Soybean isoflavones alter parvalbumin in hippocampus of mid-aged normal female, ovariectomized female, and normal male rats1
Introduction
Phytoestrogens are very important in human and animal nutrition[1]. Isoflavones are abundant in soybeans, and they are present in appreciable concentrations in a variety of beans, spouts and legumes[2,3]. The major and most relevant property of soybean isoflavones is their modest agonist effect on the β estrogen receptor (approximately one-third as potent as estradiol) and their weak effect on the α estrogen receptor (with 0.001 of the potency of estradiol)[4]. Genistein is also a potent inhibitor of protein tyrosine kinase[5–7]. Protein tyrosine kinase is known to play a key role in growth factor-related signal modulation and in programmed cell death, known as apoptosis[7]. In cells exposed to genistein, the number of cells undergoing apoptosis increase in vitro and in vivo[7,8]. In aortic artery and atrial fibers, genistein decreases the contractile response and arterial blood pressure[9,10].
In postmenopausal women, estrogen therapy significantly lowers the risk of Alzheimer disease. Estrogens also alter certain specific pathologies such as the epileptic seizure threshold and Parkinson’s disease[11–13].
Recently, it has been reported that there is a cross-talk between steroid hormones and neurotransmitters. Estrogen induces structural and functional changes in excitatory input to hippocampal CA1 pyramidal cells in adult female rats. Estrogen increases the density of dendritic spines and spine synapses on CA1 pyramidal cells[14–16] concomitant with an increase in peak Ca2+ levels, an effect that was blocked by an N-methyl-D-aspartate receptor antagonist. The estrogen-modulated signaling would presumably lead to an increase in downstream calcium-dependent responses[17]. However, the modulation of intracellular calcium is very important in long-term treatment with estrogen or isoflavones because a sustained increase in calcium may induce neuronal death. A calcium-binding protein, parvalbumin (PV), participates in the regulation of calcium homeostasis and acts as an intra-cellular calcium buffer that regulates the concentration of the ion during neuronal activity[18]. In the present study, therefore, we investigated the long-term effects of soybean isoflavones on PV immunoreactivity in the hippocampus in normal female, ovariectomized (OVX) female, and normal male rats.
Materials and methods
Extraction of soybean isoflavones Isoflavones were extracted from soybean hypocotyls with 10 volumes of 80% aqueous methanol by stirring for 4 h at room temperature. The methanol extract was condensed in a rotary evaporator at 50 °C, and the soybean isoflavone extract was obtained by freeze-drying the concentrated methanol extract. A high performance liquid chromatography (HPLC) analysis of 3 isoflavones and their 9 derivatives was performed using a diode array detector (HP1100 system, Agilent, Palo Alto, CA,USA) and an eclipse XDB C-18 column (Agilent). Ultraviolet detection was performed at a wavelength of 260 nm, and the injection volume was 5 µL. The mobile phases used for analysis were 0.1% acetic acid in H2O (solvent A) and 0.1% acetic acid in acetonitrile (solvent B). A flow rate of 1.2 mL/min under an initial condition of 93:7 (A:B) was held for 25 min, then brought to 15% B in 25 min, to 20% B in 5 min, and to 25% B in 15 min, all with a linear gradient. Genistein, daidzein, and glycitein were purchased from Sigma (St Louis, MO, USA), and the isoflavone derivatives (the malonyl-, acetyl-, and glycoside forms) were purchased from Fujico (Tokyo, Japan) for use as standards in the HPLC analyses[19].
Experimental animals The present study used the progeny of male and female Sprague-Dawley rats (3–4 months old) purchased from Bio-Genomics (Seoul, South Korea). The animals were housed at constant temperature (23 ºC) and relative humidity (60%) with a fixed 12 h light/dark cycle and free access to food and water. Procedures involving animals and their care conformed to guidelines, which are in compliance with current international laws and policies (NIH Guide for the Care and Use of Laboratory Animals, NIH Publication N
When the rats were 10 months old, they were assigned to one of 9 groups (n=7 in each group) based on body weight using a randomized complete-block design. The groups were: control diet-treated normal females, OVX females, and males; 0.3 g/kg soy isoflavone-treated normal females, OVX females, and males; and 1.2 g/kg soy isoflavone-treated females, OVX females, and males. The rats’ isoenergetic and isonitrogenous diets were based on the AIN76A formulation and the isoflavone extract was blended with the basal diet at the expense of fiber. These experimental diets were fed to the rats for 16 weeks. Distilled water was always available to the rats.
