Changes of 5-lipoxygenase pathway and proinflammatory mediators in cerebral cortex and lung tissue of sensitized rats1
Introduction
The interaction between central nervous-immune pathways or neuroendocrine-immune (NEI) networks in various disease states is affected by various pathophysiologic status. In the pathogenesis of allergic diseases, the abnormalities in immune function are mediated by the NEI network through overproduction of allergic mediators[1]. Undoubtedly, leukotrienes (LTs), the metabolites of 5-lipoxygenase (5-LO) pathways are thought to play crucial roles in the inflammatory network. Up to now, it is unclear how the metabolites of 5-LO pathways in the central nervous system regulate inflammation in lung tissue of asthma rats. From previous work, we found that the change of Th1/Th2 paradigm (ratio of interferon (IFN)-γ/interleukin (IL)-4 decreased and the content of LTB4 in the cerebral cortex increased correspondent to that in bronchoalveolar lavage fluid (BALF) or lung tissue in inflammatory status of asthma rats[2,3]. These findings indicate that there is an interactive response of LTs and proinflammatory cytokines in the central nervous system, and the changes of these proinflammatory mediators in the central nervous system may have pathophysiologic effects in asthma rats. To explore these hypotheses and get a clearer understanding of the central nervous system changes of 5-LO pathway, Tumor necrosis factor (TNF)-α, IL-4, IFN-γ, and nitric oxide (NO) in asthma rats, we have further investigated the correlative alterations of proinflammatory mediators and the expression of 5-LO pathway enzyme in lung tissue and cerebral cortex.
Materials and methods
Animal and reagent Sprague–Dawley rats of either sex weighing 180 g±20 g were purchased from the Laboratory Animal Center of Medical School of Zhejiang University (Grade II, Certificate N
Sensitization, treatment, and challenge regimens The sensitization procedure to induce IgE-mediated asthma response is as earlier described[3]. At d 14 after sensitization, the rats were placed in a 45 cm×45 cm×15 cm plastic box and challenged by exposure to an aerosol of ovalbumin (10 g/L in saline) which was generated in a jet nebulizer (partical size 1-5 µm; BARI, MASTER, Germany) for 20 min, and repeated daily for 6 d. Control rats were similarly exposed to an aerosol of saline. One hour before antigen challenge, the rats in the treatment groups were administered with DXM (0.5 mg/kg, ip) and ketotifen (5 mg/kg, ig), respectively. The rats in the control and model groups were administered with saline (ip). All the animals were studied on d 21 after the first sensitization.
BALF Bronchoalveolar lavage (BAL) was performed by flushing the airways with saline 5 mL containing 1% bovine serum albumin and 1 kU/L heparin sodium through the tracheal cannula three times. BAL fluid (BALF) were pooled and centrifuged (Eppendorf Centrifuge 5804R, Germany) at 500×g for 10 min at 4 ℃. The supernatant was collected and stored at -70 ℃ for assaying IFN-γ, TNF-α, NO, and IL-4 level.
Brain homogenates preparation Blood cells in systemic circulation were removed by perfused with D-Hanks’ liquid through ascending aorta cannula. The left cerebral cortex was then cut into 1 mm3, homogenated (DY89-I Homogenater, Linbo Xinzhi SCI-TETH research Institute, Ningbo) in ice-cold Hanks’ buffer (pH 7.5). Thereafter, the homogenates were centrifuged at 3000×g at 4 ℃ for 10 min. The supernatant was collected and stored at -70 ℃ for assaying IFN-γ, TNF-α, NO, and IL-4 level.
Assay of IFN-γ, TNF-α, NO, and IL-4 The IFN-γ, TNF-α, NO, and IL-4 levels in the BALF and cerebral cortex homogenates were measured according to the ELISA kit description. All measurements were carried out in duplicate.
