hybrid gene of the Philadelphia chromosome t (9;22). Triptolide was able to decrease the expression of bcr-abl down to 50%, 30%, and 20% of the basal value at the concentrations of 20 ng/mL, 40 ng/mL, and 80 ng/mL, respectively, after 72 h. Another study by Chang et al[16] found that triptolide enhanced chemotherapy-induced apoptosis in fibrosarcoma cell line (HT1080) and NSCLC cell line (A549). Triptolide induced p53, but inhibited p21waf1/cip1 expression. They also found that triptolide induced accumulation of cells in S phase and blocked p21-mediated accumulation of cells in G2/M. In addition, it has been shown that triptolide inhibited the proliferation of glioma cells in vitro, and could also promote the expression of Bax and inhibit the expression of Bcl-2 and therefore accelerate cell apoptosis in glioma cell lines[17]. In this study, we found that triptolide could inhibit the proliferation of B-NHL cell line Raji cells in a dose- and time-dependent manner. The 24-h IC50 value was 43.06 nmol/L, and the 36-h IC50 value was 25.08 nmol/L. Therefore, it seems that triptolide is cytotoxic to tumor cells at a relatively low concentration. The mechanism of its antiproliferative effect and the activity to initiate apoptosis are related to downregulation of NF-kappaB, activation of caspase proteins, and regulation of the expression of several oncogenes and anti-oncogenes which are important regulator of apoptosis and cell cycle. Apart from this, it was found triptolide inhibited experimental metastasis of melanoma cell line B16F10 cells to the lungs and spleens of mice, which suggested that triptolide possessed the activity to inhibit tumor metastasis[12]. However, its mechanism has not been fully elucidated.
Cell migration along chemokine gradients involves a number of steps including the establishment of cell polarity, directional cell locomotion through cytoskeletal rearrange-ments, and adhesive interactions with extracellular matrix[18]. Metastasis, which is the major cause of morbidity and mortality in the majority of tumors, shares many similarities with normal circulating cells[5]. As mentioned above, SDF-1/CXCR4 axis is an important mechanism regulating tumor cell migration. Muller et al[6] were the first to provide direct evidence to support the notion that the SDF-1/CXCR4 axis could actually mediate human tumor metastasis in vivo. They reported that CXCR4 was highly expressed on human breast cancer cell lines, malignant breast tumors, and metastases, but not on normal breast tissue. Furthermore, SDF-1 mRNA exhibited peak levels of expression in lymph nodes, lung and bone marrow, where breast cancer cells metastasize preferen-tially. Signaling through CXCR4 in breast cancer cells mediated actin polymerization and pseudopodia formation, and subsequently induced chemotactic and invasive responses at the local level. Finally, in vivo neutralization with a specific monoclonal antibody against CXCR4 effectively inhibited the metastasis of breast cancer cells in an organ-specific manner. The research on NSCLC also found that CXCR4 was significantly expressed on tumor cells in freshly isolated tumor specimens from patients, and in vitro SDF-1 stimulation of CXCR4 on NSCLC cells could lead to chemo-taxis, calcium mobilization, and activation of mitogen-activated protein kinase p42/44 (extracellular signal-related kinase, ERK-1/2). Using a severe combined immunodeficiency (SCID) mouse system of heterotopic or orthotopic xenoengraftment of human NSCLC cells, they found that SDF-1 protein levels were significantly higher in organs that are known to be highly susceptible to human NSCLC metastases, as compared with either the primary tumor or plasma levels, suggesting that a chemotactic gradient could be established between the site of the primary tumor and those organs that develop NSCLC tumor metastases. Moreover, in this model system, it appeared that NSCLC cells expressing CXCR4 had a selective advantage for metastasizing to these organs. Furthermore, in vivo neutralization of SDF-1 in model system of spontaneous metastasis of human NSCLC resulted in marked attenuation of NSCLC metastases to several organs including the adrenal glands, liver, lung, brain, and bone marrow[7].