Tissue processing and immunohistochemistry All the animals were anesthetized with pentobarbital sodium, and perfused transcardially with 0.1 mol/L phosphate-buffered saline (PBS, pH 7.4) followed by 4% paraformaldehyde in 0.1 mol/L PBS (pH 7.4). The rats’ brains were removed, and postfixed in the same fixative for 6 h. Brain tissues were cryoprotected by infiltration with 30% sucrose overnight. Thereafter the brain tissues were frozen and sectioned with a cryostat at 30 μm and consecutive sections were collected in 6-well plates containing PBS.
To ensure that immunohistochemistry data were comparable between groups, free-floating sections of the brains of experimental animals from each group were processed for immunohistochemical analysis simultaneously. The sections were sequentially treated with 0.3% hydrogen peroxide (H2O2) in PBS for 30 min and 10% normal horse serum in 0.05 mol/L PBS for 30 min. The sections were next incubated with diluted mouse anti-PV (Sigma, USA; 1:1000) overnight at room temperature. Thereafter the tissues were exposed to biotinyl-ated goat anti-mouse IgG and streptavidin peroxidase complex (Vector, USA). The sections were visualized with 3,3'-diaminobenzidine in 0.1 mol/L Tris buffer and mounted on gelatin-coated slides[20,21].
Quantification of data and statistical analysis All measurements were performed in order to ensure objectivity, by 2 observers for each experiment, who were blinded to the treatments received by the rats, carrying out the measurements for the control and experimental sections simultane-ously.
At magnifications of 25–50, the mid-point areas of the hippocampal CA1 region were measured. Images of all PV-immunoreactive structures taken from the 3 layers (striata oriens, pyramidale and radiatum in the hippocampus proper, and molecular, granule cell and polymorphic layers in the dentate gyrus) were obtained through an Axiophot light microscope (Carl Zeiss, Germany) connected via a Charge Coupled Device (CCD) camera to a computer monitor. Video images were digitized into an array of 512×512 pixels corresponding to a tissue area of 140 µm×140 µm (40×primary magnification). To evaluate the PV-immunoreactive fibers and neurons, the PV-immunolabeled fibers or neurons were selected by using the ImageJ software (RSB, Bethesda, Maryland, USA) by interactively determining each cell limit.
The resolution of each pixel was 256 gray levels. The intensity of PV immunoreactivity was evaluated by means of a relative optical density (ROD) value. ROD was obtained after transformation of mean gray values into ROD using the formula: ROD=log(256/mean gray value). The values of background staining were obtained and subtracted from the ROD values of all immunoreactive structures before statistically processing the obtained values. ROD values were presented as ROD units.
The number of PV-immunoreactive neurons was calculated by using an image analyzing software system (Optimas 6.5, CyberMetrics, USA). The mid-point of the hippocampal CA1 region (500 µm2) and the hilar region of the dentate gyrus were selected by the image analyzing system. Cell counts were obtained by averaging the counts from 30 sections taken at the same level of the hippocampal CA region and dentate gyrus.
Four to 6 separate immunohistochemical experiments were run for each primary antibody. Each individual experiment was composed of 6–10 tissue sections of each animal from each group. Five to ten fields were measured for each brain area in each section of each animal. Inter-animal differences in each group, as well as interexperiment differences, were not statistically significant. The values shown here represent the means of experiments performed for each hippocampal area. Differences among the means were statistically analyzed by one-way analysis of variance followed by Duncan’s new multiple range method or the Newman-Keuls test to elucidate the effect of isoflavones in PV immunoreactivity or to investigate the differences with respect to PV immunoreactivity in different sexes. P<0.05 was considered statistically significant.