Reverse transcription-polymerase chain reaction (RT-PCR) assay Total RNA was isolated from the cerebral cortex and lung tissue. Trizol 1 mL was added per 100 mg tissue and was homogenized; samples were left at 18–21 ℃ for 3 min. Chloroform 0.2 mL was added to the homogenizer and mixed. After 10 min at 18–21 ℃, the samples were centrifuged at 15 000×g in a microcentrifuge at 4 ℃ for 15 min. The upper phase was transferred to new tubes and the same volume isopropanol was added. RNA was allowed to precipitate at 18–21 ℃ for 10 min and collected by centrifugation at 15 000×g at 4 ℃ for 10 min. After washing precipitates in 75% ethanol and drying for 10 min, the RNA was dissolved in DEPC-treated water and quantified by the measurement of ultraviolet absorption at 260 nm (Eppendorf Biophotometer, Germany). All samples were kept at -70 ℃ until use.
For the synthesis of the single-stranded cDNA (Eppendorf Mastercycler gradient, Germany), total RNA (4 μg) from each sample was reverse-transcripted using an antisense specific primer and 200 U of SuperScript II RT. Sequences of the PCR primers for 5-LO, LTA4-H, and glyceraldehydes-3-phosphate dehydrogenase (GAPDH) were derived from published sequences as follows: 5-LO: sense 5´ AGG CTG CAG TGA GAA GCA TC 3´, antisense 5´ GCC AGT GGT TCT TGA CTC TC 3´, designed to amplify a fragment corresponding to nucleotides 181–770[4]; LTA4-H: sense 5´CAG TCA CAG GAG GAT AAT 3´, antisense 5´ GGA GTG AGC CAC TGA AGG 3´, designed to amplify a fragment corresponding to nucleotides 131–363[5]; GAPDH: sense 5´ ACC ACC ATG GAG AAG GCT GG 3´, antisense 5´ CTC AGT GTA GCC CAG GAT GC 3´, designed to amplify a fragment corresponding to nucleotides 372–899. All amplified segments extended over at least 1 intron boundary of the genomic DNA. The reactants were cycled at 94 ℃ 30 s, 59 ℃ 20 s, 72 ℃ 10 s for LTA4-H, and 94 ℃ 45 s, 58 ℃ 30 s, 72 ℃ 90 s for 5-LO and GAPDH. Reaction products were separated by electrophoresis using 1.5% standard agarose gel. Amplification of GAPDH was performed as a control. PCR products were analyzed by electrophoresis (CLP, USA), visualized by ultraviolet transillumination of ethidium bromide-stained gels, and the intensity of each band was measured by the UVP Image Analyzer (UVP, Inc. Upland, CA). The expression of 5-LO and LTA4-H were normalized to that of GAPDH.
5-LO Western immunoblotting The cerebral cortex and lung tissue were dissected and homogenized in homogenizing buffer, containing Tris-HCl 20 mmol/L, egtazic acid 2 mmol/L, edetic acid 5 mmol/L, pepstatin 1.5 mmol/L, leupeptin 2 mmol/L, phenylmethylsulfonyl fluoride 0.5 mmol/L, aprotinin 0.2 kU/L, and dithiothreitol 2 mmol/L, using a Polytron. The homogenates were centrifuged at 100 000×g at 4 ℃ for 60 min . The resulting supernatant was a portion of the cytosol (S1) fraction, and the pellet was resuspended in the homogenizing buffer and centrifuged again at 100 000×g at 4 ℃ for 60 min. The resulting supernatant (S2) was combined with the S1 fraction, and the combination was used for analyses as the “cytosol” fraction. The concentration of protein in these two fractions was determined using the procedure of Lowry et al.
Equal volumes of protein samples (40 mg of protein) and gel loading solution (Tris-HCl 50 mmol/L pH 6.8, β-mercaptoethanol 4%, sodium dodecyl sulfate 1%, glycerol 40%, and a trace amount of bromphenol blue) were mixed, and the samples were boiled for 3 min and kept on ice for 10 min. The samples were placed onto 12.5% (w/v) acrylamide gel using the Mini Protean gel apparatus (CLP, USA). The gels were electrophoresed using Tris base 25 mmol/L, glycine 192 mmol/L, and sodium dodecyl sulfate 0.1% (w/v) at 150 V. The proteins were subsequently transferred electrophoretically to an ECL nitrocellulose membrane (Amersham) using the CLP semi-dry Electroblotter(CLP, USA) at 150 mA constant current. Membranes were washed with TBST buffer (Tris base 10 mmol/L, NaCl 0.15 mol/L, and Tween-20 0.05%) for 10 min.