Similar studies regarding the role of SDF-1/CXCR4 axis were also investigated on malignant hematological diseases. It has been shown that CXCR4 was expressed on malignant B lymphocytes recovered from patients with different B-cell malignancies including acute lymphoblastic leukemia, chronic lymphocytic leukemia, hairy cell leukemia, mantle cell lymphoma, marginal zone B-cell lymphoma, small lymphocytic lymphoma, follicular cell lymphoma, and diffuse large B-cell lymphoma, and migration assays showed that SDF-1 was able to trigger migration of malignant B lymphocytes[12,19,20]. Moreover CXCR4 has been shown to be expressed in a subset of T-cell NHLs such as anaplastic large cell lymphoma, angioimmunoblastic T-cell lymphoma and peripheral T-cell lymphoma, which suggests that this chemokine receptor may have a role in the spread and metastasis of T-cell neoplasms as well[12,21]. Arai J et al[22] revealed that SDF-1 was expressed in the lymph node stromal cells present in lymphoma lesions, and most B-lymphoma cells expressed CXCR4 on their surface. CXCR4 positive primary B-lymphoma cells and B-lymphoma cell lines migrated towards rhSDF-1 and cultured stromal cells derived from the lymph node of patients with follicular or diffuse lymphoma, and these migrations were almost completely inhibited by the addition of anti-CXCR4 or anti-SDF-1 neutralizing antibody. Their data strongly suggested that the CXCR4 expressed on malignant B-lymphoma cells were functionally active, and that SDF-1/CXCR4 axis was the major chemokine system involved in the chemotactic interaction between B-lymphoma cells and lymph node stromal cells. Likewise, in a nonobese diabetes/severe combined immunodeficiency (NOD/SCID) mouse model of human high-grade NHL, CXCR4 neutralization by monoclonal antibodies had impressive in vitro effects on NHL cells including inhibition of transendo-thelial/stromal migration, enhanced apoptosis, decreased proliferation, and inhibition of pseudopodia formation[12]. In the present study, we examined the expression of SDF-1 and CXCR4 in lymph nodes of patients with NHL, and explored the possibility that metastatic lymphoma cells were responsive to stimulation of rhSDF-1 or primary lymph node stromal cells. It was found that SDF-1 transcript was strongly expressed in lymph node stromal cells from all the patients with different types of NHL, and CXCR4 was detected on primary lymphoma cells isolated from the lymph nodes of NHL patients. Chemotaxis assays revealed that primary lymphoma cells could effectively migrate towards to rhSDF-1 or cultured primary lymph node stromal cells. The results suggested that this chemokine ligand and receptor pair could mediate the metastasis of NHL cells. These results were consistent with that of other researchers[12,19–22]. Therefore, the SDF-1/CXCR4 axis might be a potential subject for therapeutic interventions in NHL.
Few data was available regarding the effect of triptolide on the lymph node metastasis in NHL in the published literature. In this report we reveal that, first, triptolide inhibited the expression of SDF-1α mRNA in lymph node stromal cells obtained from patients with NHL. Although the difference of SDF-1α expression between the group treated by triptolide (12.5 nmol/L) and the control was not significant, triptolide (25, 50 nmol/L) inhibited the SDF-1α expression and the inhibition was dose-related. Second, after a different dose of triptolide for 24 h, the expression of CXCR4 on the surface of primary lymphoma cells isolated from lymph nodes of patients with NHL decreased gradually in a dose-dependent manner. Third, chemotaxis assays showed that the migration of freshly isolated lymphoma cells towards either rhSDF-1 or cultured lymph node stromal cells was markedly inhibited by the addition of triptolide in vitro, and the inhibition was dose-dependent. Compared with the concentration to produce a marked effect on several other tumors as mentioned above, 12.5–50 nmol/L was relatively low, and might have low toxicities in renal, cardiac, hematopoietic and reproductive systems in human. In short, we concluded that triptolide was able to inhibit the migration of lymphoma cells via lymph nodes and the underlying mechanism might be related to the blockage of SDF-1/CXCR4 axis.
Furthermore, the SDF-1/CXCR4 axis has an effect on cell motility and directed chemotaxis, it also plays an important role in cell secretion. Interactions of CXCR4 with SDF-1 lead to secretion of some angiopoietic factors such as vascular endothelial growth factor (VEGF) in tumor cells[5]. These factors secreted by target cells are involved in tumor vasculo-genesis and in crosstalk between CXCR4 positive cells and endothelial[5]. In a previous study, we found that triptolide could suppress the VEGF expression on Raji cells[23]. Given the evidence that triptolide can not only block the SDF/CXCR4 axis, but also suppress the expression of VEGF in NHL, these mechanisms may be involved together in regulating tumor metastasis by triptolide in NHL. In conclusion, the inhibitory effect both on proliferation and tumor metastasis of triptolide showed that triptolide, purified by a traditional Chinese medicine, might be developed into a new anti-tumor drug in the treatment of NHL.
References
- Chen BJ. Triptolide, a novel immunosuppressive and anti-inflammatory agent purified from a Chinese herb Tripterygium wilfordii Hook F. Leuk Lymphoma 2001;42:253-65.
- Yang S, Chen J, Guo Z, Xu XM, Wang L, Pei XF, et al. Triptolide inhibits the growth and metastasis of solid tumors. Mol Cancer Ther 2003;2:65-72.
- Cyster JG. Chemokines and cell migration in secondary lymphoid organs. Science 1999;286:2098-102.