Results
Isoflavone concentrations in the diets A yield of 5018.2 mg isoflavone/100 g isoflavone extract was obtained (Table 1). The isoflavone concentrations of the 0.3 g/kg isoflavone-treated group and 1.2 g/kg isoflavone diets were 0.321 g/kg diet and 1.284 g/kg, respectively. Glycoside and malonyl forms were dominant in the extract. The daily soy isoflavone intake in the 0.3 g/kg isoflavone-treated group was 10.89 mg/kg body weight per day, and that in the 1.2 g/kg isoflavone group was 38.53 mg/kg body weight per day.
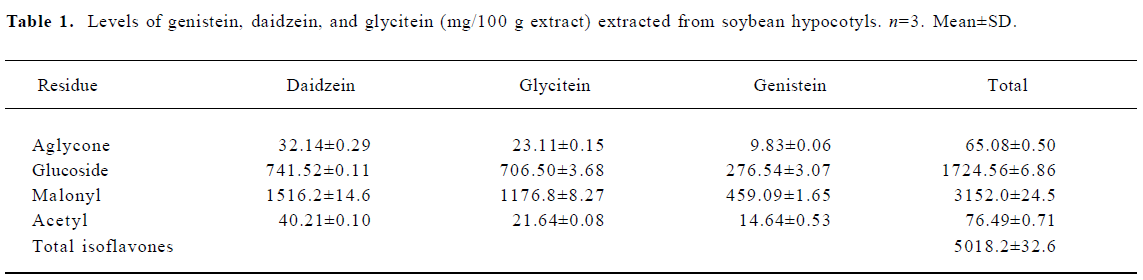
Full table
Food consumption and body weight Food consumption and body weight did not differ among the groups (data not shown).
Changes in PV immunoreactivity PV immunoreactivity was significantly changed in the hippocampal CA1 region and in the dentate gyrus, but not in the hippocampal CA2/3 region in any of the experimental groups after long-term treatment with isoflavones.
In the CA1 region, PV immunoreactivity in the control diet females was detected in non-pyramidal cells located near the striatum pyramidale (Figure 1A). PV immunoreactivity in the control diet OVX females and control diet males was higher than that in the control diet females (Figure 1B, 1C). PV immunoreactivity in the control diet OVX females was similar to that in the control diet males. PV immunoreactivity in all the 0.3 g/kg isoflavone-treated groups was decreased compared with all the control diet-treated groups (Figure 1D,1F, 2). PV-immunoreactive neuronal processes in these groups were poor compared with those of all the control diet-treated groups. PV immunoreactivity in all the 1.2 g/kg isoflavone-treated groups was decreased compared with all the 0.3 g/kg isoflavone-treated groups (Figure 1G,1H, 2). In particular, PV immunoreactivity in the 1.2 g/kg isoflavone-treated males was negligible (Figure 1I).