The blots were blocked by incubating them with 5% (wt/vol) powdered non-fat milk in TBST buffer, Nonidet P-40 2 mL, and sodium dodecyl sulfate 0.02% (wt/vol) (pH 8.0). Thereafter, the blots were incubated overnight with the primary anti-5-LO antibody (rabbit polyclonal; cayman chemical, Ann Arbor, MI, USA) at a dilution of 1:1000. The blots were then washed with TBST buffer, incubated with horseradish peroxidase-linked secondary antibody (anti-rabbit IgG; 1:3000) for 4 h at room temperature, and processed with the ECL kit. The blots were then washed with TBST and exposed to ECL film. The UVP Image Analyzer was used to measure and analyze the band intensity.
Statistical analysis Data were presented as mean±SD. Statistical difference was determined using one-way analysis of variance followed by the Student-Newman-Keuls method for multiple comparisons between groups. All statistical calculations were performed using a Sigmastat statistical package.
Results
Antigen-induced changes of IL-4 and IFN-γ, TNF-α, and NO in BALF and cerebral cortex homogenate Amounts of IL-4, TNF-α, and NO from BALF and cerebral cortex homogenates in antigen-challenged rats were markedly higher compared with samples from normal rats (P<0.05). In contrast, amounts of IFN-γ in antigen-challenged rats were less (BALF P<0.05, cerebral cortex homogenates P<0.01). Therefore, the IFN-γ/IL-4 ratio was lowered. DXM-treated rats had less IL-4, TNF-α, and NO amounts in cerebral cortex homogenates and higher IFN-γ amounts compared with that of model rats. The down-regulation of IFN-γ/IL-4 ratio in antigen-challenged rats was recovered by DXM (BALF P<0.05 and cerebral cortex homogenates P<0.01). Ketotifen had no effect on the amount of IL-4, IFN-γ, and NO in BALF and cerebral cortex homogenates, but inhibited the TNF-α both in BALF and cerebral cortex homogenates in model rats (P<0.05) (Table 1).
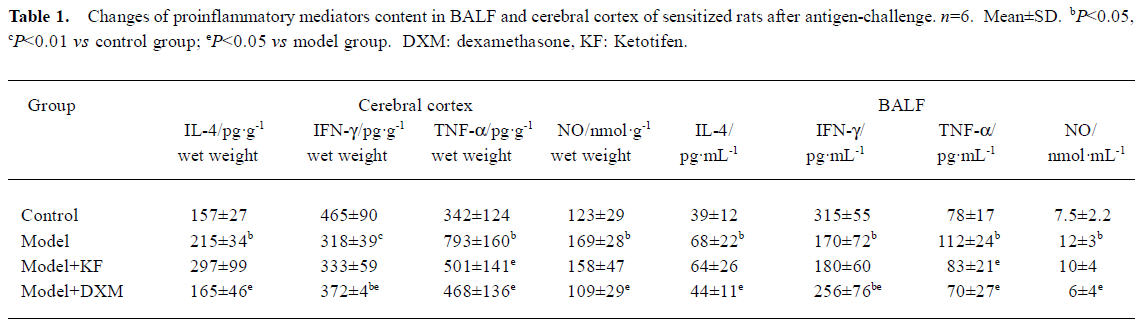
Full table
5-LO and LTA4-H mRNA expression in lung tissue and cerebral cortex Using GAPDH as the internal control, the band intensity of 5-LO and LTA4-H mRNA is shown in Figures 1, 2. The expression of 5-LO and LTA4-H mRNA in asthma rats were significantly higher than that in the control rats (P<0.05). Both DXM and ketotifen inhibited the 5-LO and LTA4-H mRNA expression of lung and cerebral cortex in the asthma rats (Figures 1, 2).