- Muller G, Hopken UE, Lipp M. Systemic immunoregulatory and pathogenic functions of homeostatic chemokine receptors. J Leukoc Biol 2002;72:1-8.
- Kucia M, Reca R, Miekus K, Wanzeck J, Wojakowski W, Janowska-Wieczorek A, et al. Trafficking of normal stem cells and metastasis of cancer stem cells involve similar mechanisms: pivotal role of the SDF-1-CXCR4 axis. Stem Cells 2005;23:879-94.
- Muller A, Homey B, Soto H, Ge N, Catron D, Buchanan ME, et al. Involvement of chemokine receptors in breast cancer metastasis. Nature 2001;410:50-6.
- Phillips RJ, Burdick MD, Lutz M, Belperio JA, Keane MP, Strieter RM. The stromal derived factor-1/CXCL12-CXC chemokine receptor 4 biological axis in non-small cell lung cancer metastases. Am J Respir Crit Care Med 2003;167:1676-86.
- Sun YX, Wang J, Shelburne CE, Lopatin DE, Chinnaiyan AM, Rubin MA, et al. Expression of CXCR4 and CXCL12 (SDF-1) in human prostate cancers (PCa) in vivo. J Cell Biochem 2003;89:462-73.
- Zhou Y, Larsen PH, Hao C, Yong VW. CXCR4 is a major chemo-kine receptor on glioma cells and mediates their survival. J Biol Chem 2002;277:49481-7.
- Barbero S, Bonavia R, Bajetto A, Porcile C, Pirani P, Ravetti JL, et al. Stromal cell derived factor 1α stimulates human glioblastoma cell growth through the activation of both extracellular signal-regulated kinase 1/2 and Akt. Cancer Res 2003;63:1969-74.
- Beutler E, Lichtman MA, Coller BS, Kipps TJ, Seligsohn U. Williams Hematology. 4th ed. New York (NY): McGraw-Hill Profession-al; 2000. p1049–57.
- Bertolini F, Dell’Agnola C, Mancuso P, Rabascio C, Burlini A, Monestiroli S, et al. CXCR4 neutralization, a novel therapeutic approach for non-Hodgkin’s lymphoma. Cancer Res 2002;62:3106-12.
- Harris NL, Jaffe ES, Stein H, Banks PM, Chan JK, Cleary ML, et al. A revised European-American classification of lymphoid neoplasms: a proposal from the International Lymphoma Study Group. Blood 1994;84:1361-92.
- Yinjun L, Jie J, Yungui W. Triptolide inhibits transcription factor NF-kappaB and induces apoptosis of multiple myeloma cells. Leuk Res 2005;29:99-105.
- Lou YJ, Jin J. Triptolide down-regulates bcr-abl expression and induces apoptosis in chronic myelogenous leukemia cells. Leuk Lymphoma 2004;45:373-6.
- Chang WT, Kang JJ, Lee KY, Wei K, Anderson E, Gotmare S, et al. Triptolide and chemotherapy cooperate in tumor cell apoptosis. A role for the p53 pathway. J Biol Chem 2001;276:2221-7.
- Zhou YX, Huang YL, Xu QN, Ye M, Sun CF, Zhou D. Several monomes from Tripterygium wilfordii inhibit proliferation of glioma cells in vitro. Ai Zheng 2002;21:1106-8.
- Sanchez-Madrid F, del Pozo MA. Leukocyte polarization in cell migration and immune reactions. EMBO J 1999;18:501-11.
- Trentin L, Cabrelle A, Facco M, Carollo D, Miorin M, Tosoni A, et al. Homeostatic chemokines drive migration of malignant B cells in patients with non-Hodgkin lymphomas. Blood 2004;104:502-8.
- Bradstock KF, Makrynikola V, Bianchi A, Shen W, Hewson J, Gottlieb DJ. Effects of the chemokine stromal cell-derived factor-1 on the migration and localization of precursor-B acute lymphoblastic leukemia cells within bone marrow stromal layers. Leukemia 2000;14:882-8.
- Weng AP, Shahsafaei A, Dorfman DM. CXCR4/CD184 immunoreactivity in T-cell non-Hodgkin lymphomas with an overall Th– Th2+ immunophenotype. Am J Clin Pathol 2003;119:424-30.
- Arai J, Yasukawa M, Yakushijin Y, Miyazaki T, Fujita S. Stromal cells in lymph nodes attract B-lymphoma cells via production of stromal cell-derived factor-1. Eur J Haematol 2000;64:323-32.
- Liu F, Cui GH, Zhang C, Wang Y, Chen Y. Effects of triptolide on the expression of vascular endothelial growth factor (VEGF) in cultured Raji cells. Chin Hosp Pharm J 2004;24:413-5.