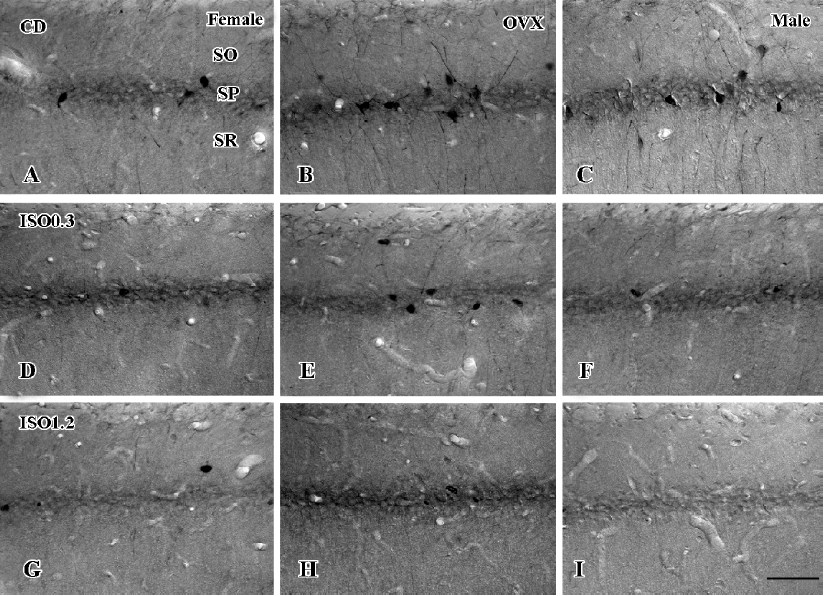
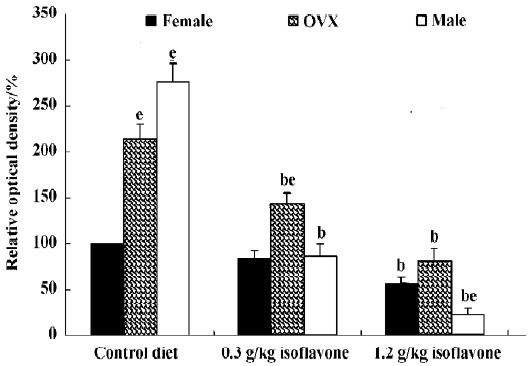
In the dentate gyrus, PV-immunoreactive neurons in the control diet females were distributed in the polymorphic layer (Figure 3A). These PV-immunoreactive neurons were non-granule cells and generally located in the subgranular zone in the polymorphic layer. Some PV-immunoreactive neurons were detected at the border between the granule cell layer and molecular layer. PV immunoreactivity in the control diet OVX females was similar to that of the control diet males, and was greater than that in the control diet females (Figure 3B, 4). PV immunoreactivity was decreased in all the 0.3 g/kg isoflavone-treated groups compared with rats in all the control diet-treated groups (Figure 3D–F, 4). In all the 1.2 g/kg isoflavone-treated groups, PV immunoreactivity was decreased compared with rats in all the 0.3 g/kg isoflavone-treated groups (Figure 3G–I, 4).
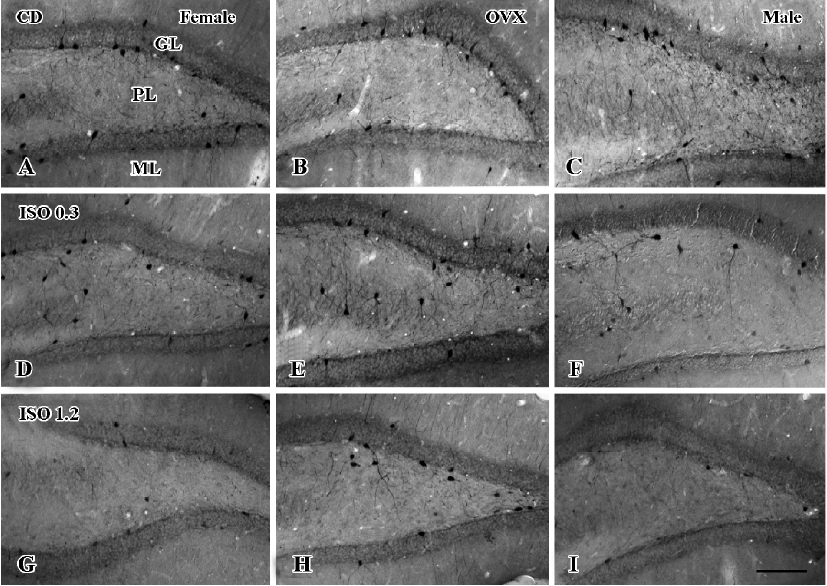
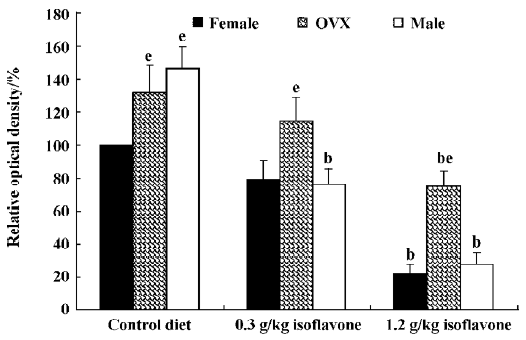
Changes in the number of PV-immunoreactive neurons In the hippocampal CA1 region, the number of PV-immunoreactive neurons in the control diet females was approximately 2.6 in a 500 µm diameter region of the CA1 (Figure 5). The number in the control diet OVX females was significantly increased compared with that of the control diet females, and similar to that for the control diet males (Figure 5). The number of PV-immunoreactive neurons in all the isoflavone-treated groups decreased dose-dependently. In the 1.2 g/kg isoflavone-treated males, there were negligible numbers of PV-immunoreactive neurons in the CA1 region.