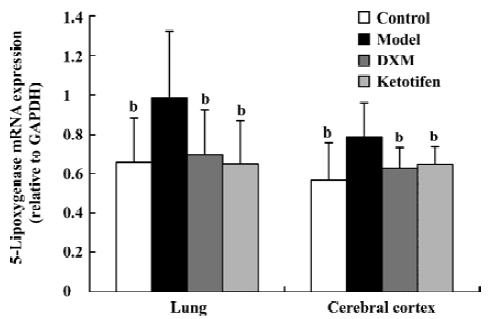
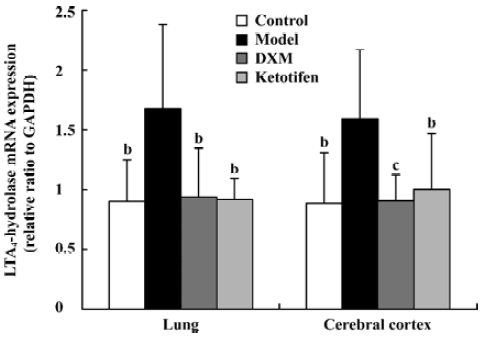
5-LO protein content Both 5-LO mRNA expression and the amount of cytosol 5-LO-immunoreactive protein in both cerebral cortex and lung tissue increased in the asthma rats compared with the control rats (P<0.05 and P<0.01 respectively; Figure 3). Both DXM and ketotifen significantly decreased the 5-LO protein level in cytosol of asthma rats (P<0.05).
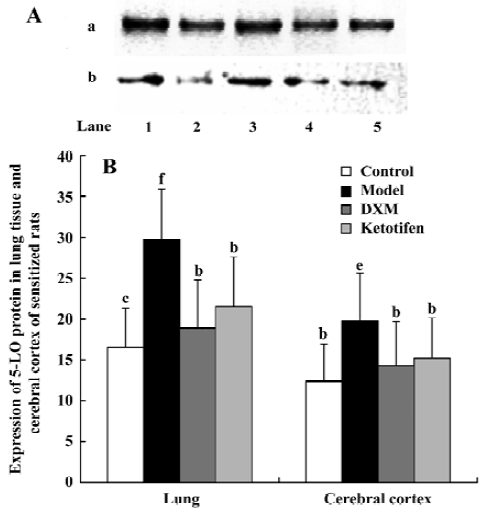
Discussion
The first step in the production of all LTs, the oxygenation of arachidonic acid (AA) to form 5-hydroperoxyeicosatetranoic acid and the immediate dehydration of this unstable intermediate to LTA4, is carried out by 5-LO. Metabolism of LTA4 by LTA4 hydrolase (LTA4-H) results in the production of the potent chemoattractant LTB4. Alternatively, LTA4 can be conjugated with glutathione by LTC4 synthase (LTC4-S) to produce LTC4 and its metabolites LTD4 and E4, collectively referred to as the cysteinyl LTs[6].
The actions of 5-LO metabolites and Th1/Th2 paradigm in the pathogenesis of asthma and allergic disease have been understood[7,8]. In the present research, IL-4 level, the expression of 5-LO and LTA4-H mRNA, and 5-LO protein are simultaneously increased in BALF and the cerebral cortex in asthma rats. We postulate that the increased expression of 5-LO pathway enzymes is partly due to the overproduction of proinflammatory cytokines, as heterogeneous profiles of eicosanoid generation would be determined by the absence or presence of additional local factors, particularly the Th2 cytokines that associate with mucosal surfaces in allergic diseases. Previous literature has shown that Th2 cytokines, thought to favor asthma and allergic diseases, upregulated LT synthesis. Recent findings showed that IL-4, IL-5, and IL-13 also upregulated cysteinyl LT1 receptor expression[9]. IL-4 priming dramatically induced the steady-state expression of LTC4-S[10], and IL-5 supported the localization of 5-LO to the nucleus of human mast cells in vivo[11]. With immunologic disorders, inflammatory mediators interacted with one another, creating upregulatory cascades by various feedback mechanisms[12]. Conversely, the regulation of cytokine expression by LTs has also been explored: cysteinyl LTs upregulated Th2 cytokine expression and decreased Th1 cytokine expression, favoring an allergic phenotype[13]. LTB4 stimulated monocytes and T cell to produce IL-1, TNF-α, and IL-5[14]. However, it is unclear how it influences pulmonary function during the increase of expression of 5-LO, LTA4-H mRNA, and LTB4 level in the cerebral cortex. Recently, our group found exogenous LTB4, by intercerebro-ventricularly injection, inhibited antigen-induced increase of lung resistance and decreased lung compliance in sensitized rats, and reduced antigen-induced eosinophils infiltration of airway in the sensitized mice (unpublished data). The results suggest the increase of 5-LO mRNA expression and metabolites in inflammatory status of asthma may be a negative feedback to relieve asthmatic inflammation.
Glucocorticoids are the most effective drugs to inhibit inflammatory reaction in asthma, but whether they are also able to inhibit the 5-LO pathway enzyme expression in sensitized rats after antigen challenge is still unclear. In our previous experiment, the effect of DXM in decreasing LTB4 and IL-4 content was found to decrease the number of inflammatory cells in cerebral cortex and lung tissue of sensitized rats[2,3]. In this study, administration of DXM (0.5 mg/kg, ip) before each challenge fully inhibited the 5-LO and LTA4-H mRNA expression. Conversely, Uz et al[15] found that DXM stimulated 5-LO mRNA expression in the brain of normal rats. These data suggest that glucocorticoid may be at least partly effective in regulating the metabolites of 5-LO pathways in the brain.
It is known that TNF-α is released in allergic responses from both mast cells and macrophages via IgE-dependent mechanisms, and elevated levels have been demonstrated in the BALF of asthmatic subjects undergoing allergen challenge[16]. In the present research, we found a coincidental increase of TNF-α between the cerebral cortex and lung tissue in asthma rats, which could be inhibited by DXM and ketotifen. Ketotifen, as the mast cell membrane stabilizer and H1 receptor antagonist, can inhibit the release of mediators from inflammatory cells. Our previous study demonstrated that ketotifen inhibited the increase of LTB4 level in the cerebral cortex and BALF in asthma rats[3]. In this study, ketotifen did not inhibit the downregulation of IFN-γ and upregulation of IL-4 but decreased 5-LO and LTA4-H mRNA expression and TNF-α level in asthma rats. Canetti et al[17] found that using a neutralizing anti-TNF-α antibody could block IL-18 induced LTB4 production, and Spanbroek et al[18] found granulocyte-macrophage colony-stimulating factor plus TNF-α promoted dendritic cells differentiation and induced a strong rise in 5-LO expression. The results suggest that ketotifen may not influence the Th1/Th2 paradigm but inhibits the release of mediators from mast cells. TNF-α may be also one of the proinflammatory mediators of regulating 5-LO and LTA4-H mRNA expression.
NO can inhibit the secretion of IL-2 and IFN-γ by Th1 cells but has no effect on IL-4 production by Th2 cells. Thus, NO seems to exert a self-regulatory effect on Th1 cells implicated in immunopathology. NO derived from airway epithelial cells, macrophages, and Th1 cells plays an important role in amplifying and perpetuating the Th2 cell-mediated inflammatory response, both in allergic and non-allergic asthma[19]. Low concentrations of reactive oxygen intermediates in combination with NO were additive in suppressing 5-LO enzyme activity, possibly through peroxynitrite generation. The ability of peroxynitrite to suppress LT synthesis is associated with its ability to cause nitrotyrosination and S-nitrosylation of the 5-LO enzyme[20]. Contrary to the inhibitory effect of DXM on NO content in this research, ketotifen did not inhibit the increased NO level in the asthma rats. Therefore, the regulatory effect of NO on 5-LO and LTA4-H requires elucidating.
In the present research, the correlative increase of 5-LO pathway and proinflammatory mediators between cerebral cortex and lung tissue were shown in sensitized rats after repeated antigen challenge. We postulate that an increase of 5-LO pathway expression and pro-inflammatory mediators of the brain may have regulatory effects on pulmonary inflammation of asthma.
References
- Frieri M. Neuroimmunology and inflammation: implications for therapy of allergic and autoimmune diseases. Ann Allergy Asthma Immunol 2003;90 Suppl 3:34-40.
- Xie QM, Chen JQ, Shen WH, Bian RL. Correlative changes of interferon-γ and interleukin-4 between cerebral cortex and pulmonary airway of sensitized rats. Acta Pharmacol Sin 2002;23:248-52.
- Deng YM, Xie QM, Chen JQ, Bian RL. Coincidental increase of leukotriene B4 between cerebral cortex and lung tissue of sensitized rats. Acta Pharmacol Sin 2003;24:1039-44.