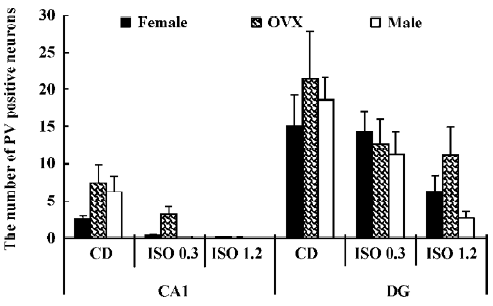
In the dentate gyrus, the number of PV-immunoreactive neurons in the control diet females was approximately 15 in a 500 µm diameter region of the dentate gyrus (Figure 5). The number of PV-immunoreactive neurons in the control diet OVX females and males was greater than that in the control diet females (Figure 5). This finding is similar to that in the hippocampal CA1 region. The number of PV-immunoreactive neurons in all the isoflavone-treated groups decreased dose-dependently.
Discussion
It has been reported that a reduction in PV immunoreactivity occured in the hippocampal CA1, CA3 and dentate gyrus following environmental enrichment. And the enriched housing animal also represents the also represents the positive effect on behavior and learning[22]. Because PV-expressing chandelier cells play an important role in controlling pyramidal cell excitability[23], the reduced expression of PV in the hippocampal CA1 region may be associated with protection against memory deficits during the aging process. This result coincides with that of our previous study, in which we found that soy isoflavones could ameliorate deficits in memory tasks resulting from the loss of cholinergic input to the hippocampus or cholinergic degeneration in elderly male rats[19].
In the present study, the formulation of the isoflavone diet was based on that used in previous studies[24,25]. Pan et al defined a dose suitable for a woman and added certain amounts of estradiol or soy isoflavones to the experimental diet[24]. The soy isoflavone content of the 0.3 g/kg isoflavone-treated group was determined by considering the caloric density of the diet, and making the dose equivalent to that for a woman. Lund et al investigated the neurobehavioral effects of isoflavones on rats[25]; they used a diet containing soy isoflavones (0.6 g/kg diet). This diet produced an improvement in cognitive function in female rats, but tests with higher concentrations of soy isoflavones were not performed. In the present study, we tried to evaluate the effects of very large amounts of isoflavone on the brains of female rats.
In the present study, we investigated changes in PV immunoreactivity in the hippocampus of normal female, ovariectomized female and normal male rats after long-term treatment with soybean isoflavones. PV immunoreactivity was found in non-pyramidal cells in the hippocampus proper and in polymorphic cells in the dentate gyrus. PV immunoreactivity and immunoreactive neurons in the control diet OVX females were more pronounced than in the control diet females. PV immunoreactivity and the number of immunoreactive neurons in the control diet OVX females were similar to those in the control diet males. In all the isoflavone-treated groups, PV immunoreactivity and the number of immunoreactive neurons decreased dose-dependently after isoflavone treatment. This result shows that ovariectomy may increase PV immunoreactivity in the hippocampus.
Choi and Lee investigated the cytotoxic effects of genistein in vivo, and found that a high intake of genistein (20 mg/day) induced cytotoxicity in rat brain, but that a lower intake (2 mg/day) produced no such effect[26]. The daily soy isoflavone intake in the 1.2 g/kg isoflavone-treated groups was 29.74 mg. However, the proportion of the soy isoflavones in the diet represented by genistein was 15%, and the daily genistein intake was only 4.461 mg/day. This amount is much lower than the level of genistein that was found to produce cytotoxic effects on rat brain[26]. Therefore, the dose of soy isoflavones used in the present study produces no cytotoxic or apoptotic effects in rat brain.
It has been reported that estrogen modulates neuronal excitability either by affecting the presynaptic release of neurotransmitters or by direct actions on the postsynaptic membrane[27]. Estrogen transiently disinhibits hippocampal CA1 pyramidal cells in adult female rats and prolongs the decay time of inhibitory postsynaptic currents in these cells, thus excitatory input to CA1 pyramidal cells is enhanced, dendritic spine and synapse density are increased[28], and spatial working memory is improved[29,30].
In neurons pretreated with isoflavones, the excitotoxic glutamate-induced rise in intracellular Ca2+ concentration is decreased to non-toxic levels[31]. In the present study, the decrease of PV immunoreactivity in the hippocampus may be associated with the sustained decrease of calcium in this region. The sustained decrease of calcium may induce the downregulation of PV in this region. The attenuation of the excitotoxic glutamate-induced rise in intracellular calcium to near the non-toxic range provides a probable mechanism by which isoflavones reduce glutamate cytotoxicity and promote neuronal survival.
In aging OVX rats, long-term hormone replacement with oestrogen or oestrogen plus progesterone produces substantial reductions in the levels of choline acetyltransferase (ChAT) and tyrosine receptor kinase A (trkA) mRNA in the medial septum and nucleus basalis magnocellularis, relative to much younger OVX controls[32]. However, the number and size of the cholinergic neurons were not significantly altered. This result suggests that long-term hormone replacement had no apparent effect on the number of ChAT-positive neurons, and did not prevent the reduction in ChAT and trkA mRNA associated with ovariectomy and aging[32].
In conclusion, long-term administration of soybean isoflavones induces the reduction of PV immunoreactivity in the hippocampus, and the reduction of PV in this region suggests that the long-term administration of isoflavones may be related to calcium homeostasis in the hippocampal CA1 region and in the hilar region of the dentate gyrus, indicating the neuronal plasticity of these neurons.
Acknowledgements
The authors would like to thank Mr Suek HAN, Seung-uk LEE and Ms Hyun-sook KIM for their technical help in this study.
References
- Ward WE, Thompson LU. Dietary estrogens of plant and fungal origin: occurrence and exposure. In: Metzler M, editor. The handbook of environmental chemistry; volume L, part I; Endocrine disruptors. Berlin: Springer; 2001. p 101–28.
- Csaky I, Fekete S. Soybean: feed quality and safety. Part 1: biologically active components. A review. Acta Vet Hung 2004;52:299-313.
- Valachovicova T, Slivova V, Sliva D. Cellular and physiological effects of soy flavonoids. Mini Rev Med Chem 2004;4:881-7.
- Kuiper GG, Lemmen JG, Carlsson B, Corton JC, Safe SH, van der Saag PT, et al. Interaction of estrogenic chemicals and phytoestrogens with estrogen receptor beta. Endocrinology 1998;139:4252-63.
- Akiyama T, Ishida J, Nakagawa S, Ogawara H, Watanabe S, Itoh N, et al. Genistein, a specific inhibitor of tyrosine-specific protein kinases. J Biol Chem 1987;262:5592-5.
- Feller SM, Wong TW. Identification and characterization of a cytosolic protein tyrosine kinase of HeLa cells. Biochemistry 1992;31:3044-51.
- Peterson G. Evaluation of the biochemical targets of genistein in tumor cells. J Nutr 1995;125:784s-9s.
- Ji ES, Yue H, Wu YM, He RR. Effects of phytoestrogen genistein on myocardial ischemia/reperfusion injury and apoptosis in rabbits. Acta Pharmacol Sin 2004;25:306-12.
- Li HF, Wang LD, Qu SY. Phytoestrogen genistein decreases contractile response of aortic artery in vitro and arterial blood pressure in vivo. Acta Pharmacol Sin 2004;25:313-8.
- Ma T, Liu S, Zhang WL, Zhao H, Yu SY, He RR. Effects of phytoestrogen genistein on electrophysiology of human atrial fibers. Acta Pharmacol Sin 2002;23:851-4.
- Buterbaugh GG, Hudson GM. Estradiol replacement to female rats facilitates dorsal hippocampal but not ventral hippocampal kindled seizure acquisition. Exp Neurol 1991;111:55-64.
- Henderson VW, Watt L, Buckwalter JG. Cognitive skills associated with estrogen replacement in women with Alzheimer’s disease. Psychoneuroendocrinology 1996;21:421-30.
- Leranth C, Roth RH, Elswoth JD, Naftolin F, Horvath TL, Redmond DE Jr. Estrogen is essential for maintaining nigrostriatal dopamine neurons in primates: implications for Parkinson’s disease and memory. J Neurosci 2000;20:8604-9.