- Shimada K, Navarro J, Goeger DE, Mustafa SB, Weigei PH, Weinman SA. Expression and regulation of leukotriene of leukotriene-synthesis enzyme in rat liver cells. Hepatology 1998;28:1275-81.
- Nakao A, Watanabe T, Ohishi N, Toda A, Asano K, Taniguchi S, et al. Ubiquitous localization of leukotriene A4 hydrolase in the rat nephron. Kidney Int 1999;55:100-8.
- Werz O. 5-Lipoxygenase: cellular biology and molecular pharmaco-logy. Curr Drug Targets Inflamm Allergy 2002;1:23-44.
- Hsieh FH, Lam BK, Penrose JF, Austen KF, Boyce JA. T helper cell type 2 cytokines coordinately regulate immunoglobulin E-dependent cysteinyl leukotriene production by human cord blood-derived mast cells: profound induction of leukotriene C(4) synthase expression by interleukin 4. J Exp Med 2003;193:123-33.
- Coffey M, Peters-Golden M. Extending the understanding of leukotrienes in asthma. Curr Opin Allergy Clin Immunol 2003;3:57-63.
- Thivierge M, Stankova J, Rola-Pleszczynski M. IL-13 and IL-4 up-regulate cysteinyl leukotriene 1 receptor expression in human monocytes and macrophages. J Immunol 2001;167:2855-60.
- Zaitsu M, Hamasaki Y, Matsuo M, Kukita A, Tsuji K, Miyazaki M, et al. New induction of leukotriene A4 hydrolase by interleukin-4 and interleukin-13 in human polymorphonuclear leukocytes. Blood 2000;96:601-9.
- Cowburn AS, Holgate ST, Sampson AP. IL-5 increases expression of 5-lipoxygenase-activating protein and translocates 5-lipoxygenase to the nucleus in human blood eosinophils. J Immunol 1999;163:456-65.
- Spanbroek R, Hildner M, Kohler A, Muller A, Zintl F, Kuhn H, et al. IL-4 determines eicosanoid formation in dendritic cells by down-regulation of 5-lipoxygenase and up-regulation of 15-lipoxygenase expression. Proc Natl Acad Sci USA 2001;98:5152-7.
- Nag S, Lamkhioued B, Renzi PM. Interleukin-2-induced increased airway responsiveness and lung Th2 cytokine expression occur after antigen challenge through the leukotriene pathway. Am J Respir Crit Care Med 2002;165:1540-5.
- Anthonsen MW, Andersen S, Solhaug A, Johansen B. Atypical lambda/iota PKC conveys 5-lipoxygenase/leukotriene B4-mediated cross-talk between phospholipase A2s regulating NF-kappa B activation in response to tumor necrosis factor-alpha and interleukin-1beta. J Biol Chem 2001;276:35344-51.
- Uz T, Dwivedi Y, Savani PD, Impagnatiello F, Pandey G, Manev H. Glucocorticoids stimulate inflammatory 5-lipoxygenase gene expression and protein translocation in the brain. J Neurochem 1999;73:693-9.
- Thomas PS. Tumour necrosis factor-α: the role of this multifunctional cytokine in asthma. Immunol Cell Biol 2001;79:132-40.
- Canetti CA, Leung BP, Culshaw S, McInnes IB, Cunha FQ, Liew FY. IL-18 enhances collagen-induced arthritis by recruiting neutrophils via TNF-alpha and leukotriene B4. J Immunol 2003;171:1009-15.
- Spanbroek R, Hildner M, Steinhilber D, Fusenig N, Yoneda K, Radmark O, et al. 5-Lipoxygenase expression in dendritic cells generated from CD34(+) hematopoietic progenitors and in lymphoid organs. Blood 2000;96:3857-65.
- Ricciardolo FL. Multiple roles of nitric oxide in the airways. Thorax 2003;58:175-82.
- Coffey MJ, Phare SM, Peters-Golden M. Interaction between nitric oxide, reactive oxygen intermediates, and peroxynitrite in the regulation of 5-lipoxygenase metabolism. Biochim Biophys Acta 2002;1584:81-90.