- Gould E, Woolley CS, Frankfurt M, McEwen BS. Gonadal steroids regulate dendritic spine density in hippocampal pyramidal cells in adult hood. J Neurosci 1990;10:1286-91.
- McEwen BS, Tanapat P, Weiland NG. Inhibition of dendritic spine induction on hippocampal CA1 pyramidal neurons by a nonsteroidal estrogen antagonist in female rats. Endocrinology 1999;140:1044-7.
- Woolley CS, McEwen BS. Roles of estradiol and progesterone in regulation of hippocampal dendritic spine density during the estrous cycle in the rat. J Comp Neurol 1993;336:293-306.
- Rajadhyaksha A, Leveque J, Macias W, Barczak A, Konradi C. Molecular components of striatal plasticity: the various routes of cyclic AMP pathways. Dev Neurosci 1998;20:204-15.
- Kawaguchi Y, Katsumaru H, Kosaka T, Heizman CW, Hama K. Fast spiking cells in rat hippocampus (CA1 region) contain the calcium-binding protein parvalbumin. Brain Res 1987;416:369-74.
- Lee YB, Lee HJ, Won MH, Hwang IK, Kang TC, Lee JY, et al. Soy isoflavones improve spatial delayed matching-to-place performance and reduce cholinergic neuron loss in elderly male rats. J Nutr 2004;134:1827-31.
- Hwang IK, Eum WS, Yoo KY, Cho JH, Kim DW, Choi SH, et al. Copper chaperone for Cu,Zn-SOD supplement potentiates the Cu,Zn-SOD function of neuroprotective effects against ischemic neuronal damage in the gerbil hippocampus. Free Radic Biol Med 2005;39:392-402.
- Hwang IK, Yoo KY, Kim DS, Jung JY, Kim KS, Park JK, et al. Changes in parvalbumin immunoreactivity in the parietofrontal cortex after transient forebrain ischemia in the Mongolian gerbil. Mol Cells 2004;17:304-8.
- Iuvone L, Geloso MX, Dell’Anna E. Changes in open field behavior, spatial memory, and hippocampal parvalbumin immunoreactivity following enrichment in rats exposed to neonatal anoxia. Exp Neurol 1996;139:25-33.
- DeFelipe J. Types of neurons, synaptic connections and chemical characteristics of cells immunoreactive for calbindin-D28K, parvalbumin and calretinin in the neocortex. J Chem Neuroanat 1997;14:1-19.
- Pan Y, Anthony M, Clarkson TB. Effect of estradiol and soy phytoestrogens on choline acetyltransferase and nerve growth factor mRNAs in the frontal cortex and hippocampus of female rats. Proc Soc Exp Biol Med 1999;221:118-25.
- Lund TD, West TW, Tian LY, Bu LH, Simmons DL, Setchell KD, et al. Visual spatial memory is enhanced in female rats (but inhibited in males) by dietary soy phytoestrogens. BMC Neurosci 2001;2:20-32.
- Choi EJ, Lee BH. Evidence for genistein mediated cytotoxicity and apoptosis in rat brain. Life Sci 2004;75:499-509.
- Wooley CS. Electrophysiological and cellular effects of estrogen on neuronal function. Crit Rev Neurobiol 1990;13:1-20.
- Woolley CS, McEwen BS. Estradiol mediates fluctuation in hippocampal synapse density during the estrous cycle in the adult rat. J Neurosci 1992;12:2549-54.
- Daniel JM, Dohanich GP. Acetylcholine mediates the estrogen-induced increase in NMDA receptor binding in CA1 of the hippocampus and the associated improvement in working memory. J Neurosci 2001;21:6949-56.
- Sandstrom NJ, Williams CL. Memory retention is modulated by acute estradiol and progesterone replacement. Behav Neurosci 2001;115:384-93.
- Zhao L, Chen S, Brinton RD. An estrogen replacement therapy containing nine synthetic plant-based conjugated estrogens promotes neuronal survival. Exp Biol Med 2003;228:823-35.
- Gibbs RB. Effects of ageing and long-term hormone replacement on cholinergic neurones in the medial septum and nucleus basalis magnocellularis of ovariectomized rats. J Neuroendocrinol 2003;15:477-85.